INTRODUCTION
One of the more difficult concepts to grasp in nephrology is that disorders of ECF volume are the result of disturbances in sodium balance and that disorders of sodium concentration (hypo- and hypernatremia) are the result of disturbances in water balance. The control of ECF volume is dependent on the regulation of sodium balance. Sodium concentration alone is not reflective of ECF volume status. This is illustrated graphically by the cases in Figure 2.1. Patient A has diarrhea (sodium concentration of diarrheal fluid is approximately 80 mEq/L) but does not have free access to water and the ECF volume as a result is depleted 3 L from its starting point of 14 L. The serum sodium concentration rises to 170 mEq/L. Patient B has an equivalent amount of diarrhea but is awake, alert, and has free access to water. Patient B drinks enough free water to increase ECF volume from 11 to 13.2 L. Sodium losses in the diarrheal fluid coupled with free water replacement results in a serum sodium concentration of 130 mEq/L. The serum sodium concentration is high in case A and low in case B, yet in both patients ECF volume is decreased. These cases illustrate that serum sodium concentration, in and of itself, does not provide information about the state of ECF volume. In both patients, sensor mechanisms detect ECF volume depletion and effector mechanisms are activated to increase renal sodium reabsorption.
FIGURE 2-1. Sodium concentration does not reflect ECF volume status. Both of the patients shown have decreased ECF volume but in case A (no fluids taken in) serum sodium concentration is increased, while in case B (losses replaced with water) the serum sodium concentration is decreased. Abbreviations: GI, Gastrointestinal; NPO, nothing by mouth (nil per os).
ECF volume reflects the balance between sodium intake and sodium excretion and is regulated by a complex system acting via the kidney. The average intake of sodium in developed countries is between 150 and 250 mEq/day and must be balanced by an equivalent daily sodium excretion.
States where ECF volume is increased are related to a net gain of sodium and often present with edema in the presence or absence of hypertension. States where ECF volume is decreased reflect a total body sodium deficit and are often a result of sodium and water losses from the gastrointestinal or genitourinary tracts and commonly present with decreased blood pressure.
A normal person maintains sodium balance without edema, hypertension, or hypotension across a broad range of sodium intake (10 to 1000 mEq/day). A variety of sensors detect alterations in sodium balance and effectors respond by adjusting renal sodium excretion (Table 2.1). Sodium sensors respond to the adequacy of intravascular filling and the effector limb modifies sodium excretion accordingly. When patients are edematous, however, there is sodium retention even in the setting of expanded total body sodium and water content.
TABLE 2-1. Sensors and Effectors of Sodium Balance
This phenomenon led to the postulation of an important but confusing concept known as the effective arterial blood volume (EABV) that is defined based on the activity of the sodium homeostasis effector mechanisms in kidney. EABV is a concept rather than an objectively measured volume. Because stimulation of the sensory limb of the system cannot be directly measured, its activity is inferred based on the response of the effector limb. It is an estimate of the net level of stimulation of all sodium and volume sensors. Volume sensors in the arterial and venous circulation, including the renal vessels, monitor the sense of fullness of the vascular tree. Ultimately, it is the relationship between cardiac output and peripheral vascular resistance that is sensed. EABV can also be defined based on how far the mean arterial pressure (estimated as the diastolic blood pressure plus one-third of the pulse pressure) is displaced from its set-point. In many edematous disorders the set-point is normal, as in CHF and cirrhosis of the liver, and the mean arterial pressure tends to be low. In nephrotic syndrome, the set-point is increased by kidney disease and the mean arterial pressure is high. Despite the fact that mean arterial pressure is high, it still remains below the set-point. In both situations the kidney retains salt and water in an attempt to return blood pressure to its set-point. In clinical practice, however, net renal sodium handling determines the state of the EABV. When the kidney retains sodium, it is inferred that EABV is decreased and when the kidney excretes sodium, it is inferred that EABV is increased.
KEY POINTS
EFFECTOR SYSTEMS
Regulation of Sodium Transport in Kidney
When ECF volume is decreased, renal sodium excretion is minimized by decreasing the amount of sodium filtered and increasing tubular sodium reabsorption. ECF volume depletion stimulates the release of angiotensin II (AII), aldosterone, and arginine vasopressin (AVP), as well as activates the sympathetic nervous system resulting in salt and water retention. Thirst and the craving for salt are also stimulated. AII and aldosterone act synergistically to stimulate salt appetite and AII is a strong stimulator of thirst. Extrarenal salt losses are minimized by decreased sweating and fecal losses. Decreased ECF volume decreases intravascular volume and results in decreased renal perfusion. The resultant decline in glomerular filtration rate (GFR) decreases the filtered sodium load (amount presented to the proximal tubule). Tubular sodium reabsorption is increased by activation of the renin-angiotensin-aldosterone system (RAAS), activation of the sympathetic nervous system, changes in peritubular physical forces, and suppression of natriuretic peptides.
The filtered sodium chloride load is 1.7 kg/day. This is 11 times the amount of sodium chloride in the ECF. Less than 1% of the filtered load is excreted in the final urine under the control of a complex system of effector mechanisms that regulate sodium reabsorption along the nephron. The cellular and molecular mechanisms of action of these effector systems in each nephron segment are discussed below.
Proximal Tubule
The proximal tubule reabsorbs 60% to 70% of the filtered sodium chloride load. Physical factors, the sympathetic nervous system, and the RAAS regulate sodium reabsorption in this segment. The principal pathway for sodium entry into the proximal tubular cell is the Na+-H+ exchanger (isoform NHE3).
Physical factors regulate sodium reabsorption through changes in filtration fraction (FF) that create hydrostatic and oncotic gradients for water movement. The FF is the ratio of the GFR to renal plasma flow rate (RPF) shown in the equation below:
Efferent arteriolar constriction by All increases the FF via 2 mechanisms. It reduces renal blood flow (decreases RPF) and increases glomerular capillary pressure, which is the main determinant of GFR (raises GFR). The resultant increase in FF increases oncotic pressure and decreases hydrostatic pressure in the peritubular capillary. Recall that the kidney has 2 capillary networks in series. Blood leaving the glomerular capillary network via the efferent arteriole then enters the peritubular capillary network that bathes the proximal tubule. These changes promote the movement of salt and water from the tubular lumen to the interstitial space and finally into the peritubular capillary. In addition, AII reduces medullary blood flow, which has similar effects on driving forces in medullary nephron segments.
The RAAS also has direct effects on tubular transport mediated via NHE3 and the Na+-K+-adenosine triphosphatase (ATPase). AII and aldosterone both upregulate NHE3. The AII effect may be mediated via protein kinase C, whereas aldosterone was shown to increase insertion of preformed transporter proteins into the apical membrane. The Na+-K+-ATPase, which is present in the basolateral membrane of all nephron segments and is the major pathway by which sodium exits tubular cells, is also stimulated by AII. The sympathetic nervous system and insulin also stimulate the movement of NHE3 to the apical membrane and increase proximal tubular sodium reabsorption.
Systemic blood pressure itself also plays a key role in proximal tubular sodium reabsorption. As blood pressure rises the renal excretion of NaCl increases in an attempt to reduce ECF fluid volume and normalize blood pressure. This phenomenon is known as pressure natriuresis. Pressure natriuresis is not mediated by an increase in filtered sodium load. An acute rise in blood pressure does not change the amount of sodium filtered by the glomerulus as a result of autoregulation of the renal microvasculature. As blood pressure increases, the afferent arteriole constricts so as to maintain glomerular capillary hydrostatic pressure constant. Afferent arteriolar constriction results from both a direct myogenic reflex and tubuloglomerular feedback (discussed below). Acute rises in blood pressure are sensed in the vasculature and a signal is transmitted to the proximal tubule to reduce sodium chloride reabsorption. This is mediated by removal of NHE3 from the luminal membrane of proximal tubule via a 2-step internalization process, regulated in part by AII, shown in Figure 2.2. NHE3 first moves from the microvillar membrane to the intermicrovillar cleft (first step) and then from the intermicrovillar cleft to subapical endosomes (second step). A fall in AII concentration plays a role in the first step. Na+-K+-ATPase activity is also decreased via a similar process of internalization.
FIGURE 2-2. Sodium transporters in proximal tubule and pressure natriuresis. NHE3 (filled circles) is internalized in 2 steps in response to elevated blood pressure. In step 1, NHE3 moves from microvilli to the intermicrovillar cleft, a process that is regulated by AII. In step 2, NHE3 moves from the intermicrovillar cleft to proteosomes and is degraded. The Na+-K+-ATPase is regulated in a similar fashion.
Increased NaCl delivery to the thick ascending limb of Henle is sensed by macula densa cells. The macula densa is a specialized region near the junction of the cortical thick ascending limb and distal convoluted tubule (DCT). The macula densa is in close proximity to the granular renin-producing cells in the afferent arteriole, and together this region is referred to as the juxtaglomerular (JG) apparatus. The JG apparatus mediates a process known as tubuloglomerular feedback. When increased sodium chloride delivery is sensed by the macula densa a signal is transmitted to the afferent arteriole to constrict and single-nephron GFR decreases. Renin release by the JG apparatus is suppressed and AII levels fall if the signal is sustained. Conversely, when sustained sodium chloride delivery is sensed by the macula densa renin release is stimulated and the RAAS activated. Tubuloglomerular feedback serves 2 purposes. First, it maintains sodium chloride delivery to distal nephron segments (DCT, connecting tubule, and collecting duct) relatively constant over a wide range of conditions in the short term. It is in distal nephron where the final fine-tune regulation of sodium and water balance occurs. Additionally, in the long term the JG apparatus is responsible for controlling renin secretion at a rate that is optimal so as to maintain sodium balance.
Thick Ascending Limb of Henle
The thick ascending limb of Henle reabsorbs 20% to 30% of the filtered sodium chloride load. Sodium and chloride enter the thick ascending limb cell via the Na+-K+-2Cl– cotransporter, which is inhibited by loop diuretics. Because sodium and chloride concentrations in urine are much higher than potassium, for the transporter to operate maximally there must be a mechanism present for potassium to recycle back into the tubular lumen. A ROMK potassium channel in the luminal membrane mediates potassium recycling. Sodium leaves the cell via the Na+-K+-ATPase and chloride via a chloride channel.
The rate of NaCl reabsorption in this segment is load dependent. The higher the delivered NaCl load the higher the reabsoprtion. Sodium reabsorption is increased by activation of the sympathetic nervous system and β-adrenergic agonists, AVP in some species, parathyroid hormone, calcitonin, and glucagon. Prostaglandin E2 inhibits sodium reabsorption.
Distal Convoluted Tubule
The DCT reabsorbs 5% to 10% of the filtered sodium load. Sodium and chloride enter the DCT cell via the thiazide-sensitive Na+-Cl– cotransporter (NCC) and sodium exits via the Na+-K+-ATPase. For mineralocorticoids to play a role in the regulation of sodium transport in any nephron segment, that segment must also express the mineralocorticoid receptor and type 2 11β-hydroxysteroid dehydrogenase (HSD). The mineralocorticoid receptor is expressed in the entire DCT, while type 2 11 β-HSD is expressed in the later half (DCT2) of the DCT. DCT2 also contains the epithelial sodium channel (ENaC). Type 2 11β-HSD degrades cortisol to the inactive cortisone in mineralocorticoid target tissues. This is required to maintain mineralocorticoid specificity, given the facts that the mineralocorticoid receptor binds glucocorticoids and that glucocorticoids circulate at much higher concentrations than mineralocorticoids.
Genetic studies of a rare monogenic disorder provide insight into NCC regulation. Familial hyperkalemic hypertension (FHH), also known as pseudohypoaldosteronism type II (PHA II), is an autosomal dominant disease characterized by hypertension, hyperkalemia, and extreme sensitivity to thiazide diuretics. Mutations in 2 members of the WNK (with no lysine [K]) kinase family, WNK1 and WNK4, cause the disease. Three members of this gene family WNK1 (also known as long WNK1), 2, and 4 are expressed in kidney. In addition, an alternatively spliced isoform of WNK1, kidney-specific WNK1 (KS-WNK1) is also expressed. Their expression pattern varies: long WNK1 (L-WNK1)—all along the distal nephron; KS-WNK1—in the DCT and decreases gradually in the connecting tubule; WNK3—along the entire nephron; and WNK4—DCT1 to the collecting duct. WNK4 reduces expression of NCC in the cell membrane. It does this via a kinase-dependent mechanism that does not involve changes in the synthesis or processing of NCC. Mutations in WNK4 lead to NCC overactivity via a loss of function mechanism of WNK4. Recently, other kinases including SPAK (Ste20p-related proline-alanine rich kinase) and OSR1 (oxidative stress response) have been identified as intermediates in the pathway between WNK4 and NCC. WNK4 inhibits the ROMK potassium channel. ROMK inhibition is not dependent on WNK4 kinase activity but occurs through clathrin-dependent endocytosis of the channel. Mutations result in a gain of function of this process with further increases in endocytosis from the luminal membrane.
In collecting duct, WNK4 increases claudin phosphorylation, resulting in increased paracellular chloride transport and stimulation of ENaC activity. FHH mutations further increase both of these processes and augment NaCl reabsorption. Interestingly, the WNK4 mutations of FHH increase NCC activity but decrease ROMK activity. This not only explains the hypertension and hyperkalemia of FHH but also shows that WNK4 can differentially regulate NCC and ROMK.
Aldosterone production is stimulated by both hypovolemia and hyperkalemia. In hypovolemia the distal nephron must reabsorb sodium but not increase potassium secretion, whereas in hyperkalemia the goal is to secrete potassium without an effect on sodium homeostasis. How 1 hormone can mediate 2 apparently disparate functions has been termed the aldosterone paradox. When aldosterone concentrations are elevated, how does the distal nephron know whether to reabsorb sodium (stimulate NCC and inhibit ROMK) or excrete potassium (stimulate ROMK and inhibit NCC)? WNK4 may be the master switch that regulates the balance between NaCl reabsorption and potassium secretion in the distal nephron. With hypovolemia both AII and aldosterone are stimulated. AII even in the presence of WNK4 stimulates NCC. Experimental studies have also shown that AII stimulates the phosphorylation of SPAK and NCC. AII thereby activates NCC in DCT1 directly and in DCT2 indirectly via aldosterone. AII also inhibits ROMK activity through WNK4 dependent and independent mechanisms. The combined interaction of AII with aldosterone favors the electroneutral reabsorption of sodium with chloride.
By contrast, in hyperkalemia, aldosterone is stimulated but AII is not. WNK4-mediated inhibition of NCC in DCT1 is maintained because type II 11 β-HSD is not expressed in DCT1. This inhibition of sodium reabsorption in DCT1 results in sodium delivery further downstream to the connecting segment and collecting duct where sodium reabsorption via an electrogenic process (ENaC) can stimulate potassium secretion. S1169 phosphorylation mediated by Sgk1 (serum and glucocorticoid-regulated kinase) releases WNK4 inhibition of ENaC and ROMK in the connecting segment and collecting duct. In addition, Sgk1 phosphorylates Nedd4-2 on 3 motifs. This creates binding sites for the 14-3-3 protein that blocks interaction of Nedd4-2 with ENaC and prevents its ubiquitinization and subsequent removal from the luminal membrane.
L-WNK1 is expressed in a variety of chloride-transporting epithelia, including kidney, colon, sweat ducts, pancreas, and bile ducts. L-WNK1 does not appear to bind NCC but rather interacts with WNK4 and inhibits its ability to downregulate NCC. In FHH, mutations in L-WNK1 increase its expression and further augment its ability to inhibit WNK4, resulting in increased NCC activity. KS-WNK1 is stimulated by aldosterone and antagonizes the effects of L-WNK1. WNK3 increases NCC activity and inhibits the activity of ROMK and KCl cotransporters in the distal nephron and may also play a role in hypovolemia. In the model of DCT sodium transport shown in Figure 2.3, delivery of NCC to the luminal membrane is inhibited by WNK4, while L-WNK1 inhibits the activity of WNK4, and KS-WNK1 inhibits L-WNK1. Mutations in either L-WNK1 or WNK4 result in increased NCC activity and the FHH phenotype.
FIGURE 2-3. Simplified model of DCT sodium transport and FHH. The FHH phenotype is caused by mutations in both WNK4 and WNK1. WNK4 impairs delivery of the NCC to the luminal membrane by shunting the protein to a lysosomal compartment and mutations that decrease its activity increase NCC expression in the cell membrane. L-WNK1 interacts with WNK4 and decreases its activity. KS-WNK1 interacts with L-WNK1 and decreases its activity.
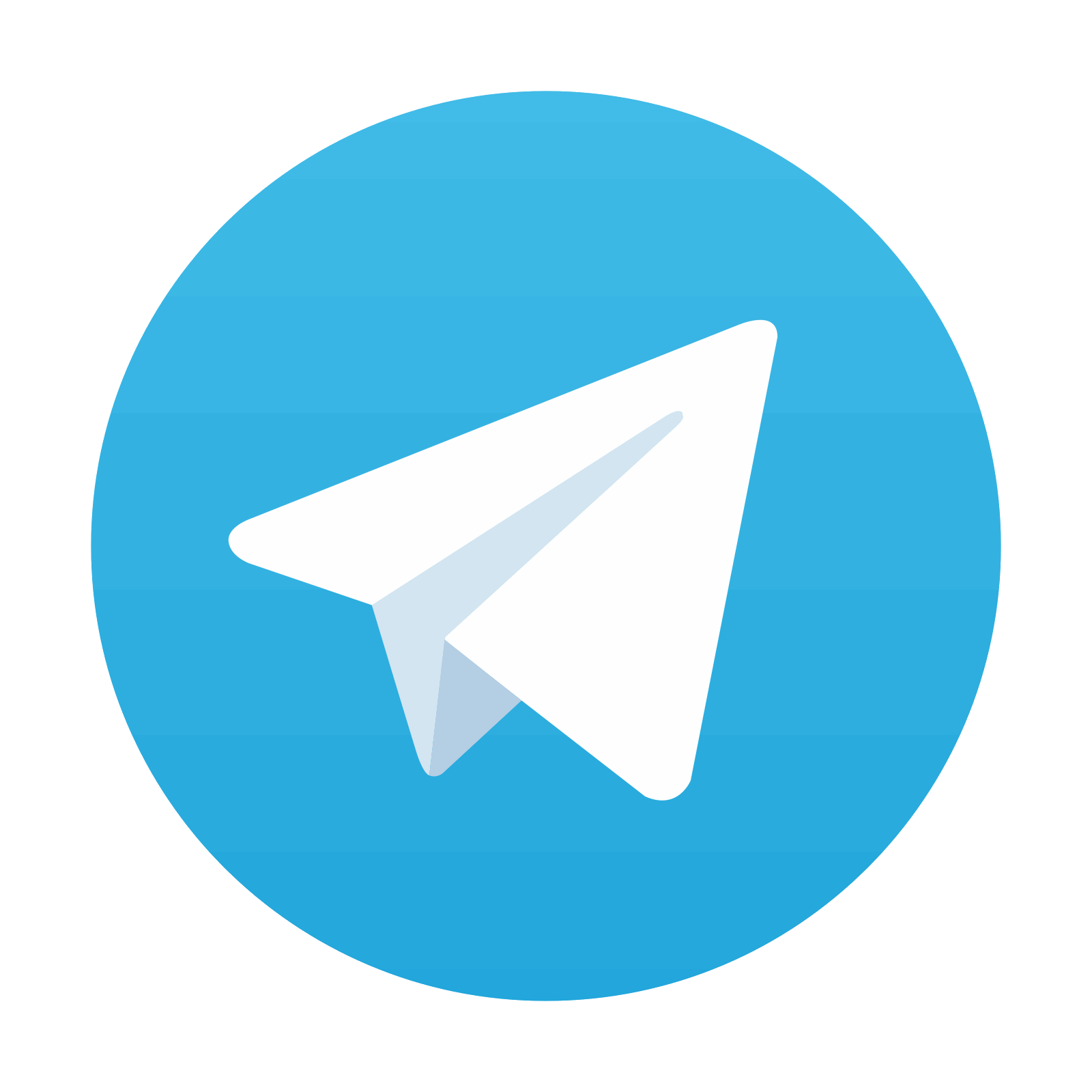
Stay updated, free articles. Join our Telegram channel

Full access? Get Clinical Tree
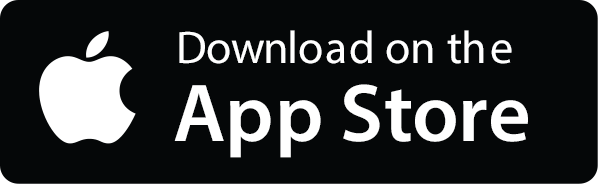
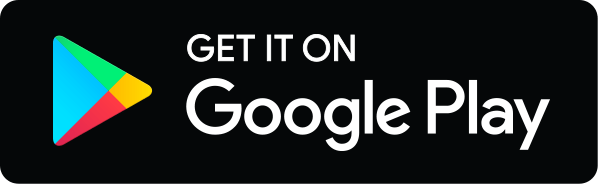