BRIEF HISTORY OF VASCULAR ACCESS
The history of vascular access for hemodialysis is closely tied to the history of dialysis. In 1924, Georg Haas in Giessen, Germany, performed the first hemodialysis treatments in humans. It lasted for 15 minutes, and he used glass needles to access the radial artery and return blood into the cubital vein (1). In a total of 11 treatments in uremic patients until 1929, Haas used fractionated dialysis to clear approximately 400 mL of blood at a time. The artificial kidney was made up of three glass cylinders with U-shaped collodion tubes. Willem Kolff, a young physician from Kampen, The Netherlands, with the support of H. Berk in 1943 developed a “rotating drum kidney” with larger surface area of the filter made of a cellophane membrane. The first patient he dialyzed was a 29-year-old housemaid with CKD. She underwent 12 dialysis treatments, but the therapy had to be stopped as each cannula placement required a cut down to the artery and she ran out of access sites (2). In 1946, Kolff came to the United States and after a time in New York came to Boston, where he worked with John P. Merril and Carl Walter to construct the “Brigham Artificial Kidney.”
The challenge of repetitive vascular access prevented dialysis from becoming a routine method for the treatment of chronic kidney insufficiency. This changed dramatically in the 1960s. Building on the idea of Nils Alwall (Lund, Sweden) to connect artery and vein with rubber tubing and a glass cannula, Quinton et al. (3) developed in Seattle an AV Teflon shunt. Their first patient, Clyde Shields, a Boeing machinist, survived for over 10 years after the insertion of his first Teflon AV shunt in March 1960. The tapered ends of two thin-walled Teflon cannulas were inserted into the radial artery and the adjacent cephalic vein in the distal forearm. While not on dialysis, the external ends were connected by a curved Teflon bypass tube, which was later replaced by a more flexible silicon rubber tubing. As with the availability of cellophane for the earliest artificial kidneys and heparin to maintain anticoagulation, the invention of Teflon and silicon allowed for new medical devices.
In 1966, Brescia et al. (4) (all New York) published their landmark account of 14 side-to-side anastomoses between the radial artery and the cephalic vein at the wrist, the native AVF was born. One year later, Sperling et al. (5) (Würzburg, Germany) presented 15 patients with end-to-end anastomoses, and in 1968, Lars Röhl et al. (6) (Heidelberg, Germany) presented results from 30 patients with radial-artery side-to-vein-end anastomoses. The different anastomosis techniques each fit specific anatomic needs and physiologic considerations. Another example is the Gracz fistula (7) presented in 1977, and modified by Klaus Konner (8), a proximal forearm fistula, that relies on the perforating vein from the superficial to the deep forearm venous system to limit blood flow in the fistula and prevent steal in patients with peripheral artery disease due to age, hypertension, or diabetes.
The Teflon shunt is an early example of an implanted medical device, partly internal, partly external. In 1961, Stanley Shaldon (London, United Kingdom) unable to find a surgeon to place the cannulae necessary for dialysis, inserted catheters into the femoral artery and vein with the Seldinger technique (9,10). Since then, catheters as a dialysis device have evolved to be placed with a cutaneous tunnel in which a Dacron cuff at the catheter’s neck ensures secure placement and a relative seal against microorganisms.
Material sciences have continued to evolve the development of grafts for use in hemodialysis. In 1969, George Thomas (11) (Seattle) attached Dacron patches to the common femoral artery and vein, which were then connected with a silastic tube and brought to the surface of the anterior thigh. The Thomas shunt was soon replaced by the arrival of the expanded polytetrafluoroethylene (PTFE) graft, as LD Baker (12) (Phoenix) in 1976 presented first results in 72 hemodialysis patients. The PTFE graft has remained the graft material of choice, although grafts made of biologic material have been available since 1972 (13). More recent strategies are aiming at drug-eluting grafts to enhance the functional life of the access (14) and tissue-engineered blood vessels from autogenous fibroblasts and endothelial cells (15).
In parallel with the advances in surgical techniques and material science, the diagnostic armamentarium of physicians concerned with vascular access care has expanded dramatically. Most of this technology centers on minimally invasive imaging and endovascular procedures. Seldinger’s idea of an endovascular guidewire over which diagnostic and therapeutic devices can be positioned is the basis for much of this development (9). In 1972 and 1973, Thelen et al. (16) and Staple (17) independently from each other described the angiographic retrograde imaging of AVFs in hemodialysis patients. David Ergun in 1979 first described a computerized fluoroscopy technique that revolutionized noninvasive cardiovascular imaging (18) and is now widely used in the diagnostic and therapeutic evaluation of dialysis accesses. Dotter et al. (19) and Grüntzig et al. (20) advanced the use of catheter-guided balloon angioplasty, that in 1982 found its first documented use in hemodialysis AVFs and AVGs by Gordon et al. (21).
Providers of hemodialysis care into the 1990s were predominantly access surgeons with vascular, transplant, or general subspecialization and interventional radiologists. This left many of the growing number of dialysis patients in the United States without dedicated access care, and other types of procedures and patients often trumped their interventions. It was into this void that the nephrologists such as Steve Schwab and Gerald Beathard stepped by acquired expertise in dialysis access procedures, documenting equal or superior outcomes compared to established care (22–28), in turn creating interventional nephrology as a distinct clinical field.
VASCULAR ACCESS FOR ACUTE DIALYSIS
Nontunneled dual-lumen central venous catheters are the most available form of vascular access for acute dialytic therapy. Central venous catheters can be inserted at the bedside using the Seldinger technique with ultrasound guidance and may support extracorporeal blood-flow rates greater than 300 mL/min. Separation of the arterial and venous ports minimizes recirculation of blood. Central venous catheters may be used for conventional intermittent hemodialysis as well as continuous renal replacement therapies.
The National Kidney Foundation Dialysis Access Guidelines recommend nontunneled hemodialysis catheters for hemodialysis duration of less than 1 week in acute kidney injury because of the increased infection risk compared with tunneled hemodialysis catheters. Unlike time-limited and relative predictable episodes for intoxications requiring dialysis, recovery from acute kidney injury with need for dialysis is very difficult to predict. In fact, only a minority of patients recover renal function in less than 1 week (29). For this reason, we primarily place tunneled catheters in patients with acute kidney injury, unless the patient is too unstable for transport to the procedure suite or has other contraindications to insertion of a tunneled catheter.
Catheter Materials
Currently on the market available are polyurethane and silicone catheters. Polyurethane catheters are relatively rigid at room temperature allowing bedside insertion, and their softness at body temperature minimizes the risk of vein perforation during prolonged catheterization (30).
Although silicone is the softest and least thrombogenic material, its softness necessitates the use of a peel-away sheath or internal stylets for percutaneous insertion (31). Insertion of silicone catheters by internal or external jugular venotomy is an alternative approach.
Acute Catheter Insertion Sites
Dual-lumen hemodialysis catheters may be inserted into the femoral or internal jugular vein. Because subclavian vein catheter placement may lead to subclavian vein stenosis, subclavian vein catheters should not be placed in patients who will ultimately require fistula creation for chronic hemodialysis.
Femoral Vein
Cannulation of the femoral vein should be performed under ultrasound guidance; instructional videos for insertion technique have been published online (32). Femoral vein cannulation is an invaluable technique for patients with pulmonary edema because it may be performed with the patient in the semirecumbent position. The femoral vein is cannulated immediately below the inguinal ligament as with increasing distance from the inguinal ligament the femoral artery is found more frequently overlying the femoral vein such that attempts to puncture the femoral vein distally leads to higher complications (33). As in obese patients, the inguinal fold is often more distal than the inguinal ligament; its location has to be carefully determined by feeling for the bony landmarks, medially the pubic tubercle, laterally the anterior superior iliac spine. Usually the abdomen has to be moved cranially and taped in place for safe access to the vein. External rotation of the leg increases total and accessible femoral vein diameter and decreases surfaces depth (34). In patients with bleeding diathesis, the initial puncture can be performed using the 21G needle of a micropuncture kit and then increase the guidewire from 0.018 inches to the usually used 0.035 inches wire in the dialysis catheter kit.
The incidence of life-threatening complications is lower for femoral vein cannulation than for internal jugular vein cannulation. Complications of femoral vein cannulation include ileofemoral vein thrombosis, AVF, retroperitoneal hemorrhage from vein perforation, and hemorrhage from accidental puncture of the femoral artery. Using ultrasound guidance increases success of the procedure (risk of failure reduced by 90%) as well as shortens procedure time, while the risk of arterial puncture and hematoma formation are fourfold reduced (35). Patients with femoral vein catheters must remain at bed rest in the hospital. In intensive care unit (ICU) patients with body mass index <28.5, femoral vein catheter colonization rates are comparable or lower than internal jugular vein catheters, while slightly higher in more obese patients, but overall comparable (FIGURE 3.1) (36). This observation has been confirmed for nontunneled catheters used in the ICU in general (37) as well as in subsequent studies of dialysis catheters (38). Catheter infections appear to be a random effect and not related to a threshold time beyond which a catheter has been in place as colonization of removed catheter in the same large randomized prospective study (36) was in fact higher for catheters removed within 5 days than those removed after 5 days.
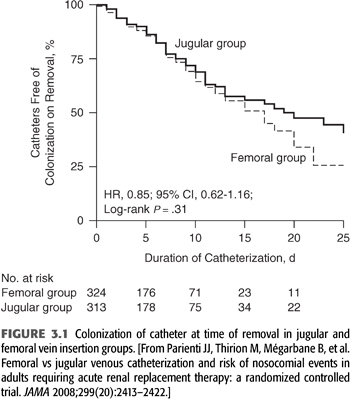
The length of the femoral dialysis catheter is important and should be 24 cm or longer to place the catheter tip in the inferior vena cava as otherwise recirculation increases significantly (39).
Subclavian Vein
Subclavian vein catheterization may lead to subclavian vein stenosis, precluding fistula placement in the ipsilateral arm. As mentioned previously, subclavian vein catheters should not be placed in patients for whom chronic hemodialysis is anticipated. Recent guidelines discourage this technique regardless of anticipated duration of use.
Internal Jugular Vein
Ultrasound-guided insertion of internal jugular vein catheters is associated with high success and very low complications rates (35). Traditionally, due to relative stiffness of nontunneled hemodialysis catheters, the recommended target tip position for internal jugular vein catheters has been the superior vena cava to avoid atrial perforations. Typically 13- to 16-cm-long catheters are used for right-sided insertions, and 16 to 20 cm catheters for left-sided insertions. A recent randomized trial of longer, soft silicone nontunneled catheters targeting the right atrium for tip position documented improved dialyzer life span as well as increased dialysis dose for continuous renal replacement therapy in the ICU (40) without increasing atrial arrhythmias. Risk of local hematoma formation as well as vein thrombosis is higher for internal jugular than femoral vein catheters.
Duration of Temporary Catheter Use
Temporary catheters achieve lower blood-flow rates than other types of permanent vascular access. The 2006 update of the National Kidney Foundation/Kidney Disease Outcomes Quality Initiative (NKF/KDOQI) Clinical Practice Guidelines for Vascular Access (41) recommend limiting use of nontunneled internal jugular vein catheters to 1 week because of the high incidence of infection. Other risks include inadvertent removal, hemorrhage, air embolism, and patient discomfort, all of which are increased with temporary catheters (42). Plans should be made to convert all temporary catheters to a permanent access if use is expected to exceed 1 week (43). For most instances of acute kidney injury requiring renal replacement therapy, insertion of a tunneled hemodialysis catheter is preferred as the primary access modality (29). In some clinical settings where tunneled dialysis catheters are not available or too costly, nontunneled dialysis catheters have been used in conjunction with antibacterial ointments at the exit site for over 30 days with fewer than two infections per 1,000 catheter days (44), and nontunneled catheters may even be used to bridge the time until fistula maturation using antibiotic lock solutions (45). Given the stiffer nature of these temporary catheters, increased central vein stenoses seem likely, but data are lacking, and the use of nontunneled catheters beyond 1 to 2 weeks is strongly discouraged where tunneled catheters are available.
Complications of Central Vein Cannulation
Insertion Complications
Landmark-guided insertion complications become less likely as the operator gains experience (46). The incidence of serious insertion complications is higher for subclavian vein and internal jugular vein cannulation than for femoral vein cannulation (47). TABLE 3.1 lists the most common insertion complications, which have markedly decreased with the transition from landmark techniques to ultrasound guidance.
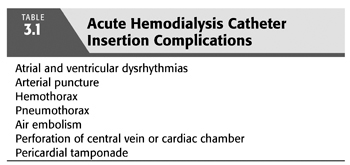
Atrial arrhythmias may result from endocardial irritation by the guidewire or catheter but are generally of limited clinical significance (48). Ventricular arrhythmias occur in approximately 20% of patients but rarely require therapy (49).
Inadvertent arterial puncture is a common insertion complication. Manual pressure will prevent significant hematoma from femoral artery and carotid artery puncture, even in patients with bleeding diatheses (50). Although serious hemorrhage complicates less than 1% of subclavian vein catheterization attempts (42,51), we do not recommend attempts at subclavian vein catheterization in patients with coagulopathies or severe thrombocytopenia because of the difficulty of applying direct manual pressure to the subclavian artery.
To minimize the chance of fatal pneumothorax, two rules must be followed:
1. The operator should never attempt cannulation of the subclavian vein ipsilateral to the only healthy lung.
2. Even an unsuccessful attempt at subclavian or internal jugular cannulation may still lead to pneumothorax. A chest radiograph should be obtained before cannulation of the contralateral subclavian is attempted.
Perforations of the superior vena cava or cardiac chamber may lead to hemothorax, mediastinal hemorrhage, pericardial tamponade, and death (51). These complications are rare but are more likely following subclavian vein cannulation than following internal jugular vein cannulation, perhaps because of the curved path that a subclavian catheter must follow. To minimize the chance of perforation during insertion, the operator must never advance the catheter without protection by a J-tipped guidewire. A chest radiograph must be obtained following insertion to confirm that the catheter is within a central vein and exclude pneumothorax. If the catheter tip is in the right atrium, the catheter should be pulled back to prevent right atrial perforation. Prolonged catheterization may lead to erosion and perforation of the superior vena cava; pericardial tamponade should be considered in the hypotensive hemodialysis patient with a central venous dialysis catheter.
Air embolism rarely complicates central vein cannulation (51), but care should be taken to avoid accidental introduction of air through the introducer needle, dilator, or catheter.
Injuries to the brachial plexus (52), trachea (53), and recurrent laryngeal nerve (54) are rare complications of internal jugular vein cannulation.
Use of ultrasonographic guidance during catheter insertion decreases complications and should be used (55,56). Ultrasonographic confirmation of target vein patency before cannulation prevents futile attempts at cannulation of thrombosed veins. In addition, the introducer needle may be inserted under direct ultrasonographic visualization. Fewer needle passes are required for successful cannulation when the ultrasonographic device is used (35). Recent guidelines strongly encourage this technique.
Infection
The mechanism of infection is migration of bacteria from the patient’s skin through the catheter insertion site and down the outer surface of the catheter or even more common contamination of the catheter lumen during hemodialysis (57). Rarely, infection is caused by infusion of infected solutions. The use of chlorhexidine-impregnated dressing at the exit site prevents catheter colonization as well as catheter-related bloodstream infections (58). Nontunneled catheter infection rates are higher for femoral than internal jugular and then subclavian vein catheters (59): 1.5, 3.6, and 4.6 infections per 1,000 catheter days in one recent large randomized controlled study (47) with the mean length of catheter use of 5 days.
Bacterial isolates associated with catheter colonization and catheter-related bacteremia (CRB) reflect the location of the catheter. While typical skin flora, such as Staphylococcus epidermidis, is most prevalent for any catheter site, gram-negative isolates are more common for femoral vein catheters (36).
Evidence of exit-site infection, even in the absence of systemic symptoms, should prompt catheter removal. Exit-site infection presages bacteremia, presumably because the absence of a cuff permits migration of bacteria along the tunnel and outer surface of the catheter.
Other preventive measures are listed in TABLE 3.2. Disinfection with 2% aqueous chlorhexidine at the time of insertion is preferred to disinfection with povidone-iodine or isopropyl alcohol (60,61). Careful attention to use of maximal sterile barrier precautions during catheter insertion or guidewire exchange is recommended (TABLE 3.3) (62). Hemodialysis catheters should not be used for infusions or blood sampling, but temporary catheter designs with a third access port for blood sampling and infusions are available. After catheter placement and at the end of each hemodialysis treatment, the skin should be disinfected using chlorhexidine or povidone-iodine solution (63). An antimicrobial ointment, mupirocin or Polysporin, applied to the catheter exit site reduces infections (44,64,65), and antibiotic lock solutions such as a mixture of cefazolin (10 mg/mL), gentamicin (5 mg/mL), and heparin (10,000 U/mL) can reduce catheter associated infections (45) with catheter infection rate very similar to tunneled catheters over an initial 30- to 60-day period. Lastly, a dry gauze dressing or a semipermeable transparent dressing should be applied at each hemodialysis treatment rather than an occlusive dressing (66,67).
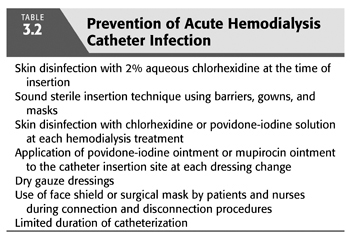
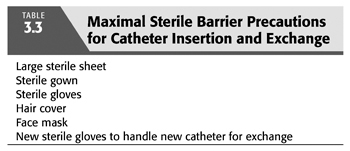
Adherence to the Centers for Disease Control and Prevention guidelines (68) for hand hygiene is low in both acute and chronic hemodialysis units (69). Aseptic technique during access handling, including use of a surgical mask for staff and patients, and adherence to hand hygiene guidelines decrease the risk for catheter-related infection (62).
A febrile hemodialysis patient with a central venous catheter should be presumed to have CRB unless there is strong evidence to the contrary. Rigors are especially suggestive of bacteremia. Blood cultures should be obtained, the catheter removed, and antibiotics administered. A new catheter may be placed once the patient defervesces. Antibiotic therapy should be continued for 2 to 3 weeks. In the event of metastatic infection (e.g., vertebral osteomyelitis or endocarditis), a longer course of antibiotic therapy will be required.
Catheter Thrombosis
Intracatheter thrombus impedes extracorporeal blood flow. Treatment consists of instilling reconstituted alteplase [recombinant human tissue plasminogen activator (tPA)] (or similar agent) in the affected catheter lumens for 30 to 120 minutes (70). This procedure may be repeated if necessary or tPA left in the catheter overnight. The slow leaking of tPA from the catheter may assist in lysing thrombus that may have formed around the catheter.
If catheter occlusion persists, catheter exchange may be performed using a J-tipped guidewire. We use a method where after careful cleaning of the skin and catheter, the sterile drape is applied such that the catheter ports are outside the sterile field. The catheter is then carefully retracted 2 to 4 cm, clamped just at the skin level, and cut 1 cm from the clamp. The guidewire is passed into the catheter while manually compressing the catheter. This approach uses a catheter segment that was inside the patient and reduces contamination from passing through and handling of the catheter ports which are difficult to sterilize effectively.
Extracatheter or mural thrombosis is a less frequent but more serious complication (71). Clinically symptomatic vessel thrombosis is most frequent with femoral vein catheters (47); however, the overall incidence is between 0.5% and 1.4%. Treatment of symptomatic central venous thrombosis should include systemic anticoagulation. If the catheter is still needed, it remains in its position but should be removed if not further necessary. In critically ill patients in the ICU, there is often a multitude of catheters, and possible cardiac device leads crowding the central veins, and removal of a catheter at one site and insertion into another may just lead to a second site of thrombosis.
Central Vein Stenosis
Subclavian vein stenosis may occur after placement of subclavian dialysis catheters (72–74), while central vein stenosis occurs less commonly after placement of internal jugular vein catheters (75–77). Arm edema is the presenting complaint of most central vein stenoses. As blood-flow rates in each of the subclavian veins is in the range of 100 to 250 mL/min, and 250 to 500 mL/min in the brachiocephalic veins, it is often only after the creation of an ipsilateral AV access with additional flow rates of 500 to 2,500 mL/min that clinical symptoms develop. This can be an immediate or a delayed occurrence, and in some cases, central vein stenoses develop and become apparent without prior cannulation of the central veins. Formation of venous collaterals can alleviate symptoms over time. While the exact pathogenesis of symptomatic central vein stenoses has not yet been determined conclusively, any form of vein injury and increased blood-flow rates seem to contribute and exacerbate each other.
VASCULAR ACCESS FOR CHRONIC HEMODIALYSIS
According to the most recent United States Renal Data System (USRDS) data collection, at the end of 2013, there were 466,607 prevalent dialysis patients in the United States, a 63.2% increase since 2000. The incidence of new dialysis patient in 2013 was 113,944, 24% larger than in 2000 (78); 90.8% of incident ESKD patient received hemodialysis, necessitating an autogenous AV access (AVF), a non-autogenous (prosthetic or biograft) AV access (AVG), or TC to provide access to high-volume blood flow. Successful hemodialysis procedures require adequate performance of any of these access options.
The ESKD population remains at less than 1% of the Medicare population but accounts for about 5.6% of Medicare spending in 2013. Costs for Medicare patient with an AVF are lower than for patients with a tunneled catheter or graft, and per person per year access event costs are also highest for graft, and higher for catheters than AVFs (USRDS 2010) (79). Creating and maintaining functional access for the large and growing number of dialysis patients poses economic challenges. One may speculate that these costs will rise out of proportion as diabetes and hypertension both affect the peripheral vasculature with potential detriment to long-term access options and increased vascular access complications. Vascular access remains the Achilles heel of chronic hemodialysis. Vascular access that is beset with complications is costly to both the patient and society; vascular access failure has been the most frequent cause of hospitalization for patients with ESKD/CKD; however, the availability of outpatient access centers has markedly reduced the need for admission. The primary AVF is the form of vascular access most likely to provide long-term complication-free vascular access. The importance of careful preparation for primary AVF placement months before the need for hemodialysis cannot be overemphasized.
Types of Permanent Vascular Access
There are three general types of long-term vascular access: tunneled hemodialysis catheters, autogenous AV accesses (AVF), and non-autogenous (prosthetic or biograft) AV accesses (AVG). The nomenclature for dialysis accesses follows the Society of Vascular Surgery (SVS) definitions. A tunneled catheter is named after the vessel it enters; for example, internal jugular vein tunneled hemodialysis catheter. For AVF site of use (forearm, upper arm, thigh, etc.), the originating artery and the connecting vein are used as well as an indication that this is an autogenous, transposed, or translocated access; for example, upper arm brachial-cephalic autogenous access for an AVF that connects the upper arm cephalic vein with the brachial artery (80).
Tunneled Cuffed Catheters
In 2013, more than 80% of patients initiating dialysis in the United States were using a tunneled hemodialysis catheter (78). The proportion of TC users decreased to 68.3% at 90 days and 20% at 1 year. Overall mortality of patients using a tunneled catheter is higher than for those using an AVF or AVG for dialysis (81,82).
Subcutaneous ports connected to catheters were used for several years in the United States as part of the LifeSite Hemodialysis Access System (83–86). However, this device was removed from the market after several cases of skin breakdown and infection around the device access site.
Catheters for acute dialysis are made of the relatively rigid polyethylene, while TCs are made of silicone, polyurethane, or Carbothane (polyurethane/polycarbonate copolymers). Silicone catheters are very soft, require a larger wall thickness to prevent collapse, and are greatly weakened by iodine, and for these reasons are rarely used. Polyurethane has high material strength, which allows for thinner catheter walls and therefore thinner catheters with equal flow characteristics, and is also less thrombogenic than silicone (30). However, polyurethane is weakened by alcohol, which today is the preferred catheter cleansing solution. Carbothane combines the exceptional material advantages of polyurethane with even greater strength and tolerance of alcohol, iodine, and peroxide cleaning solutions (87).
For two decades, subclavian placement of dialysis catheters was the preferred route (88); however, this has now been abandoned, as venograms revealed the frequent presence of severe stenosis or venous occlusion at the site of subclavian cannulation. Internal and external jugular vein insertion is preferred over femoral, inferior vena cava, and transhepatic catheters. Subclavian vein insertion is only a last resort. More recently, inside-out placement of tunneled catheters, similar to cardiac lead placement, from the right atrial appendix through the anterior mediastinum (89) or the junction of brachiocephalic-subclavian vein (90) with percutaneous exit in the subclavian region has been demonstrated for patients with total occlusion of the chest central veins.
TC insertion can be achieved either be conversion of an existing nontunneled catheter (43) or by de novo insertion. Right-sided TC insertion is preferred as the catheter has a more direct passage into the right atrium with fewer wall contacts and better function (91). With each heartbeat, the catheter moves, and a left-sided TC will have two to three wall contact points, eliciting endothelial injury and potentially initiating a process of progressive central vein stenosis and occlusion. Tunneled catheters should be inserted using ultrasound and fluoroscopic guidance. Methods have been published for insertion of tunneled catheters without fluoroscopy (92), but success rates are lower, and the risk for major injury to large vascular structures as well as the pericardium through dilators and valved sheath is higher. We do not recommend insertion of tunneled catheters without real-time fluoroscopic guidance.
Technical details of the tunneled catheter insertion procedure are beyond the scope of this chapter. However, there are several procedural circumstances, knowledge of which aids the general nephrologist diagnosis and care of the patient with a TC.
The catheter should be sutured to skin at the wings of the catheter. Sutures that attach to the skin at the exit site irritate the skin and may facilitate exit site infections and protrusion of the catheter cuff. The cuff of the catheter should be positioned within 1 to 1.5 cm of the exit site to allow easy removal and exchange of the catheter while at the same time minimizing the length of the catheter segment that is colonized by skin flora between cuff and skin. Sutures that are tied circumferentially around the catheter body have the potential to constrict the catheter leading to dysfunction and facilitate cuff extrusion (FIGURE 3.2). This may present as an immediate catheter dysfunction on the day of insertion. The catheter cuff should be fully attached after 3 to 4 weeks, and the wing sutures can be removed if they irritate the patient’s skin.
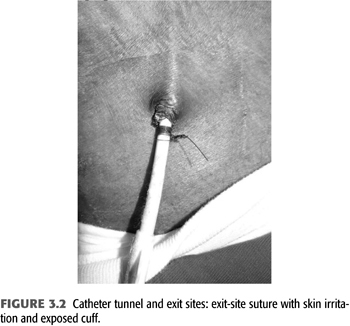
The catheter tip should be positioned in the right atrium when the patient is recumbent during catheter insertion for a particular reason: TCs are encased in a fibrinous sheath in their course through the skin (“tunneled segment”) as well as in the intravascular lumen. The only fix point for the catheter is the Dacron cuff which is fixed to the skin by invading skin cells and firmly connected to the fibrinous sheath of the tunneled segment. When sitting or standing, the tissue fixed to the cuff usually follows gravity to a more dependent location pulling up the catheter tip as much as the cuff moves downward. This effect is more pronounced in patients with voluminous breast tissue. The exit site should be in the lateral segment of clavicle and not more than 3 to 5 cm below its lower margin as elastic stretch of skin adds to catheter tip movement. Tunneled catheters support bidirectional 400 to 450 mL/min blood-flow rates (800 to 900 mL/min total volume moved). Recirculation is increased if these flow volumes are exchanged in the superior vena cava or the brachiocephalic veins rather than the right atrium. In addition, prolonged exposure of the relatively narrow brachiocephalic vein and superior vena cava to large blood-flow volumes leads to stenosis at the site of the catheter tips. This may be an effect of the mechanical injury by catheter tips, jet-like fluid propulsion or suction of the vessel wall, as well as presence of catheter-tethered thrombus. For all these reasons, catheter tip position in the right atrium is preferred.
Several catheter tip designs have been developed to reduce recirculation and allow high flow into the catheter tips: split tips, step tip, symmetric tip, and centrally angled tips. During episodes of catheter dysfunction (see the following texts for more in depth discussion), catheters are often used in reversed configuration, which increases recirculation in all but symmetric tip catheters. Colored dye video studies revealed that only symmetric tip catheters had negligible changes in recirculation when used in a reversed configuration (93). In patients with central vein stenosis or occlusion who are tunneled catheter–dependent, the available space for the catheter tip is shortened, and symmetric-tip catheters are more suitable to function in 2 to 3 cm shorter spaces.
Catheter diameter and length both influence venous and arterial pump pressure with a given blood flow; there is partial collapse of the arterial lumen and expansion of the venous lumen, such that the fraction of delivered flow in catheters decreases with increasing absolute flow rates (91,93). This effect is less pronounced in larger diameter catheters (#15.5 or #16 French vs. #14.5 French) and more pronounced in longer catheters, such as femoral vein or translumbar catheters.
Catheter Dysfunction
Catheter dysfunction can be categorized as early (within the first 4 weeks) and late (after 4 weeks) and, in addition, as static (the catheter has no or minimal aspiration but may be flushable) and dynamic (arterial or venous pressure alarms when blood-flow rates are increased beyond a threshold too low to deliver sufficient clearance, <200 mL/min).
Early dysfunction is usually related to tip position and other technical issues associated with the insertion of the catheter. Immediately after catheter insertion, circumferential sutures around the catheter and fixed at or around the exit site can—either in the context of procedural tissue edema or gravitational pull—constrict the catheter. This type of dysfunction can be treated by cutting the constricting suture and placing new sutures through the catheter wings. Posteroanterior and lateral chest x-ray images, if possible in recumbent and sitting position, are used to evaluate the catheter tip position and confirm that is in the right atrium and not outside vascular structures or in the azygous or hemiazygous vein. If the catheter tip position is not in a position consistent with the superior vena cava or right atrium, a chest computed tomography or fluoroscopic evaluation with contrast dye are necessary to determine location of the catheter and guide further treatment steps. Catheter tips that are retracted into the central veins usually present with early dynamic dysfunction, while catheter tips outside the vessel do not allow for fluid removal (early static).
Late static dysfunction is usually due to intracatheter formation of thrombus. Any fill solution in the catheter lumen very effectively leaks out of the catheter and blood mixes into the catheter (95) within hours after application due to the large diameter of the catheter and differences in viscosity between blood and typically used fill solutions. This type of endoluminal thrombus is very effectively treated by instilling 2 mg tPA into the catheter lumina (96).
Late dynamic dysfunction is usually seen in the context of a fibrinous sheath and catheter-associated thrombus (97). Guidewire-assisted exchange of the tunneled catheter with balloon disruption of the fibrinous sheath is very effective in treating this type of catheter dysfunction. An alternate strategy for sheath removal is stripping it with a gooseneck snare that is passed through a common femoral vein sheath into the right atrium. The snare encircles the catheter and strips away the fibrin sheath as it is retracted (FIGURE 3.3) (98).
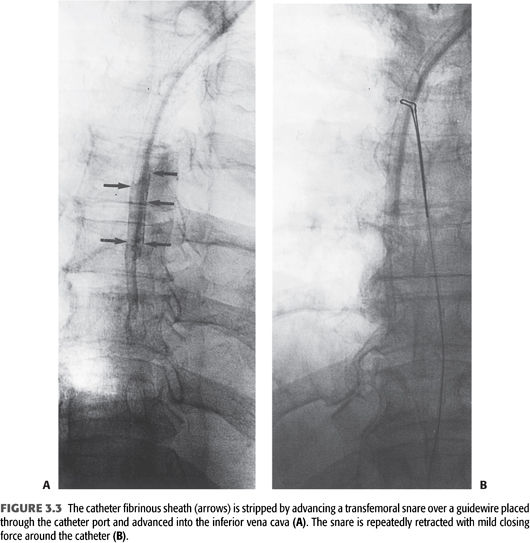
Cuffed Tunneled Catheter Infection
There are four compartments in a tunneled catheter, each of which may become infected: the exit site, the subcutaneous tunnel, the intravascular segment of the catheter with its associated fibrinous sheath, and intra-atrial organized thrombus attached to the catheter (FIGURE 3.4). Indwelling catheters are seen to be colonized within 48 hours when the catheter surface is examined with electron microscopy (99). Infections during the first 10 days after catheter insertions are usually considered to come from the outer surface of the catheter (contamination during insertion procedure or migration along the catheter from the skin), while infections 30 days or more after insertion are considered to originate from the inner surface biofilm. Usually there is colonization of the biofilm prior to bacteremia and clinical symptoms (100).
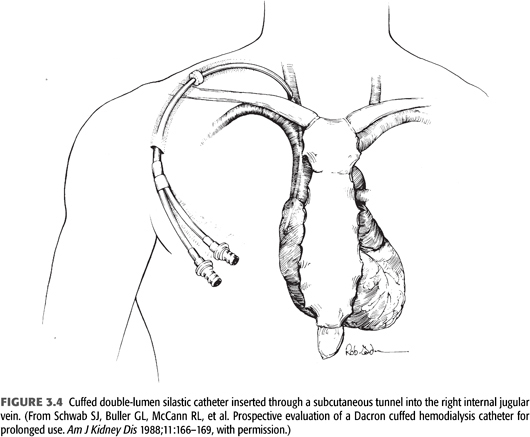
Exit Site
Exit-site infections (FIGURE 3.5) are more common than CRB episodes, by some accounts 50% more, and over 90% are successfully treated with systemic antibiotics as well as local exit site (91). Prophylaxis of exit-site colonization to prevent infections has been evaluated. Johnson et al. (65) demonstrated that application of 2% mupirocin ointment to tunneled catheter exit sites significantly decreased rates of staphylococcal exit-site infections and bacteremias. A potential problem associated with mupirocin prophylaxis is the appearance of resistant staphylococcal strains (101,102). Using Polysporin ointment for tunneled catheter exit-site prophylaxis, Lok et al. (64) demonstrated decreased catheter-related infections and decreased mortality. A preliminary study has shown that application of antibacterial honey (MEDIHONEY) to catheter exit sites is as effective as mupirocin in decreasing catheter-related infections (103). When choosing to apply ointments at the skin exit site, the carrier bases have to be compatible with the catheter material as otherwise focal deterioration may be caused (FIGURE 3.6). A dry gauze dressing or a semipermeable transparent dressing should be used to dress the catheter exit site (66,67). Occlusive dressings should be avoided; they trap any drainage and create a moist environment at the exit site. If the integrity of an exit site appears compromised without infection of the tunnel segment (see FIGURE 3.5), then the catheter can be exchanged with retunneling to a new exit site away from the infected area. This procedure requires a cut down on the catheter tunnel near the neck vein insertion site.
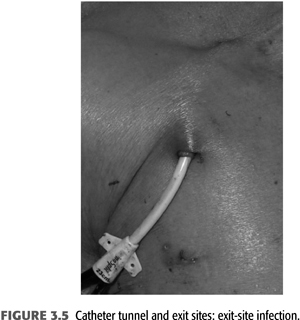
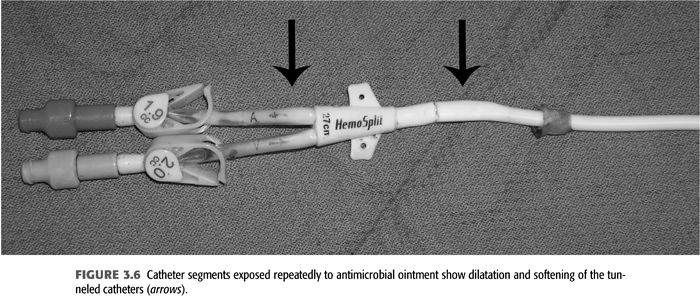
Catheter Tunnel
Infections of the tunneled catheter (FIGURE 3.7) require removal of the catheter and insertion of a new catheter, usually on the contralateral side as there often is some degree of cellulitis around the inflamed tunnel segment. As there is continuity of the skin tunnel into the endovascular fibrinous sheath, systemic antibiotics should be used for 2 to 3 weeks similar to a CRB. Blood culture and cultures of purulent material expressed during the catheter removal should be sent to narrow antibiotic treatment after initial coverage for gram-positive and gram-negative organisms. The choice of the initial combination depends on local patterns of drug resistance but usually includes vancomycin and a third- or fourth-generation cephalosporin.
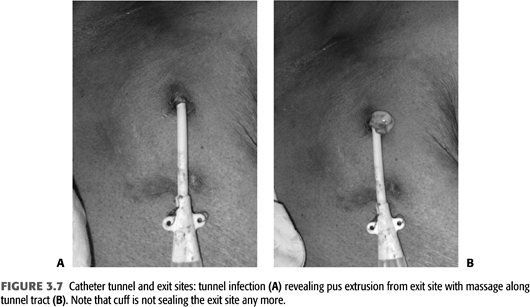
Endovascular Segment and Fibrinous Sheath
Infection of the endovascular catheter segment is associated with CRB which is common in patients using a dialysis catheter, and the presence of a catheter is a major risk factor for bacteremia among hemodialysis patients (104,105). The relative risk for infection-related death is increased twofold to threefold among catheter-dependent hemodialysis patients compared to those using fistulas or grafts (106,107). The frequency of CRB reported in several large series ranges from 0.8 to 5.5 cases per 1,000 patient days or 0.9 to 2.0 episodes per patient-year (108,109). This wide range likely reflects differences in practice patterns as rates of bacteremia as low as 1/1,000 days at risk have been achieved with detailed catheter protocols (110). Infection is a common reason for catheter removal and morbidity in dialysis patients (108,110). Bacteremia can result in life-threatening complications, including septic shock, endocarditis, septic arthritis, osteomyelitis, or epidural abscess.
Bacteremia should be suspected when a hemodialysis patient with a catheter develops fever and chills. Paired quantitative blood cultures from the peripheral blood and the catheter are the most accurate for diagnosing CRB (111). This is not routinely performed in outpatient units because of the difficulty in peripheral venous sticks in hemodialysis patients. In the absence of concurrent blood cultures from the catheter and a peripheral vein, a clinical diagnosis of CRB requires exclusion of alternate sources of infection (108). However, bacteremia will often lead to seeding of endovascular devices such that exchange of a tunneled catheter during successful treatment of bacteremia from a different source is reasonable, in particular for Staphylococcus aureus. Blood cultures should be obtained from the catheter 1 to 2 weeks after completion of antibiotics for non-CRB to ascertain that the catheter has not been colonized.
Catheter-Related Bacteremia Management
Empiric antibiotic treatment should be initiated immediately if CRB is suspected, without waiting for blood cultures to become positive (108). Gram-positive organisms, including a significant number of methicillin-resistant species, are isolated in the majority of CRBs. However, a substantial portion of bacteremia isolates are gram-negative organisms (108). Therefore, the optimal empiric antibiotic regimen for suspected CRB includes both vancomycin and an antibiotic with broad-spectrum gram-negative bacterial coverage, such as a third-generation cephalosporin. Antibiotic regimens should be adjusted once culture and sensitivity reports are available. Hospitalization is indicated if the patient is clinically septic or has evidence of metastatic complications such as endocarditis, osteomyelitis, epidural abscess, or septic arthritis. Aminoglycosides are excellent adjuncts for gram-positive infections treated with vancomycin as they are bacteriocidal rather than bacteriostatic. However, there are cumulative effects of aminoglycosides on hearing and balance such that lifetime exposure should be minimized (112). Continued bacteremia with hemodynamic instability despite sufficient treatment with appropriate antibiotics should prompt evaluation for catheter removal.
Treatment strategies for CRB that have been studied in hemodialysis catheter patients with regard to reemergence of the original organism after completion of antibiotics include (a) systemic antibiotics alone for 3 weeks, (b) systemic antibiotics plus a catheter lock solution, (c) systemic antibiotics plus catheter exchange (with or without disruption of a fibrinous sheath), and (d) catheter removal with delayed reinsertion (TABLEs 3.4 to 3.8).
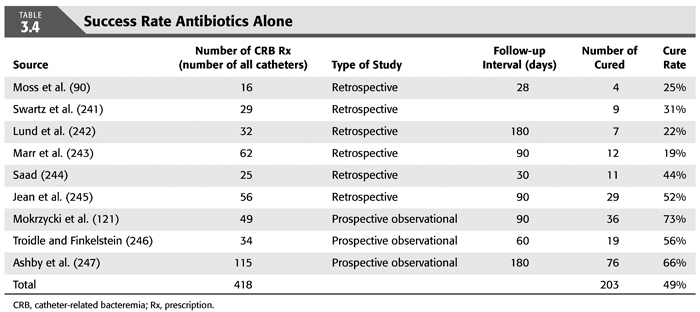
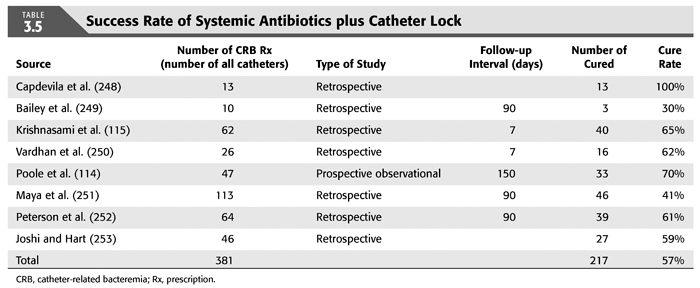
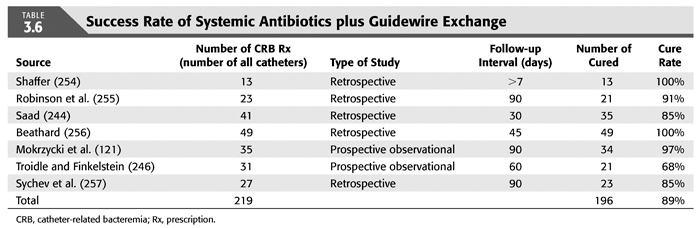
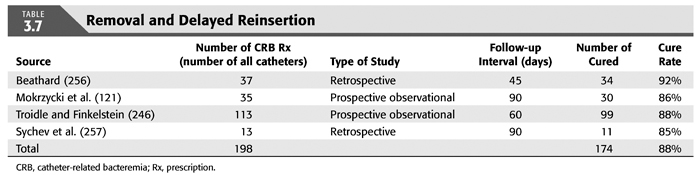
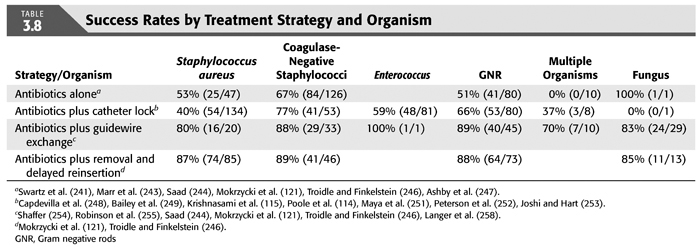
The overall success rate of antibiotics alone is only 50%, and we discourage this approach. Systemic antibiotics with catheter lock overall only succeed in just over 50% of catheter infections, but in some patients with coagulase-negative staphylococci infections, the success rate of approximately 77% may be sufficient if catheter exchange were to represent an undue burden. The utility of antibiotic lock therapy is believed to be treatment directed to the bacterial biofilms that form on the internal and external surfaces of catheters soon after insertion. These biofilms are thought to play a critical role in bacterial antimicrobial resistance and recalcitrant infections (113). Systemic antibiotics do not penetrate the biofilm well, and proteins in the biofilm provide adhesion sites for organisms to bind to, particularly S. aureus. Instillation of a concentrated antibiotic–anticoagulant solution into the catheter lumen (the antibiotic “lock”) at concentrations significantly higher than those achievable in the blood may permit successful eradication of the infection while salvaging the catheter (114,115). Systemic antibiotics with exchange over guidewire, and as we perform it, with disruption of the fibrinous sheath during the procedure are as successful as removal and delayed reinsertion. Even for fungal infection, this approach is successful in over 80% of cases. A minority of about 10% of patients in the United States who use tunneled catheters long term is catheter dependent. Moving the catheter to a new site is often not possible, and exchange over a guidewire, frequently with treatment of an associated central vein stenoses or occlusions, preserves the only access option these patients rely on. Instillation of gentamicin (20 to 40 mg) into the catheter tunnel can be used as an adjunct during exchange. Guidewire exchange is the first recommendation for CRB by the Infectious Disease Society of America (116), and nephrologists will have to work within their institutions to create awareness for the special balance that needs to be struck between loss of access sites and catheter removals.
Infection Prophylaxis for Cuffed Tunneled Catheters
Strategies to prevent CRB have been evaluated. Silver-coated tunneled dialysis catheters showed no difference in frequency of bacteremia compared to untreated catheters (117). Routine instillation in catheter lumens of antimicrobial solutions alone and in combination with anticoagulant or lytic solutions has been investigated. A gentamicin–citrate solution and a taurolidine solution have shown efficacy in decreasing incidence of bacteremias when used as interdialytic antibiotic lock solutions (118–120). These practices have not yet gained wide use but may hold promise for decreasing infection rates in the future. Several studies have shown that initiation of best practice techniques and staff education can improve outcomes for CRB, including decreased antibiotic treatment failure and decreased incidence of catheter infections (110,121,122). In a retrospective analysis of 872 patients during more than 476 patient catheter years, Sedlacek et al. (123) found that aspirin therapy, the 325-mg dose, was associated with a decrease in CRB caused by S. aureus. This effect was not seen with other antiplatelet agents, at a lower dose of aspirin, or for pathogens other than S. aureus. Tunnel dialysis catheters may also be heparin-coated based on studies in central venous catheters that documented a decreased rate of thrombosis and infection (124); however, clinical studies in dialysis populations did not show an effect on dysfunction and mixed effects on CRB rates (125,126).
Emerging Drug Resistance
Since 1996, five vancomycin-indeterminate Staphylococcus aureus (VISA) strains, vancomycin minimum inhibitory concentration (MIC) = 8 to 16 mg/L, have been identified worldwide. Vancomycin-resistant Staphylococcus aureus (VRSA), vancomycin MIC ≥32 mg/L, have been reported in the United States between 2002 and 2005 (127). Activity against these organisms is reported with new antimicrobials including ceftobiprole, quinupristin/dalfopristin, linezolid, and daptomycin. An older drug, trimethoprim-sulfamethoxazole, appears to be active against some of these organisms as well (128). Cases of resistance to linezolid and daptomycin have already been documented (129). Preventing transmission of antimicrobial-resistant microorganisms is of paramount importance in limiting the occurrence of these infections.
Autogenous and Prosthetic Arteriovenous Accesses
The creation of an AV access, either by direct anastomosis or by insertion of a prosthetic or biologic graft connecting an inflow artery with an outflow vein, fundamentally changes the existing flow and pressure states of the involved vasculature. Normal brachial artery blood flow is about 30 to 50 mL/min, while radial artery flow is as low as 10 to 15 mL/min. After anastomosis to a suitable graft or vein, flow rates in a 2-mm-diameter radial artery may increase to 800 mL/min. The associated shear stress will lead to arterial remodeling and over time to a larger diameter artery. The larger artery again will allow for higher flow rates at similar blood pressures. Arterial blood-flow wave forms change from triphasic before access creation to biphasic. The access vein experiences very similar changes in blood-flow volume, from less than 10 mL/min in a forearm cephalic vein to 400 to 800 mL/min within several weeks after access creation. Continuous flow changes to pulsatile flow near the anastomosis, which becomes less pulsatile with increasing distance from the anastomosis and addition of collaterals to the outflow network. Veins similarly remodel outward if exposed to the right shear stress and intra-access pressures, remodel little with low flows and pressures, and may form a stenosis with shear stress and intra-access pressures too high. The exact relationship between the different components of the vessel wall and the physiologic stimuli related to blood flow (shear stress and pressure) is currently not fully understood on the molecular level.
Stenoses at the inflow or outflow side of an access affect flow volume as well as intra-access pressures and thereby the biology of the involved vasculature. Recurrent outflow stenoses cause prolonged exposure to elevated intra-access pressures. Healing of needle insertion sites under elevated pressure leads to apposition of additional cell layers and connective tissue, which clinically manifests as aneurysm at the needle insertions sites. Over time, the skin thins and consists mostly of scar tissue. In this state, the reduced elasticity predisposes for prolonged bleeding after needle removal as well as scabbing and eventually ulceration of the overlying skin which may manifest as a terminal bleeding event.
While access stenoses can be treated with angioplasty, stents, or surgical revision, they are highly stenoses and are highly recurrent (outflow more so than inflow), such that this is properly referred to as chronic access disease.
Maturation of an autogenous access is defined by its usability for repeated needle insertion and removal. This requires a certain thickening of the vessel wall that is a result of shear stress and pressure-induced remodeling. Augmentation of the access vessel is necessary to avoid penetrating needle insertions and may be weak if there is an inflow stenosis or collaterals in or right after the needle insertion segment. Increased intra-access pressures (pulsatility) may lead to early infiltration and prolonged bleeding and can be found with high inflow (mismatch with access outflow) or an outflow stenosis.
Physical Examination of Vascular Access
Dialysis units may call to refer a patient for a variety of specific complaints:
• “Needles are pulling clots.”—There may indeed be thrombus forming in a needle insertion segment, but this is rare. More commonly, needle tip position is such that the tip or the back hole is not fully within the access lumen; thrombus forms in the adjacent tissue from blood leaking from the needle. This is primarily a sign of difficulty with needle insertion. Most commonly, augmentation of the access vessel is impaired, either due to a collateral or an anastomotic or juxta-anastomotic inflow stenosis. An alternative is the presence of a very small vein diameter as the length of a dialysis needle bevel is about 4 mm such that the tip can easily cause injury of the posterior wall.
• “Vessel collapses,” “access spasms”—During the first needle insertions into an access or a new segment of an access, there may be spasm of the vein at the needle insertion site. Typically, this resolves over the course of 20 to 30 minutes. However, intermittent collapse of the inflow vessel during the entire dialysis treatment may be a sign of access flows near or below dialysis pump speed. This can be seen with a true inflow stenosis or when a needle with the tape holding it in place compresses a flow-regulating inflow narrowing in a way that otherwise sufficient blood flows are reduced to or below pump speed. This can be exacerbated by periods of low systemic blood pressures which also affect access flows.
• “Long bleeding,” “bleeding on a nondialysis day”—Bleeding from an access in such fashion is due to increased intra-access pressures, skin deterioration, anticoagulation, or a combination of the three. Other signs of a stenosis such as changes in pulsatility and location of a thrill should be determined.
The physical examination is instrumental in guiding imaging evaluation as well as correlating observed angiographic abnormalities with clinical dysfunction to counter the “oculo-manual” reflex for intervention. Each dialysis access presents with unique features as a result of smallest inflow diameter (flow-limiting/regulating segment), blood pressure, outflow capacity, and presence of collaterals. To capture all (patho-)physiologic components of a given access by physical examination, we suggest to evaluate the following five categories: pulsatility, flow murmur/bruit, thrill, augmentation, collapse of access against gravity (TABLE 3.9). Repeated examinations and correlation of physical findings with angiographic features as well as flow measurements will lead to useful mastery of this skill. Collapse of access against gravity can usually not be examined in prosthetic accesses, except if there are well-established needle insertion segment where the surface layer of PTFE has rarified over time (needle insertion aneurysm).
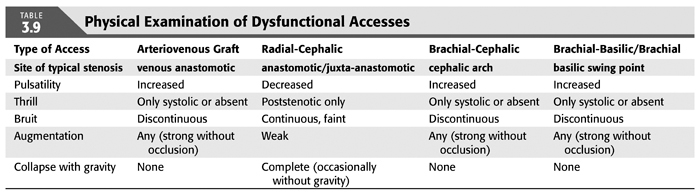
Pulsatility describes the force of access expansion during systole and the degree of softening during diastole. Very high blood pressures will suggest increased pulsatility, but the access softens remarkably during diastole. An outflow stenosis will lead to increased pulsatility and reduced softening during diastole. An inflow stenosis will blunt the systolic component and create the impression of an “empty” access during diastole, unless there is a coexisting outflow stenosis. Pressure measurements in the body of accesses (needle insertion segment) with “normal” pulsatility typically are in the 20 to 25 mm Hg range for forearm accesses and 25 to 30 mm Hg for upper arm accesses.
The audible flow murmur can be characterized by pitch and continuity. A change in pitch toward higher frequency is typical at the site of a stenosis due to accelerated flow velocity at this site. A discontinuous flow murmur indicates that during diastole, flow is so low that no audible shear force is created. This is the sign of a severe inflow or outflow stenosis. Typically, the stenotic inflow murmur is faint (like a whistle), while the stenotic outflow murmur can be coarse and loud (akin to a wood saw).
A thrill is palpable through the skin when the vessel is close enough to the surface and the flow high enough in relation to the diameter of the vessel to create vibration of the vessel wall. A thrill can be the sign of a well-developed access, usual in the inflow segment, dissipating as the access vessel branches and takes a deeper course. It is usually continuous with slight pronunciation during systole. In contrast, a discontinuous thrill can be found with severe stenosis. An isolated thrill is also found focally immediately after a stenosis. The differentiation from a “healthy” thrill can be made by documenting a change in pulsatility at the site of the focal thrill, increased retrograde (inflow side), and decreased antegrade (outflow).
Augmentation is the firming up and enlargement of the body of the access (where needles are inserted) with occlusion of the outflow. Without proper augmentation, needle insertion will be complicated by perforating injuries and hematomas. An inflow stenosis will impair augmentation as will side branches and collaterals between the occluding finger/tourniquet and the inflow. The location of side branches can be elucidated by moving the occluding finger closer toward the anastomosis until augmentation is achieved. With several collaterals, this may be a staged phenomenon.
Collapse of the access with arm elevation (against gravity) is a measure of inflow and outflow capacity match or mismatch. A forearm access typically displays complete collapse, while upper arm accesses typically show only partial collapse. Outflow stenoses or very high inflow will decrease the degree of collapse; banding of an upper arm access or a natural flow-limiting stenosis may lead to complete collapse of an upper arm access.
Enlarged needle insertion sites (and any sites of suspected skin thinning) are best examined while occluding the inflow: The completely empty access allows palpation of firm, layered thrombus inside aneurysms as well as better appreciation of the thickness of the overlying skin by rolling it between thumb and index finger.
Diagnostic imaging includes use of ultrasound as well as angiography using iodinated contrast agents or CO2 gas. Ultrasound can accurately determine the depth of an access, demonstrate intraluminal thrombus, and provide estimates of access flow by measuring flow in the inflow artery. Angiography provides patterns of flow distribution, unmasking relevant side branches and stenoses. During angiography, access flows can be directly measured using special endovascular catheters based on a modified Fick’s equation.
Guided by physical examination, angiographic studies and interventions can be performed with minimal contrast volume (5 to 10 mL), which typically does not jeopardize renal function even in patients with advanced CKD. We dilute contrast 1:1 with normal saline for central venous imaging and 1:3 with normal saline for peripheral images and perform pullback angiograms from central to peripheral (the usual access entry site). Diluted contrast allows for better appreciation of vascular details otherwise hidden by dense contrast.
Decisions to intervene on a given access have to be based on a sum of clinical findings, physical examination, and requirements for dialysis. For instance, a pre-ESKD patient with a stable inflow stenosis and relatively low flows may be best served with observation rather than recurrent interventions. Care systems have to be created to allow treatment of these access issues immediately before or at initiation of dialysis.
Natural History of Access Function and Dysfunction
First described by Brescia et al. (4) in 1961, the autogenous AV access remains the best form of long-term vascular access. AVFs are the preferred type of dialysis access because they are associated with the lowest morbidity and mortality, have excellent long-term patency, and require the fewest number of interventions to maintain patency. This is in particular true for forearm autogenous accesses (130). It is for these reasons that the NKF/KDOQI guidelines have set a goal of 65% of all hemodialysis patients having a functional AVF which we are soon approaching. The Fistula First Breakthrough Initiative also seeks to increase the use of AVFs for access while simultaneously decreasing the use of central venous catheters. In the United States, without dedicated programs to detect access dysfunction early and treat it appropriately, maturation failure is high (131). Even with interventions, some autogenous accesses do worse than others, require more interventions to function adequately, and still have higher failure rates (132), but accesses with low flow or maturing accesses seem to benefit from interventions (133). Once mature, native fistulas have excellent long-term patency rates and rarely become infected. The authors have seen primary AVFs provide adequate vascular access for 20 years.
The three dominant types of fistulas used in dialysis are the forearm radial-cephalic autogenous access, the upper arm brachial-cephalic or proximal radial-cephalic autogenous access, and the upper arm transposed brachial-basilic access.
Radial-cephalic forearm access is the preferred access if there is an appropriate cephalic vein and associated radial artery. The radial artery ideally will be without calcifications; however, even calcified radial arteries with 2-mm diameter may deliver 600 to 800 mL/min blood flow. The cephalic vein usually branches near the elbow with outflow toward the upper arm basilic, brachial, and cephalic veins, ensuring low intra-access pressures. If only one outflow is available in the upper arm, basilic/brachial is preferred over upper arm cephalic vein outflow as the cephalic arch has a tendency to develop stenoses over time. Maturation of the access to usability is most commonly impaired by juxta-anastomotic stenoses in the swing segment of the cephalic vein as well as lack of augmentation in the setting of multiple collateral veins. We prefer side-branch ligation over coil embolization as the veins are typically very superficial and easy to reach, and the foreign body sensation of a coil in the forearm can be limiting.
The upper arm brachial-cephalic, or proximal radial-cephalic, access is created in the antecubital area where the cephalic vein is mobilized for an end-to-side anastomosis to the brachial artery. A similar anastomosis can be fashioned using the proximal radial artery which aids in limiting flow and preventing steal. In some instances, a Gracz or Konner access (134) can be created by connecting the perforans branch to the deep venous system for an anastomosis with the proximal radial artery. Accesses utilizing the upper arm cephalic vein very often develop a chronically recurrent cephalic arch stenosis and less frequently also in the cephalic vein swing segment. Stenoses of the cephalic arch can be effectively treated with cephalic arch stent grafts (135).
An upper arm brachial-basilic superficialized access can be created as a two-stage or a single-stage procedure. A single-stage procedure involves mobilization of the upper arm basilic vein including a forearm branch after an incision from axillary to elbow, tunneling of the vein in a curve toward the anterior aspect of the arm with anastomotic area to the brachial artery near the elbow. Instead of tunneling, the vein can just be lifted within the skin flap. Ideally, the vein should come to rest away from the scar of the incision to allow easy palpation and needle insertion. In the two-stage procedure, a forearm branch of the basilic vein below the elbow is anastomosed to the proximal segment of a forearm artery or the brachial artery. This allows the vein to remodel and once there is wall thickening as well as increase in diameter, which is usually after 6 to 8 weeks, when the formal transposition can be done. Tunneling the vein to a new site of anastomosis with the brachial artery has often the best anatomic results. The vein can usually be cannulated 4 to 6 weeks after transposition. Typical sites for stenoses in this access are the “swing” point of the basilic vein as well as the inflow segment. Two-stage accesses appear to mature more frequently.
Other autogenous accesses that can be done are a superior femoral–saphenous vein access in the thigh, but the longevity of type of access over time has not been as good as upper (fore-)arm accesses. A superficial femoral-superficial femoral transposition thigh access is very similar to a basilic vein transposition in the arm and may have very high flows due to the larger vessels involved.
Non-autogenous accesses typically use expanded PTFE (ePTFE) (prosthetic) and less frequently bovine carotid artery, cryoveins, or tissue-engineered tubes with human cells (biograft). They are placed in the forearm in either a loop (brachial or proximal radial artery to cubital median, basilic, brachial, or cephalic vein) or straight (distal radial artery to vein) configuration. Within 3 to 4 weeks, fibrous tissue secures the graft in its subdermal tunnel and helps achieve hemostasis at needle puncture sites. Over time, the PTFE graft material wears out at site of repeated needle puncture. More recently, early cannulation ePTFE grafts have become available that allow cannulation within days after placement, thereby reducing the need for tunneled catheters.
Grafts can be placed in many sites—after exhausting autogenous options and typical placement sites in the upper extremities, chest wall (axillary artery to axillary vein and axillary artery to jugular vein), or thigh (femoral artery to femoral vein), grafts are possible. FIGURE 3.8 depicts a looped forearm graft and an upper arm graft.
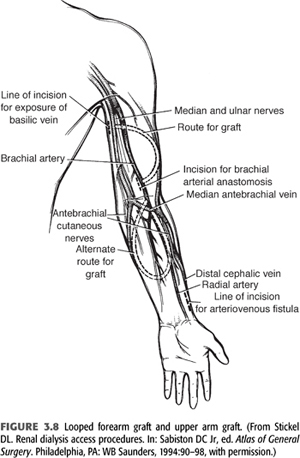
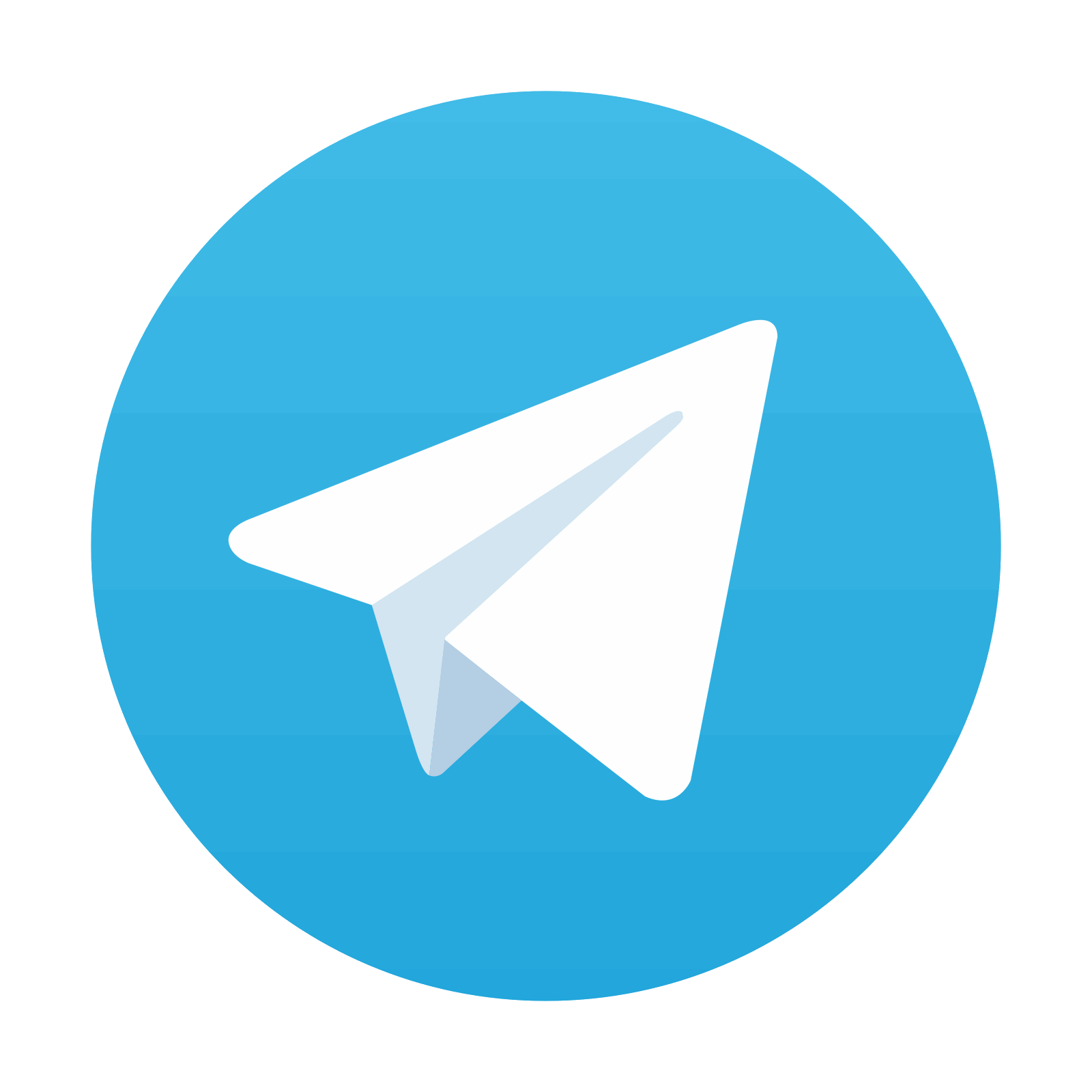
Stay updated, free articles. Join our Telegram channel

Full access? Get Clinical Tree
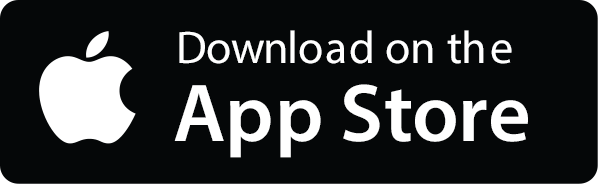
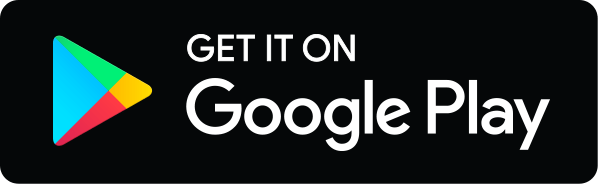