© Springer International Publishing AG Switzerland 2015
Luca Aldrighetti, Francesco Cetta and Gianfranco Ferla (eds.)Benign Tumors of the Liver10.1007/978-3-319-12985-3_33. Genetic Syndromes and Benign Liver Tumors
(1)
IRCCS MultiMedica, Milan, Italy
(2)
HPB Surgery and Liver Transplant Unit, Royal Free Hospital, University College of London, London, UK
A wide range of inherited syndromes or diseases related with congenital genetic alterations include liver tumors as a part of the syndrome [1–10].
Deficit of alpha 1 antitrypsin has been typically related to the occurrence of hepatocellular carcinoma (HCC), during early life, requiring liver transplantation. However, since A1AT is so closely associated with HCC, it will not be included in the present chapter, dealing specifically with benign liver tumors, even if genetic diseases due to a single gene alteration, determining a single enzymatic protein malfunction, which is subsequently responsible for hepatocellular carcinoma, could be one of the few examples of tumors that could be cured or prevented by genetic engineering. In fact, the possibility of substituting the mutated gene with its wild-type variant could represent a veritable example of HCC cured or prevented by genetic engineering.
One of the most important genetic syndromes associated with benign liver tumors is glycogen storage disease (GSD) [1–3]. Genetic GSD is caused by any inborn error of metabolism (genetically defective enzymes) involved in these processes. In the USA is it is estimated to occur in 1 per 20–25,000 births. GSD is an inherited autosomal recessive disorder characterized by intracellular accumulation of glycogen. There are 11 distinct diseases that are commonly considered to be GSD [1–3].
Type I GSD is the most common form and results from genetic mutations determining deficits in the glucose-6-phosphatase system. On the other hand, type III GSD is due to a defective glycogen debranching enzyme. Both diseases determine glycogenolysis alterations with increased glycogen accumulations in various districts, namely, in the liver and in the kidney [1, 2].
Epidemiologic studies showed that 22–75 % of patients with type I GSD develop hepatic adenomas, which are usually diagnosed by the second decade of life. On the contrary, the prevalence of hepatic adenomas is lower in type III GSD, where only 4.4–25 % of affected siblings develop HA. The estimated risk of malignant transformation of hepatic adenomas related with type I GSD is as high as 10 %, whereas the risk for malignant transformation is much lower in patients with type II GSD. In fact, only six patients have been reported up to now in the literature.
All these findings suggest that increased glycogen storage in the liver seems to induce hepatic tumorigenesis, likely altering glucose metabolism and glycogen synthesis. However, there is no detailed pathophysiological hypothesis concerning liver tumorigenesis from “metabolic alterations.”
Other hereditary diseases, acting as risk factors associated, namely, with hepatic adenomas [5–8] include: (1) galactosemia, (2) hepatic iron overload related to beta thalassemia, and (3) maturity-onset diabetes of the young type 3 (MODY3) [4].
Galactosemia is a rare autosomal recessive metabolic disorder, occurring in about 1 per 60.00 births. In individual with galactosemia, the enzymes needed for further metabolism of galactose are severely diminished or missed entirely, leading to toxic levels of galactose 1-phosphate in the various tissues, resulting in hepatomegaly and subsequent cirrhosis but also in an increased occurrence of benign liver tumors. Gene responsible for galactosemia include GALT, GALK1, and GALE, which are responsible for galactosemia type 1, 2, and 3, respectively.
Thalassemia is caused by variant or missing genes that affect hemoglobin synthesis. They include different variants considered as autosomal recessive blood disorders. People with thalassemia have microcytic anemia and develop significant complications, including iron overload, bone deformities, and cardiovascular diseases. Since it confers a degree of protection against malaria (selective survival advantage of heterozygous carriers), it is more frequent in the Mediterranean region where malaria was endemic. Iron overload determines the occurrence of liver alterations, also including hepatic adenomas.
MODY3 is determined by heterozygous germline mutations of the HNF1 alpha gene that is mapped at chromosome 12q24.2. This gene encodes for the hepatocyte nuclear factor 1 (HNF1) alpha, a 681 AA homeodomain transcription factor that is involved in hepatocyte differentiation. HNF1 alpha controls the expression of some liver-specific genes, such as beta fibrinogen, alpha 1 antitrypsin, and albumin. It has currently been considered as a tumor suppressor gene involved in liver tumorigenesis. In particular, biallelic inactivating mutations of HNF1 alpha have been reported in 35–40 % of HA. HNF1alpha mutations occurring in sporadic HAs are somatic in 90 % of cases. However, when occurring in patients with MODY3, they are related to the germline gene variant determining the disease [4].
Another type of genetic alteration that is strictly related with liver tumorigenesis, frequently determining the occurrence of benign hepatic tumors, is the complex termed “vascular alterations.” In addition to be responsible for the two most frequent benign liver tumors, i.e., hemangiomas and focal nodular hyperplasia (FNH), vascular alterations are integral to some genetic syndromes, determining congenital vascular malformations, also showing peculiar liver tumors or alterations.
Congenital vascular malformations associated with benign liver tumors include:
1.
Portal vein agenesis and/or extrahepatic congenital portosystemic shunts (Abernethy syndrome) [9]. It is a very rare congenital condition. The term “syndrome” means that there is a wide range of possible malformations, including partial or complete agenesis of the portal vein, associated with a different flow from the splanchnic district to the vena cava. In particular, the flow from splanchnic viscera usually bypasses the liver and drains directly into the inferior vena cava, left renal vein, iliac veins, or right atrium. This alteration is frequently associated with live tumors (up to 40 % of patients), including focal nodular hyperplasia, liver adenoma, and hepatocellular carcinoma. These lesions may coexist together in the same subject.
It has been hypothesized that alterations of the liver vascular perfusion can be responsible for the occurrence of all types of lesions, making the Abernethy syndrome an intriguing model for “alternative” liver tumorigenesis. In these patients, who may require surgery during childhood or the second decade of life, liver transplantation has been suggested.
2.
Hereditary hemorrhagic telangiectasia (HHT) (Rendu–Osler–Weber disease). Kasabach–Merritt syndrome, ataxia telangiectasia, and peliosis hepatis are other genetically determined vascular abnormalities, also involving the liver. HTT is an autosomal dominant genetic disorder characterized by widespread vascular malformations, including cutaneous, mucosal, and visceral telangiectasias that can involve the lung, brain, and liver. In patients with HHT, the liver shows increased hepatocellular regenerative activity. This could act as a potent stimulus for the development of FNH. In fact, in subjects affected by HHT, the prevalence of FNH is 100-fold greater than in the general population [10].
The putative role of vascular disturbances in the genesis of FNH is also supported by the observation of FNH-like lesions in patients with cirrhosis. In explanted livers of 130 cirrhotic patients, the presence of esophageal varices was significantly associated with the presence of FNH-like nodules, suggesting a contribution of portal hypertension to the formation of nodular hyperplastic lesions.
Recently, anecdotal evidence was published on the proposed sequence from hemangioma to FNH, probably starting with thrombosis of the hemangioma, followed by involution of the lesion and subsequent occurrence of a hyperplastic regenerative reaction. A child that was analyzed at the age of 6 months for a palpable hemangioma of the liver presented 5 years later with a lesion of unknown origin in the same topographic area of the organ. Surgical excision and pathologic examination revealed an FNH [11].
On the basis of these findings, therefore, the association between HHT and FNH further supports the vascular etiology not only of hemangiomas but also of FNH. The notion that FNH lesions develop secondary to vascular abnormalities is suggested by several observations. In some adults, the liver contains both hemangiomas and FNH. A study among 247 patients with hepatic masses identified 148 patients with FNH, of which 20 % had concomitant hemangiomas of the liver [12].
Benign liver tumors in patients with activating mutations of the beta-catenin gene or of the gp 130 gene. Beta-catenin-activating mutations have been identified in a number of HAs [5–8]. Beta-catenin mutations lead to an activation of the Wnt pathways, which plays a key role in liver development and physiology, involving functions such as lineage specification, differentiation, stem cell renewal, epithelial–mesenchymal transition, zonation, proliferation, cell adhesion, and liver regeneration. With mutation of the beta-catenin gene, there is an inappropriate upregulation of many of its targets, including glutamine synthetase (GS) and the G-protein-coupled receptor 49. This subsequent aberrant upregulation contributes to the development of cellular atypia and an increased risk of malignant transformation to HCC that is more frequent in this type of HA than other HA subtypes [5].
Beta-catenin mutations may determine similar effects as germline mutation of the APC gene, causing familial adenomatous polyposis (FAP) – an inherited multitumoral syndrome, responsible for the occurrence of hundreds to thousands of colonic polyps, evolving invariably toward colonic cancers, and of a wide range of other extracolonic manifestations, also including benign and malignant liver tumors. Beta-catenin and APC cooperate together in the Wnt pathway. Interestingly, patients with APC germline mutations do not have beta-catenin mutations, i.e., APC, and beta-catenin are mutually excluding of each other in disrupting the Wnt pathway. In addition, no HA cases with both beta-catenin-activating and HNF1 alpha biallelic inactivating mutations have been observed, suggesting that these two tumorigenic pathways are also mutually exclusive. Finally, it is of interest that beta-catenin mutations are also found in 20–34 % of patients with HCC [5–8].
Gene expression data reveal an activation of the beta-catenin pathway in FNH. Beta-catenin is regarded as a pivotal stimulus for the proliferation of hepatocytes and a master actor of liver development and liver regeneration after injury, as well as a master regulator of liver zonation. In FNH, beta-catenin shows a heterogeneous distribution along the hepatocellular nodules in the absence of inactivating mutations of the gene and in the absence of alterations in the Wnt signaling pathway [5].
In normal hepatocytes, beta-catenin and its known target genes, such as GS, are expressed exclusively in the immediate perivenous areas. This zonated defined pattern of expression differs from that observed in beta-catenin-activated HA or HCC, which usually shows more diffuse cytoplasmic GS staining and nuclear beta-catenin staining, in accordance with the monoclonal origin of the beta-catenin-activating mutations in these tumors. In FNH, beta-catenin activation shows a characteristic architectural distribution on relatively large areas, centered on visible veins. In contrast to activating mutations, hepatocytes in FNH remain sensitive to repression of the beta-catenin pathway in regions surrounding fibrosis [5–8].
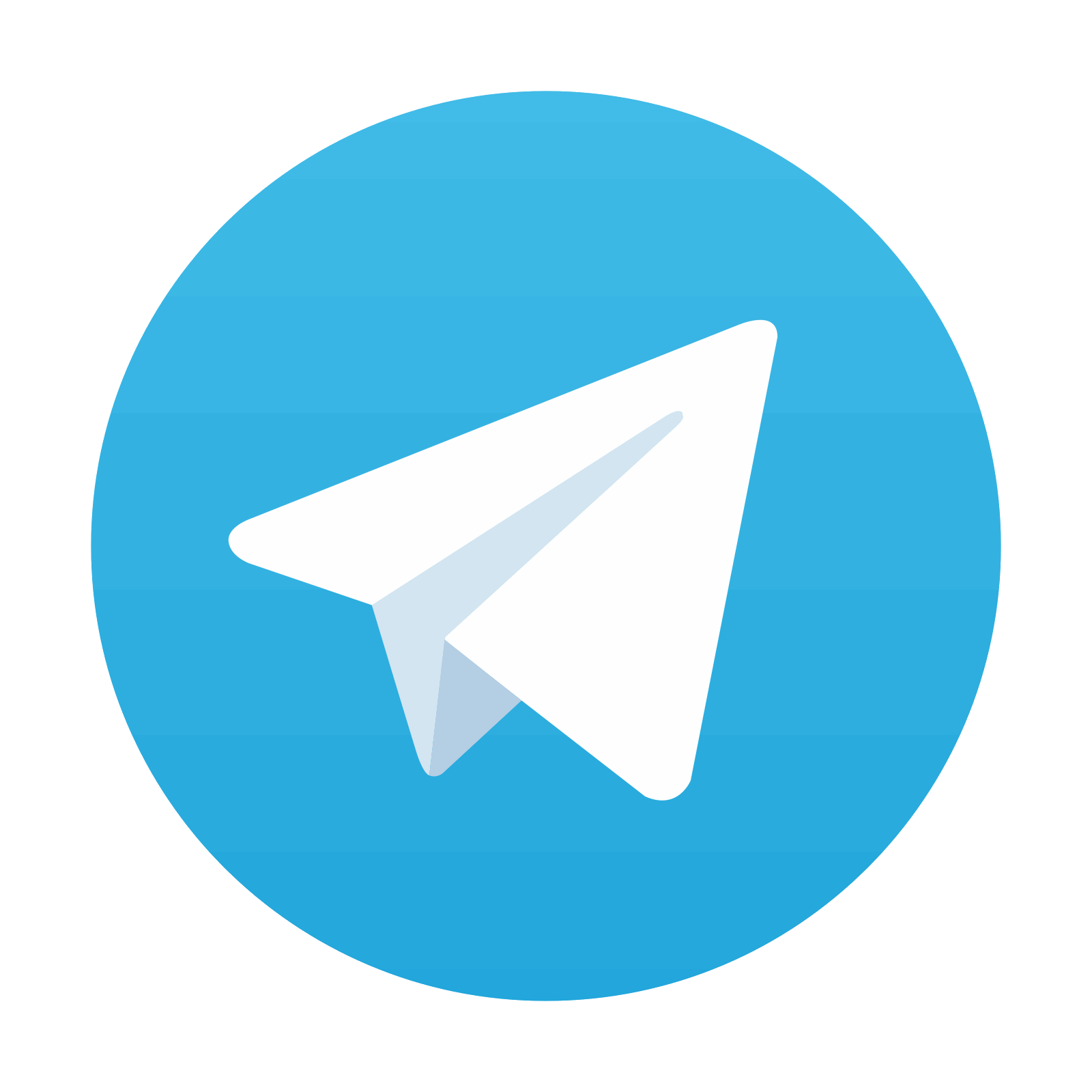
Stay updated, free articles. Join our Telegram channel

Full access? Get Clinical Tree
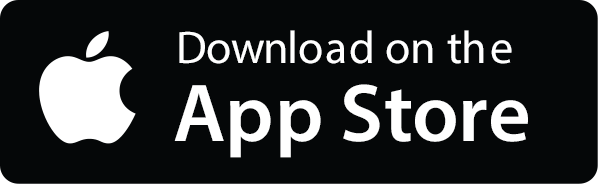
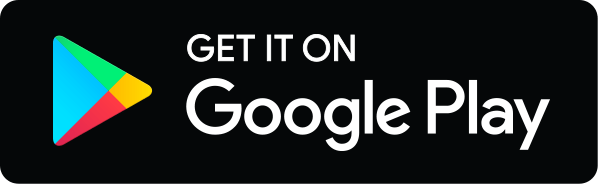