Chapter 37 USE OF CADAVERIC FASCIA FOR PUBOVAGINAL SLINGS
The pubovaginal sling (PVS) is a popular and successful treatment for female stress urinary incontinence whether due to urethral hypermobility or intrinsic sphincter deficiency. There are many variations for this procedure, and techniques have changed since it was first introduced in 19071 and then later resurrected by McGuire and Lytton.2 Over the last two decades, there have been many modifications in technique, and even the supposed underlying principle of function has changed. Historically, the mechanism by which the PVS was thought to work was to provide support and prevent urethral descent during increases in intra-abdominal pressure and to provide a backstop against which urethral compression would occur. The “new era” slings, positioned at the mid-urethra, are suggested to function by providing a point of support about which distortional “obstruction” occurs during stress.
GRAFT SELECTION
Cadaveric Fascia
The successful use of cadaveric fascia has been described in the orthopedic and ophthalmologic literature for many years,3,4 and the use of allograft fascia for PVS surgery was first reported in 1994.5 Currently in the United States, more than 90,000 soft tissue allografts are used annually.6 These tissues are harvested, processed, preserved, and distributed by certified tissue banks that comply with the policies of the American Association of Tissue Banks.
The benefits of allograft fascia over autologous fascia include shorter operative time and hospital stay, lower morbidity, and less postoperative pain.7–10 Potentially, the cadaveric fascia might also be superior to the patient’s own fascia, because the latter may be collagen deficient, which may be a cause for the original support deficiency. It has been reported that the theoretical mechanism of action of the allograft fascia is to serve as a scaffold for the in-growth of host tissues, ultimately leading to replacement of the allograft collagen with host collagen.11 However, in many cases in our experience with exploration years after the original surgery, these grafts usually have maintained their integrity and show little evidence of “incorporation” by the host.
PROCESSING OF FASCIA
The preparation of cadaveric fascia is a multistep process. It begins with the medical and social screening of a potential donor, using information provided by the potential donor’s family and physician. Next comes testing of the potential donor’s blood with tests approved by the U.S. Food and Drug Administration (FDA) to rule out AIDS, hepatitis B and C, human immunodeficiency virus (HIV) types 1 and 2, and syphilis.12
After completion of the screening step, the fascia is harvested with aseptic technique and processed to remove all cells and DNA, thus further decreasing the risk of disease transmission while maintaining the native collagen/protein framework. Ideally, the fascia should be harvested from within 2 cm of the iliotibial band, because only this location has the integrity for graft strength and durability. Processing and decellularization techniques vary, but all maintain a common theme that requires incubation in 70% isopropyl alcohol or other bactericidal and antiviral solutions to remove any viruses.
The tissue is then dehydrated via deep-freezing (lyophilization) or with organic solvents. The fascia may then undergo gamma irradiation. Radiation dosage is not standardized, and it is suggested that doses greater than 25,000 Gy (2.5 Mrads) may increase tissue weakening.13
Despite these preparation techniques, intact genetic material has still been found in final allografts.14,15 Whether this isolated DNA has the ability to replicate or to transmit infectious material remains to be seen. Explanations for failure of disease transmission include an unclear mode of pathogen transmission, an unidentified pathogen, and a window of time before a donor seroconverts with positive antibody (e.g., HIV testing).14 Of course, the donors may simply have been free of transmissible disease.
Fitzgerald and colleagues showed that allografts retain donor antigens after processing but that ultimately host antigens replace donor antigens after implantation.16 Because donors and recipients are not cross-matched, and graft rejection does not appear to be a problem, the identification of donor antigens in a graft is of questionable clinical significance.
Effect of Preparation on Fascia Strength
Tissue banks differ in the method used for graft preparation, and researchers have attempted to determine which process is associated with improved outcomes. However, outcomes are influenced by many other patient variables that are rarely included when these analyses are considered. Not surprisingly, therefore, the results have been conflicting. Sutaria and Staskin compared freeze-dried, freeze-dried/gamma-irradiated, and solventdehydrated/gamma-irradiated fascias and found no statistical differences in maximum load and thickness-adjusted maximum load.17 However, Lemer and coworkers found that freeze-dried allograft had inferior mechanical properties compared with autologous fascia or solvent-dehydrated fascia.18 Hinton and associates compared solvent-dehydrated/gamma-irradiated fascia with freeze-dried/nonirradiated fascia and found a higher stiffness, higher maximum load to failure, and higher maximum load per unit width of graft with the former.19
The cause of the potential differences in various fascia preparations is unclear and also is of questionable significance. A possible explanation for the difference between freeze-dried and solvent-dried fascia is that ice crystal formation during the freeze-drying process may weaken the collagen structure in the fascia.18 Of note, the forces generated in studies measuring fascial strength are usually much greater than the physiologic forces created at the bladder neck and proximal urethra. This raises the issue that ex vivo strength is not the only variable necessary for success with an allograft fascia PVS. Lin and colleagues attempted to confirmed this suspicion, showing that, with the patient in a horizontal and mild tilt position, the tension placed on the sling was far less than that necessary to break the fascia.20 Finally, but most importantly, the variability of fascial thickness is not addressed in any of these studies.
Risk of Disease Transmission
The prevention of disease transmission focuses on the areas of screening and sterilization. Since 1985, when the American Association of Tissue Banks began requiring the screening of prospective donors and testing of tissue for viruses, there have been no documented cases of HIV transmission.21 Other extensive reviews have not identified a single case of disease originating from transplanted allograft fascia.22
However, there remains concern about the transmission of prions, the causative agent of Creutzfeldt-Jakob disease (CJD) and other forms of spongioencephalopathies. It is estimated that, with current methods of processing, the theoretical risk of a patient’s developing CJD from an allograft is 1 in 3,500,000. This is significantly lower than the 1 in 10,000 to 1 in 100,000, overall risk in the general population of “naturally” acquiring the disease.23
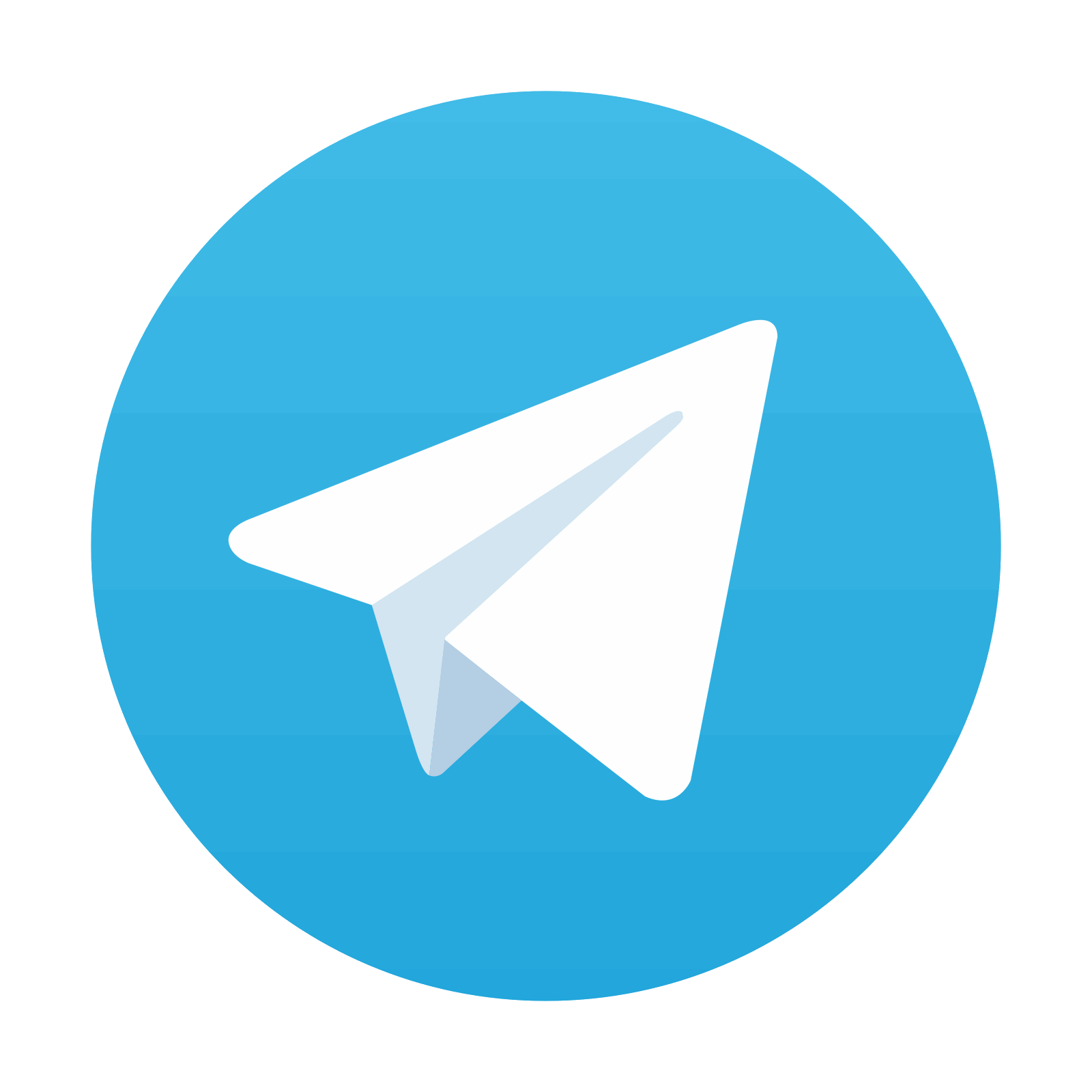
Stay updated, free articles. Join our Telegram channel

Full access? Get Clinical Tree
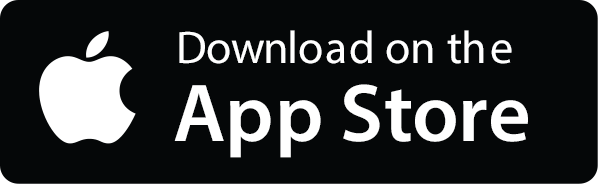
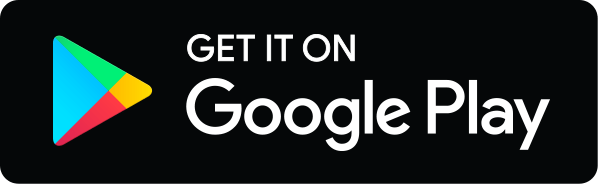