Chapter 3 NEUROANATOMY AND NEUROPHYSIOLOGY: INNERVATION OF THE LOWER URINARY TRACT
The storage and periodic elimination of urine depend on the coordinated activity of two functional units in the lower urinary tract: a reservoir, which is the urinary bladder, and an outlet consisting of the bladder neck, the urethra, and the urethral sphincter. Coordination between these organs is mediated by a complex neural control system located in the brain, spinal cord, and peripheral ganglia. Urine storage and release depend on central nervous system pathways. This distinguishes the lower urinary tract from many other visceral structures (e.g., the gastrointestinal tract, cardiovascular system) that maintain a certain level of function even after extrinsic neural input has been eliminated.
INNERVATION
The innervation of the lower urinary tract is derived from three sets of peripheral nerves: sacral parasympathetic (i.e., pelvic nerves), thoracolumbar sympathetic (i.e., hypogastric nerves and sympathetic chain), and sacral somatic nerves (i.e., primarily pudendal nerves) (Fig. 3-1).1–5
Sacral Parasympathetic Pathways
The sacral parasympathetic nerves, which in humans originate from the S2 to S4 segments of the spinal cord, provide most excitatory input to the bladder. Cholinergic preganglionic neurons located in the intermediolateral region of the sacral spinal cord send axons to ganglionic cells in the pelvic plexus and the bladder wall (see Fig. 3-1). Transmission in bladder ganglia is mediated by a nicotinic cholinergic mechanism. In some species (e.g., cats, rabbits) ganglionic synapses act like gating circuits, exhibiting marked facilitation during repetitive preganglionic activity and modulation by various transmitter systems, including muscarinic, adrenergic, purinergic, and enkephalinergic (Table 3-1).6,7 The ganglia can have an important role in regulating neural input to the bladder. Whether bladder ganglia in humans have similar properties is not known. Ganglion cells excite the bladder smooth muscle. Histochemical studies of the ganglia and nerves supplying the human lower urinary tract have shown that many ganglion cells contain choline acetyltransferase, acetylcholinesterase, and the vesicular acetylcholine transporter and therefore are cholinergic. Choline acetyltransferase–, acetylcholinesterase-, and acetylcholine transporter– positive nerves are abundant in all parts of the bladder but are less extensive in the urethra.1,2,5 Neuropeptide Y and nitric oxide synthase also have been identified in 40% to 95% of intramural ganglia of the human bladder. Several populations of axonal varicosities have been detected close to intramural ganglion cells, including substance P–, vasoactive intestinal polypeptide (VIP)–, and calcitonin gene–related peptide (CGRP)–positive axons, which are presumably collaterals of extrinsic sensory nerves; tyrosine hydroxylase and neuropeptide Y axons, which are likely to be sympathetic axons; and galanin- and neuropeptide Y–containing axons.
Parasympathetic neuroeffector transmission in the bladder is mediated by acetylcholine acting on postjunctional muscarinic receptors.8–11 M2 and M3 muscarinic receptors subtypes are expressed in bladder smooth muscle; however, examination of subtype-selective muscarinic receptor antagonists and studies of muscarinic receptor–knockout mice have revealed that the M3 subtype is the principal receptor involved in excitatory transmission.8–11 Activation of M3 receptors triggers intracellular Ca2+ release, whereas activation of M2 receptors inhibits adenylate cyclase.10 The latter may contribute to bladder contractions by suppressing adrenergic inhibitory mechanisms that are mediated by β3-adrenergic receptors and stimulation of adenylate cyclase.
In bladders of various animals, stimulation of parasympathetic nerves produces a noncholinergic contraction that is resistant to atropine and other muscarinic receptor–blocking agents. Adenosine triphosphate (ATP) (see Table 3-1) has been identified as the excitatory transmitter mediating the noncholinergic contractions.10–13 ATP excites the bladder smooth muscle by acting on P2X receptors, which are ligand-gated ion channels. Among the seven types of P2X receptors that have been identified in the bladder, P2X1 is the major subtype expressed in the rat and in human bladder smooth muscle.12,13 Purinergic agonists also modulate transmission in bladder ganglia.6,14,15 Although purinergic excitatory transmission is not important in the normal human bladder, it appears to be involved in bladders from patients with pathologic conditions such as detrusor overactivity, chronic urethral outlet obstruction, or interstitial cystitis.12,16,17
Parasympathetic pathways to the urethra induce relaxation during voiding.1,10,11,18–20 In various species, the relaxation is not affected by muscarinic antagonists and therefore is not mediated by acetylcholine. However, inhibitors of nitric oxide synthase block the relaxation in vivo during reflex voiding or block the relaxation of urethral smooth muscle strips induced in vitro by electrical stimulation of intramural nerves, indicating that nitric oxide is the inhibitory transmitter involved in relaxation.1,2,11,18,19 In some species, neurally evoked contractions of the urethra are reduced by muscarinic receptor antagonists or by desensitization of P2X purinergic receptors, indicating that acetylcholine or ATP are involved in excitatory transmission to urethral smooth muscle.21
Thoracolumbar Sympathetic Pathways
Sympathetic preganglionic pathways that arise from the T11 to L2 spinal segments pass to the sympathetic chain ganglia and then to prevertebral ganglia in the superior hypogastric and pelvic plexus and to short adrenergic neurons in the bladder and urethra. Sympathetic postganglionic nerves that release norepinephrine provide excitatory input to smooth muscle of the urethra and bladder base, inhibitory input to smooth muscle in the body of the bladder, and inhibitory and facilitatory input to vesical parasympathetic ganglia.1,7,11 Radioligand receptor binding studies showed that α-adrenergic receptors are concentrated in the bladder base and proximal urethra, whereas β-adrenergic receptors are most prominent in the bladder body.1,10 These observations are consistent with pharmacologic studies showing that sympathetic nerve stimulation or exogenous catecholamines produce β-adrenergic receptor–mediated inhibition of the body and α-adrenergic receptor–mediated contraction of the base, dome, and urethra. Molecular and contractility studies have shown that β3-adrenergic receptors elicit inhibition and α1-adrenergic receptors elicit contractions in the human bladder.10 The α1A-adrenergic receptor subtype is most prominent in the normal bladders, but the α1D-adrenergic receptor subtype is upregulated in bladders from patients with outlet obstruction, raising the possibility that α1-adrenergic receptor excitatory mechanisms in the bladder may contribute to irritative lower urinary tract symptoms in patients with benign prostatic hyperplasia.10
Sacral Somatic Pathways
Somatic efferent pathways to the external urethral sphincter are carried in the pudendal nerve from anterior horn cells in the third and fourth sacral segments. Branches of the pudendal nerve and other sacral somatic nerves also carry efferent impulses to muscles of the pelvic floor and proprioceptive afferent signals from these muscles, as well as sensory information from the urethra.2,22–24 Analysis of urethral closure mechanisms in the female rat during bladder distention or sneeze-induced stress conditions revealed that the major rise in urethral pressure occurred in the middle urethra and was mediated by efferent pathways in the pudendal nerve to the external urethral sphincter and by pathways in nerves to the iliococcygeus and pubococcygeus muscles, although not by pathways in the sympathetic or parasympathetic nerves.25
Neural Modulation
Postganglionic nerve terminals are sites of “cross-talk” between sympathetic and parasympathetic nerves and possible sites of gating mechanisms.26–30 For example, in the rat lower urinary tract, activation of M2/4 (muscarinic) cholinergic receptors on nerve terminals suppresses acetylcholine and norepinephrine release,30 whereas activation of M1 cholinergic receptors or α1-adrenergic receptors enhances acetylcholine release (see Table 3-1).26–29 Inhibitory M2/4 mechanisms are dominant at low frequencies of nerve activity and therefore may contribute to urine storage, whereas M1 facilitatory mechanisms are dominant at high frequencies of nerve stimulation and may contribute to an enhancement of neurally evoked bladder contractions during micturition to induce complete bladder emptying.
Other putative transmitters that have been identified in efferent pathways to the lower urinary tract of animals and humans may modulate neuroeffector transmission (see Table 3-1).3,31,32 Neuropeptide Y is present in adrenergic and cholinergic neurons,32 and when administered exogenously, it acts prejunctionally to suppress the release of norepinephrine and acetylcholine from postganglionic nerve terminals.21 Although nitric oxide relaxes smooth muscle in the urethra, it is inactive in bladder smooth muscle.10 However, in the bladder, it may act on afferent nerves to modulate excitability.10,31 VIP, which is released with acetylcholine, may function as an inhibitory transmitter in the bladder.10 Efferent control of the bladder and urethra is potentially complex and involves many transmitters and many synaptic gating mechanisms in ganglia and at postganglionic nerve terminals.
Lumbosacral Afferent Pathways
Afferent activity arising in the bladder is transmitted to the central nervous system by both sets of autonomic nerves.2,33–37 The afferent nerves most important for initiating micturition are those passing in the pelvic nerves to the sacral spinal cord. These afferents consist of small, myelinated (A) and unmyelinated (C) fibers that convey impulses from tension receptors and nociceptors, respectively, in the bladder wall.2,3,36 Electrophysiologic studies in the cat have shown that A bladder afferents respond in a graded manner to passive distention and to active contraction of the bladder.35–37 The intravesical pressure threshold for activation of these afferents ranges from 5 to 15 mm Hg, which is consistent with pressures at which humans report the first sensation of bladder filling during cystometry. The A afferents in the cat show a linear increase in firing with increasing intravesical pressures that extend into the noxious range, suggesting that nociceptive stimuli in the bladder may be encoded in part by high rates of firing in polymodal afferents that also transmit non-noxious information.
High-threshold C-fiber afferents have also been detected in the cat bladder.34 Under normal conditions, most of these afferents do not respond to bladder distention and therefore have been designated silent C fibers, but many can be activated by chemical irritation of the bladder mucosa3,34 or cold temperatures (Fig. 3-2).38 After chemical irritation, C-fiber afferents exhibit spontaneous firing with the bladder empty and increased firing during bladder distention.34 Activation of C-fiber afferents by chemical irritation facilitates the micturition reflex and decreases bladder capacity.2,3,39–41 Administration of capsaicin, a neurotoxin that desensitizes C-fiber afferents, blocks this facilitation but does not block micturition reflexes in normal animals, indicating that C-fiber afferent pathways are not essential for normal voiding.2,42,43
In the rat, A-fiber and C-fiber bladder afferents are not distinguishable on the basis of stimulus modality; both types of afferents consist of mechanosensitive and chemosensitive populations.44–47 C-fiber afferents that respond only to bladder filling have also been identified in the rat bladder and appear to be volume receptors possibly sensitive to stretch of the mucosa.2,44 C-fiber afferents are sensitive to the neurotoxins capsaicin and resiniferatoxin and to other substances, such as tachykinins, nitric oxide, ATP, prostaglandins, and neurotrophic factors, released in the bladder by afferent nerves, urothelial cells, and inflammatory cells (see Fig. 3-2).2,3,41,45,48–54 These substances can sensitize the afferent nerves and change their response to mechanical stimuli.
Mechanosensitive afferents from the bladder and urethra have been identified in sympathetic nerves passing to the lumbar spinal cord.55 These afferent pathways consist of myelinated and unmyelinated axons that respond to similar stimulus modalities as afferents in the pelvic nerve. The function of sympathetic nerve afferents in the control of micturition is uncertain; however, based on clinical observations, it is clear that in humans they carry nociceptive information from the lower urinary tract.
Afferent pathways from the urethra, which induce the sensations of temperature, pain, and passage of urine, travel in the pudendal nerve to the lumbosacral spinal cord.2,3 Afferents from the female sex organs, including the clitoris, vagina, and part of the innervation of the uterine cervix, also travel in the pudendal nerve.56,57 These afferents and pudendal nerve afferents from the striated sphincter muscles have a modulatory influence on micturition.3,24
The properties of lumbosacral dorsal root ganglion cells innervating the bladder, urethra, and external urethral sphincter in the rat have been studied with patch-clamp recording techniques in combination with axonal tracing methods to identify the different populations of neurons.58–65 Based on responsiveness to capsaicin, it is estimated that approximately 70% of bladder afferent neurons in the rat are the C-fiber type. These neurons exhibit high-threshold, tetrodotoxin-resistant sodium channels and action potentials and phasic firing (one to two spikes) in response to prolonged depolarizing current pulses.58,60,61 Approximately 90% of the bladder C-fiber afferent neurons also are excited by ATP, which induces depolarization and firing of afferent neurons by activating P2X3 or P2X2/3 receptors.65 These neurons express isolectin-B4 binding, which is commonly used as a marker for ATP-responsive sensory neurons.60 A-fiber afferent neurons are resistant to capsaicin and ATP and exhibit low-threshold, tetrodotoxin-sensitive sodium channels and action potentials and tonic firing (multiple spikes) to depolarizing current pulses. C-fiber bladder afferent neurons also express a slowly decaying A-type K+ channel that controls spike threshold and firing frequency.58,62 Suppression of this K+ channel induces hyperexcitability of bladder afferent nerves.58 These properties of dorsal root ganglion cells are consistent with the different properties of A-fiber and C-fiber afferent receptors in the bladder.
Immunohistochemical studies have shown that a large percentage of bladder afferent neurons contain peptides: CGRP, VIP, substance P, pituitary adenylate cyclase-activating polypeptide (PACAP), corticotropin-releasing factor (CRF), and enkephalins.66,67 In the bladder, these peptides are common in nerves in the submucosal and subepithelial layers and around blood vessels. The neurotoxin capsaicin, when applied locally to the bladder in experimental animals, releases peptides from peripheral afferent terminals and produces inflammatory responses, including plasma extravasation and vasodilatation.41 Afferent peptides can also modulate transmission in bladder ganglia.6 These findings suggest that the neuropeptides may be important neurotransmitters in afferent pathways in the lower urinary tract.
Urothelial-Afferent Interactions
Studies have revealed that the urothelium, which has been traditionally viewed as a passive barrier at the bladder luminal surface,2,68,69 has specialized sensory and signaling properties that allows urothelial cells to respond to their chemical and physical environment and to engage in reciprocal chemical communication with neighboring nerves in the bladder wall (see Fig. 3-2).68–80 These properties include expression of nicotinic, muscarinic, tachykinin, adrenergic, purinergic, and capsaicin (TRPV1) receptors70–76,80–83; responsiveness to transmitters released from sensory nerves; close physical association with afferent nerves74,84,85; and an ability to release chemical mediators such as ATP, acetylcholine, and nitric oxide that can regulate the activity of adjacent nerves and thereby trigger local vascular changes or reflex bladder contractions.71,75,77,82,86 The role of ATP in urothelial-afferent communication has attracted considerable attention because bladder or urethral distention releases ATP from the urothelium,72,77,87,88 and intravesical administration of ATP induces bladder hyperactivity,86,88 an effect blocked by administration of P2X purinergic receptor antagonists that suppress the excitatory action of ATP on bladder afferent neurons.65,86,88 Mice in which the P2X3 receptor was knocked out exhibited hypoactive bladder activity and inefficient voiding,76,88 suggesting that activation of P2X3 receptors on bladder afferent nerves by ATP released from the urothelium was essential for normal bladder function. It has also been reported that urothelial cells obtained from patients or cats with a chronic, painful bladder condition (i.e., interstitial cystitis) released significantly larger amounts of ATP in response to mechanical stretching than urothelial cells from normal patients.72,89 The release of other substances, including nitric oxide90 and a nonapeptide glycoprotein called APF,91–93 is also increased in feline interstitial cystitis and human interstitial cystitis, respectively. This raises the possibility that enhanced signaling between the urothelium and afferent nerves is involved in the triggering of painful bladder sensations (see Fig. 3-2).
Communication between the urothelium and afferent nerves may also involve another cell type, the myofibroblast.94–96 These cells, which stain intensely for vimentin and gap junction protein connexin 43, have been identified immediately below the urothelium in human and guinea pig bladders. Electron microscopic studies have revealed that the cells make close appositions with unmyelinated suburothelial nerve endings.95 Exogenous ATP can induce Ca+2-activated chloride currents in myofibroblasts by stimulation of P2Y receptors.96 It has been suggested that stretch-induced ATP released from urothelial cells may act on myofibroblasts, which modulate the excitability of adjacent afferent nerves.
ANATOMY OF CENTRAL NERVOUS PATHWAYS
Efferent Neurons
Preganglionic neurons in the lumbosacral parasympathetic nucleus have been identified in the intermediolateral region of the spinal cord in several species.3,97 In the cat, the sacral parasympathetic nucleus is divided into two groups of cells: a dorsal band and a lateral band. Neurons innervating the urinary bladder are located in the lateral band. These neurons have an extensive network of axon collaterals98 that is involved in a recurrent inhibitory mechanism99 that regulates reflex bladder activity. The neurons send dendrites to discrete regions of the spinal cord, including the lateral and dorsal lateral funiculus, lamina I on the lateral edge of the dorsal horn, the dorsal gray commissure, and the gray matter and lateral funiculus ventral to the autonomic nucleus (Fig. 3-3).97 It has been suggested that the dendrites in the lateral funiculus receive inputs from descending pathways from the brain, whereas the other dendrites receive segmental inputs from interneurons or primary afferents. Lumbar sympathetic preganglionic neurons and motor neurons in Onuf’s nucleus that innervate the external urethral sphincter have similar dendritic distributions (see Fig. 3-3).100–102
Afferent Projections
Afferent pathways from the lower urinary tract project to discrete regions of the dorsal horn that contain the soma or dendrites of efferent neurons innervating the lower urinary tract. Pelvic nerve afferent pathways from the urinary bladder of the cat103,104 and the rat105 project into Lissauer’s tract at the apex of the dorsal horn and then pass rostrocaudally, giving off collaterals that extend laterally and medially through the superficial layer of the dorsal horn (lamina I) into the deeper layers (laminae V to VII and X) at the base of the dorsal horn (Fig. 3-4A and see Fig. 3-3). The lateral pathway, which is the most prominent projection, terminates in the region of the sacral parasympathetic nucleus and also sends some axons to the dorsal commissure (see Figs. 3-3 and 3-4A).
Pudendal nerve afferent pathways from the external urethral sphincter of the cat have central terminations that overlap in part with those of bladder afferents in lateral laminae, I, V to VII, and X.100,101 Afferents from the female sexual organs also terminate in these areas.56,57 The overlap of the central projections of bladder afferents and the afferents in the pudendal nerve to the sex organs and urethra is of interest, because activation of the latter afferents can markedly influence bladder function.106–109
Spinal Interneurons
The spinal neurons involved in processing afferent input from the lower urinary tract and the sex organs have been identified by physiologic and anatomic tracing techniques.110–115 Interneurons that fire in response to bladder distention or mechanical stimulation of the vagina or uterine cervix have been detected in lateral intermediate gray matter near the sacral parasympathetic nucleus and in the region of the dorsal commissure (lamina X).2,108 Commonly, stimulation of sex organ afferents inhibits neurons activated by bladder distention, which is consistent with the inhibitory effect of these afferents on bladder reflexes.108,109
Spinal interneurons have also been identified by the expression of the immediate-early gene FOS (see Fig. 3-4B)110–112 and by transneuronal transport of pseudorabies virus from the bladder (see Fig. 3-4C).40,113–115 The protein product of the FOS gene can be detected immunocytochemically in the nuclei of neurons within 30 to 60 minutes after synaptic activation. In the rat, noxious or non-noxious stimulation of the bladder and urethra and electrical stimulation of the pelvic or pudendal nerves increased the levels of FOS protein, primarily in the dorsal commissure and in the area of the sacral parasympathetic nucleus (see Fig. 3-4B), the major sites of termination of afferents from the lower urinary tract. Neurons in these same regions are labeled by the pseudorabies virus (see Fig. 3-4C), which passes transsynaptically in a sequential manner from postganglionic to pregang-lionic efferent neurons and then to segmental interneurons and eventually to neurons in the brain (Fig. 3-5) (discussed later).113–115 Interneurons in the dorsal commissure region also project to the sphincter motor nucleus.116,117 These results indicate that interneurons in restricted regions of the intermediate gray matter and dorsal commissure play a major role in coordinating the different aspects of lower urinary tract function.
Patch-clamp recordings from parasympathetic preganglionic neurons in the rat spinal slice preparations have revealed that interneurons located immediately dorsal and medial to the parasympathetic nucleus make direct monosynaptic connections with the preganglionic neurons (PGNs).118–120 Microstimulation of interneurons in both locations elicits glutamatergic, N-methyl-D-aspartic acid (NMDA), and non-NMDA excitatory postsynaptic currents in PGNs. Stimulation of a subpopulation of medial interneurons elicits GABAergic and glycinergic inhibitory postsynaptic currents.118 In this way, local interneurons likely play an important role in excitatory and inhibitory reflex pathways controlling the preganglionic outflow to the lower urinary tract. Glutamatergic excitatory inputs have also been elicited by stimulation of the projections from lamina X and the lateral funiculus.121,122
Pathways in the Brain
The neurons in the brain that control the lower urinary tract have been studied with a variety of anatomic tracing techniques in several species. In the rat, transneuronal virus tracing methods113–115 have identified many populations of central neurons that are involved in the control of the bladder, urethra, and urethral sphincter, including the Barrington nucleus, the pontine micturition center (PMC); the medullary raphe nucleus, which contains serotonergic neurons; the locus ceruleus, which contains noradrenergic neurons; the periaqueductal gray (PAG); and the A5 noradrenergic cell group (see Fig. 3-5). Several regions in the hypothalamus and the cerebral cortex also exhibited virus-infected cells. Neurons in the cortex were located primarily in the medial frontal cortex.
Other anatomic studies in which anterograde tracer substances were injected into brain areas and then identified in terminals in the spinal cord are consistent with the virus-tracing data. Tracer injected into the paraventricular nucleus of the hypothalamus labeled terminals in the sacral parasympathetic nucleus and the sphincter motor nucleus (see Fig. 3-3).123–125 Neurons in the anterior hypothalamus project to the PMC, and neurons in the PMC project primarily to the sacral parasympathetic nucleus and the lateral edge of the dorsal horn and the dorsal commissure, areas containing dendritic projections from the preganglionic neurons, sphincter motoneurons, and afferent inputs from the bladder. Conversely, projections from neurons in the lateral pons terminate rather selectively in the sphincter motor nucleus (i.e., Onuf’s nucleus) (see Fig. 3-3).125 The sites of termination of descending projections from the PMC are optimally located to regulate reflex mechanisms at the spinal level.
REFLEX MECHANISMS CONTROLLING THE LOWER URINARY TRACT
The central pathways controlling lower urinary tract function are organized as simple on-off switching circuits that maintain a reciprocal relationship between the urinary bladder and the urethral outlet. The principal reflex components of these switching circuits are listed in Table 3-2 and are illustrated in Figure 3-6. Intravesical pressure measurements during bladder filling in humans and animals reveal that bladder pressure is maintained at a low and relatively constant level when bladder volume is below the threshold for inducing voiding (Fig. 3-7A). Accommodation of the bladder to increasing volumes of urine is primarily a passive phenomenon that depends on the intrinsic properties of the vesical smooth muscle and the quiescence of the parasympathetic efferent pathway.2–4 In some species, urine storage is also facilitated by sympathetic reflexes that mediate inhibition of bladder activity and closure of the urethral outlet (see Table 3-2 and Fig. 3-6).126,127 During bladder filling, the activity on the sphincter electromyogram also increases (see Fig. 3-7), reflecting an increase in efferent firing in the pudendal nerve and an increase in outlet resistance that contributes to the maintenance of urinary continence.24
Table 3-2 Reflexes to the Lower Urinary Tract
Afferent Pathway | Efferent Pathway | Central Pathway |
---|---|---|
Urine storage: low-level vesical afferent activity (pelvic nerve) | External sphincter contraction (somatic nerves) | Spinal reflexes |
Internal sphincter contraction (sympathetic nerves) | ||
Detrusor inhibition (sympathetic nerves) | ||
Ganglionic inhibition (sympathetic nerves) | ||
Sacral parasympathetic outflow, inactive | ||
Micturition: high-level vesical afferent activity (pelvic nerve) | Inhibition of external sphincter activity | Spinobulbospinal reflexes |
Inhibition of sympathetic outflow | ||
Activation of parasympathetic outflow to the bladder |
The storage phase of the urinary bladder can be switched to the voiding phase involuntarily (by reflex) or voluntarily (see Fig. 3-7B). The former is readily demonstrated in the human infant (see Fig. 3-7A) and in the anesthetized animal when the volume of urine exceeds the micturition threshold. At this point, increased afferent firing from tension receptors in the bladder reverses the pattern of efferent outflow, producing firing in the sacral parasympathetic pathways and inhibition of sympathetic and somatic pathways. The expulsion phase consists of an initial relaxation of the urethral sphincter (see Fig. 3-7A) followed in a few seconds by a contraction of the bladder, an increase in bladder pressure, and flow of urine. Secondary reflexes elicited by the flow of urine through the urethra facilitate bladder emptying.4
These reflexes require the integrated action of neuronal populations at various levels of the neuraxis (Fig. 3-8 and see Fig. 3-6). Certain reflexes, such as those mediating the excitatory input to the sphincters and the sympathetic inhibitory input to the bladder, are organized at the spinal level (see Fig. 3-6A), whereas the parasympathetic input to the detrusor has a more complicated central organization involving spinal and spinobulbospinal pathways (see Fig. 3-6B).
Storage Reflexes
Sympathetic Pathways
Studies in animals indicate that sympathetic input to the lower urinary tract is tonically active during bladder filling.3 Surgical or pharmacologic blockade of the sympathetic pathways can reduce urethral resistance, bladder capacity, and bladder wall compliance and increase the frequency and amplitude of bladder contractions. Sympathetic firing is initiated at least in part by a sacrolumbar intersegmental spinal reflex pathway triggered by vesical afferent activity in the pelvic nerves (see Table 3-2 and Fig. 3-6A).126,127 This vesicosympathetic reflex represents a negative-feedback mechanism whereby an increase in bladder pressure triggers an increase in inhibitory input to the bladder, allowing the bladder to accommodate larger volumes of urine. The reflex pathway is inhibited during micturition, and the inhibition is abolished by transection of the spinal cord at the thoracic level, indicating that it originates at a supraspinal site, possibly the PMC (see Fig. 3-6B).127
Somatic Efferent Pathways to the Urethral Sphincter
Reflex control of the striated urethral sphincter is similar to control of sympathetic pathways to the lower urinary tract (see Fig. 3-6A). During bladder filling, pudendal motor neurons are activated by vesical afferent input, whereas during micturition, motor neurons are reciprocally inhibited. Inhibition depends in part on supraspinal mechanisms, because in animals with chronic spinal lesions and in paraplegic patients, it is weak or absent. In paraplegics, the uninhibited spinal vesicosphincter excitatory reflex pathway commonly initiates a striated sphincter contraction in concert with a contraction of the bladder (i.e., bladder-sphincter dyssynergia) (see Fig. 3-7C). This reflex interferes with bladder emptying.
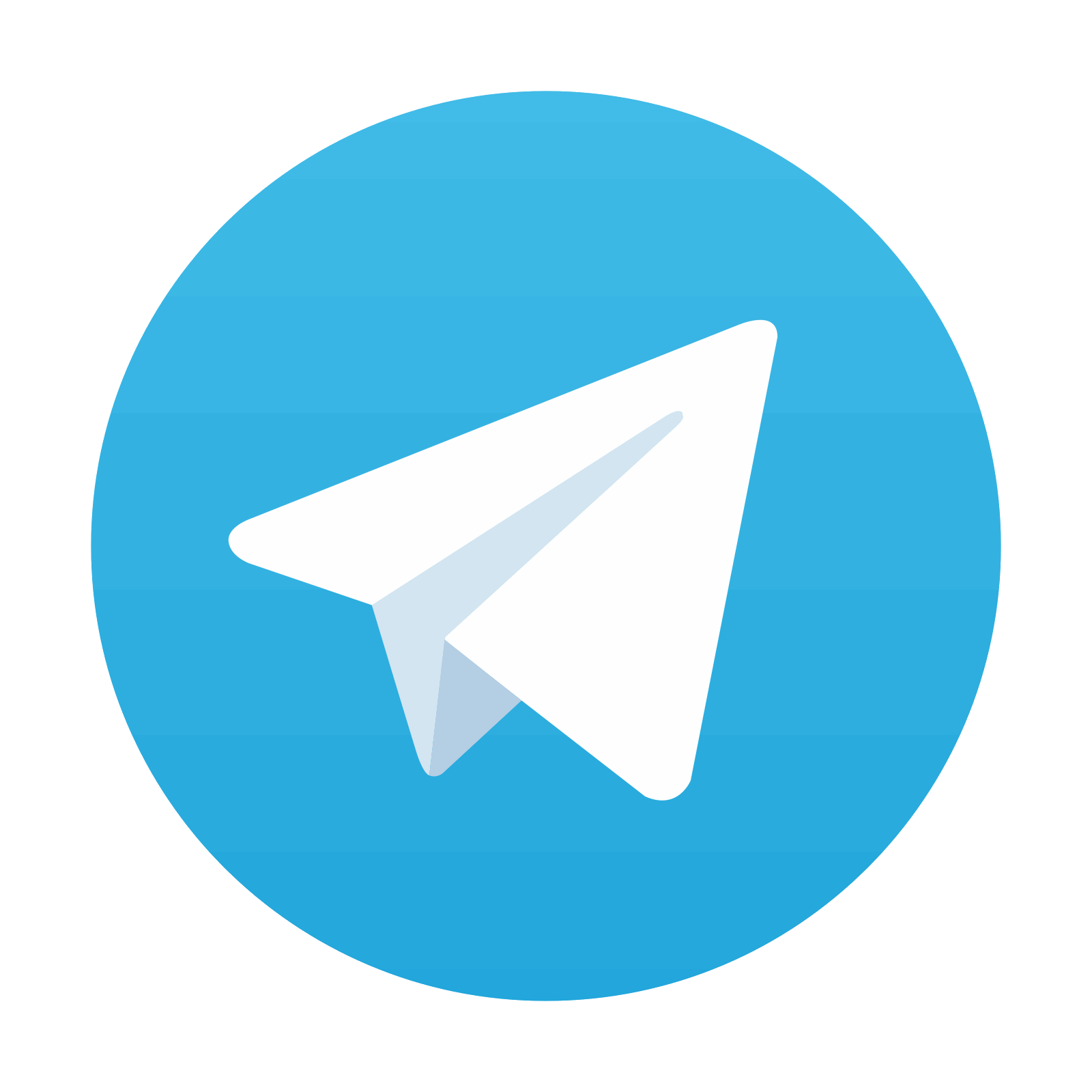
Stay updated, free articles. Join our Telegram channel

Full access? Get Clinical Tree
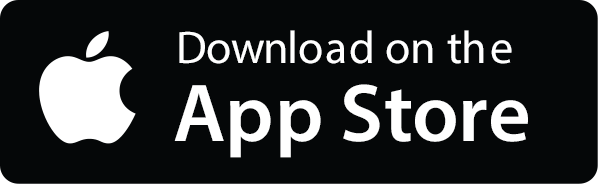
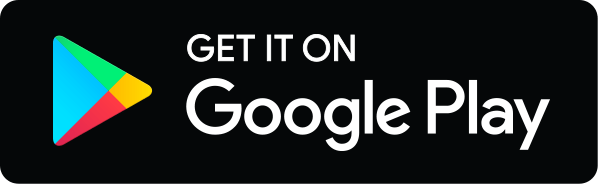