© Springer International Publishing AG Switzerland 2015
Luca Aldrighetti, Francesco Cetta and Gianfranco Ferla (eds.)Benign Tumors of the Liver10.1007/978-3-319-12985-3_44. Liver Pathophysiology: Liver Regeneration, Vascular Alterations, and Ischemia/Reperfusion
(1)
IRCCS MultiMedica, Milan, Italy
(2)
HPB Surgery and Liver Transplant Unit, Royal Free Hospital, University College of London, London, UK
The liver is the only solid organ that has a tremendous capacity for restoration of tissue loss by regeneration. Liver regeneration is a highly controlled process and stops once the volume of the lost tissue is restored [1–11].
Also, the liver is unique in a sense that it is the only organ that receives dual blood supply from two different sources, one of which is portal circulation. It has been assumed that liver functions, including the regenerative capacity, are remotely controlled by the splanchnic organs, including intestine and intestinal microbiota, pancreas, and also the spleen [1–6].
Surgical resection of hepatic lobes or hepatocyte loss caused by viral or chemical injury triggers hepatocyte replication, while enlarged liver mass is corrected by apoptosis. Hepatocytes have a great replicative capacity and are capable of repopulating the liver. However, “stem-like” cells proliferate when hepatocyte replication is blocked or delayed [1–3].
Detailed studies of the mechanisms that regulate liver growth have been done in animals subjected to partial hepatectomy or chemical injury. Substantial progress have been achieved by (1) using appropriate transgenic and knockout mouse models and (2) studying liver vascularization and experimental changes such as those induced by portal vein embolization, portal vein ligation [6–8], or, more recently, by the use of the ALPPS procedure [12, 13].
The liver receives its dual blood supply from the portal vein and the hepatic artery. The normal liver receives approximately 80 % of its blood from the portal vein and 20 % from the hepatic artery. Blood flow from the two vessels follows a different pattern intrahepatically but drains into a terminal hepatic venule as the common drainage vessel [6].
A decrease in portal venous flow is compensated for by an increase in hepatic arterial flow. Although the underlying physiopathology is still unclear, adenosine, a vasodilator agent, has been suggested as a contributing factor. In normal portal venous flow, adenosine accumulates in the space of Mall but is constantly being washed away by the portal venous flow [9, 10].
When the portal blood flow is severely reduced, such as following portal vein embolization (PVE), less adenosine is washed into the portal blood and adenosine accumulates. Induced adenosine concentrations cause dilatation of the hepatic artery and increase hepatic arterial flow [6, 9, 10].
A dramatic change in hepatic blood flow, in particular from the portal vein, induces a rapid change in liver volume. This feature is observed either after PVE or partial hepatectomy (PH). Because hepatic arterial flow is preserved in the non-embolized lobe, hepatic hemodynamics following PVE slightly differ from those following PH. Hepatic arterial flow in the non-embolized lobe increases immediately secondary to intrinsic blood flow regulation by the liver, the so-called hepatic arterial buffer response (HABR) [6, 9, 10]. This may preserve hepatic viability, and marked atrophy of the embolized lobe occurs due to a reduction in total blood flow. On the contrary, portal venous flow to the non-embolized lobe increases because venous return from the splanchnic system is unchanged. The overall effect is to induce marked hypertrophy of the non-embolized lobe [6, 9, 10].
Experimental studies using rats have shown that after complete ligation of the left portal vein, left hepatic arterial flow increases by 210 %, whereas right hepatic arterial flow decreases by 67 %. Overall, total left hepatic flow decreases by 45 %, whereas total right hepatic flow increases by 230 % [11]. This finding suggests that attenuation of the increased hepatic arterial flow to the embolized lobe further decreases blood flow and enhances the effect of PVE. Nagino et al. reported the effect of dual embolization (PVE followed by arterial embolization) to enhance hepatic regeneration when insufficient hypertrophy was achieved by PVE alone. This procedure is a veritable “in situ partial hepatectomy” and carries the risk of massive necrosis in the embolized lobe [8].
Recently developed ALPPS (associating liver partition and portal vein ligature for staged hepatectomy) induces faster hypertrophy of the future liver remnant (FLR) compared to both the conventional two-stage hepatectomy and portal vein ligature or embolization [12, 13].
In addition to hemodynamic changes, upregulation of various humoral mediators and subsequent activation of intrahepatocyte signal transduction occur in the process of liver regeneration.
In brief, stimulation by PH or PVE increases circulating interleukin-6 (IL6) and tumor necrosis factor alpha (TNF-alpha). These pro-inflammatory cytokines prepare hepatocyte for growth [2, 14–18].
Further stimulation by various growth factors, such as hepatocyte growth factor (HGF), transforming growth factor alpha (TGF-alpha), and epidermal growth factor (EGF), stimulates DNA synthesis through the activation of transcriptional factors (nuclear factor kappa B (NFkB), activator protein-1 (AP-1), CCAAT enhancer-binding protein beta (C/EBP-beta)) and signal transducers and activators of transcription 3 (STAT3) [2, 6, 14–18].
Gene expression in the regenerating liver can be divided into several phases, starting with the expression of a large number of immediate early genes [2, 14–18].
The first phase of gene expression after PH, referred as “the immediate early phase,” occurs very rapidly after the operation and lasts for approximately 4 h. The proto-oncogenes c-fos, c-jun, and c-myc were the first genes to be identified in this group. Subsequently, it was shown that as many as 70 genes participate in the immediate early response to PH. These genes do not share a common function and include, among others, transcription factors, tyrosine phosphatases, as well as secreted and intracellular metabolic proteins. Surprisingly, although the mitogen-activated protein (MAP) kinases ERK1 and 2 increase in activity after PH, their elevation is relatively modest compared to that of JNK. JNK is likely to play an important role in liver regeneration through the phosphorylation of c-jun, a gene that is required for hepatic development. JNK and p38 activation after partial hepatectomy may be components of a “stress” response, but it is not known to what extent such response is required for hepatocyte replication.
After PH there is activation of transcription factors. They are proteins which bind specific recognition sites in genes to initiate or enhance their transactivation. A transcription factor can bind and activate multiple target genes. Conversely, single genes bind multiple transcription factors. Thus, transcription factors can propagate signals by activating many different genes.
After PH the following transcription factors, NFkB, AP-1 C/EBP-beta, and STAT 3, have been found activated. In particular, NFkB is a transcription factor that transactivates genes involved in (1) inflammation, (2) cell adhesion, (3) proliferation, and (4) apoptosis.
TNF through the production of reactive oxygen species (ROS) at the mitochondrial level is a potent activator of NFkB in the liver. The critical step of NFkB activation is the removal of the inhibitor IkB from the NFkB heterodimer formed by the p65 and p 50 subunits. The IKK complex phosphorylates IkB and initiates its degradation. NFkB heterodimer, free of the inhibitor, migrates to the nucleus and binds to genes containing the NFkB recognition sequences.
The second phase of gene expression after PH is referred to as “delayed early gene response.”
Bcl-Xl is an interesting example of these genes. It is the main antiapoptotic gene expressed in the liver (bcl2 is expressed only in biliary cells), and its mRNA increases after PA, reaching a maximum at 8 h after PH. One possibility is that Bcl-X1 functions as an antioxidant to prevent damage from reactive oxygen species (ROS) generated by mitochondria [1, 2]. Cell cycle genes are activated between 8 and 20 h after PH. They include p53, mdm2, p21, cyclins, and cyclin-dependent kinases. The induction of cyclin D1 is the most reliable marker for cell cycle (G1 phase) progression in hepatocytes. Overexpression of cyclin D1 in primary hepatocytes causes DNA replication even in the absence of growth factors. Therefore, hepatocytes that express CD1/cdk4 have passed the G1 restriction point and enter the G1/S transition [1, 2].
However, in liver regeneration after PH, not only stimulators but also repressors are activated. Examples of cell cycle repressor activation after PH include TGF-beta, activin, p21, and p53 [6, 18–20]. TGF-beta 1 is a multifunctional cytokine that works as both proponent and inhibitor of cell proliferation depending on the cell type, differentiation status, and milieu in which the cell works [20]. It is considered as one of the most potent growth inhibitors for hepatocytes in vitro but exerts a pivotal role during liver regeneration [2, 20]. The liver is housed by different categories of cells of hepatic, biliary, and mesenchymal origin. During liver regeneration, all of these cells restore the liver volume through a concerted action in which TGF-beta 1 seems to produce the platform for the newly divided hepatocytes by cementing them to the reconstructed extracellular matrix, thus inhibiting further growth of the hepatocytes beyond necessity. In summary, it seems that TGF-beta 1 is a negative regulator of hepatocytes proliferation and in this way restricts the volume of the liver [20].
Interestingly, after extreme hepatectomy (such as that removing up to 90 % of liver parenchyma, using the ALPPS technique), even if an adequate liver volume can be achieved in short time, in particular in old subjects with a liver remnant also altered by previous chemotherapy, patients show variable features of nodular regenerative hyperplasia [21, 22] (NRH)-like alterations, with cell plates that are markedly thickened, up to 4–5 cell thick, without concomitant vascular regeneration and collagen deposition, responsible for impaired liver function. Therefore, the normal architecture of the liver has less or no chance to be reproduced in the regenerative effort, thus impairing patient recovery during the delicate early phases of liver regeneration. It can be hypothesized that in this case, an imbalance in the proper function of TGF-beta 1 could play a role [20].
In addition to TGF-beta, glucagons and glucocorticoid also play a role as inhibitory factors in liver regeneration. On the contrary, eicosanoids, nitric oxide, prostaglandin E2 (PGE2), insulin, and estrogen have all shown to facilitate hepatic regeneration [6].
Anyway, it is the dual activation of stimulators and repressors that makes liver regeneration a precisely regulated process that never goes beyond its predicted end point. In other words, it is the equilibrium between stimulators and inhibitors expressed after PH that may explain why liver regeneration is a tightly regulated growth process [2]. However, hepatocytes need to be “primed” before they can fully respond to growth factors such as HGF, TGF-alpha, and EGF [2, 16]. Priming requires the cytokines TNF and IL-6 in addition to other agents that prevent cytotoxicity. ROS and glutathione content can determine whether the TNF effect on hepatocytes is proliferative or apoptotic.
According to Fausto [2], at least four transcription factors, NFkB, STAT3 (which are strongly induced by TNF), AP-1, and C/EBP-beta, play major roles in the initiation of liver regeneration. In addition, extensive remodeling of the hepatic extracellular matrix occurs shortly after PH. Progression through the cell cycle beyond the initiation phase requires growth factors. The expression of cyclin D1 probably establishes the stage at which replication becomes growth factor independent and autonomous [2].
Several conditions are known to suppress hepatic regeneration and cause clinical problems. Biliary obstruction, diabetes, chronic ethanol consumption, nutritional status, male gender, and infections all limit liver regeneration. Controlling these factors, whenever possible, is crucial to optimize liver hypertrophy [6].
Biliary obstruction decreases regenerative capacity both in PVE and PH. High total bilirubin concentration at the time of PVE reduces the extent of hypertrophy in the non-embolized lobe. Proposed mechanisms include: (1) restricted portal venous flow, (2) altered expression of regeneration-associated factors, and (3) impaired enterohepatic circulation. Biliary obstruction rapidly decreases portal venous flow. Portosystemic shunting is associated with acute cholestasis and may also decrease the portal venous flow velocity. Furthermore, chronic biliary obstruction induces hepatic fibrosis and increase total perfusion resistance in the portal venous system. Hepatic fibrosis is a crucial mechanism, occurring in the liver after chronic viral infection; exposure to drugs, toxicant, and xenobiotics; and chronic metabolic alterations, also including steatosis, nonalcoholic fatty liver disease (NAFLD), and steatohepatitis (NASH) associated with obesity and diabetes, and it plays a key role in most of pathophysiologic alterations leading to chronic liver disease, but also to benign and malignant liver tumors.
In addition to hemodynamic changes, cholestasis modulates molecular events, with suppression of c-myc and C/EBP-alpha and C/EBP-beta and altered production of HGF, EGF, and IL-6, so reducing the regenerative capacity. Biliary obstruction also impairs enterohepatic circulation. Lack of bile in the intestine not only has a negative effect on the enteric immune system, but also negatively affects the regenerative capacity [23–25]. Interestingly, external bile drainage for obstructive jaundice markedly suppresses liver regeneration after PH. Therefore, internal biliary drainage is preferable to external drainage in patients with biliary obstruction scheduled for PVE [23, 24].
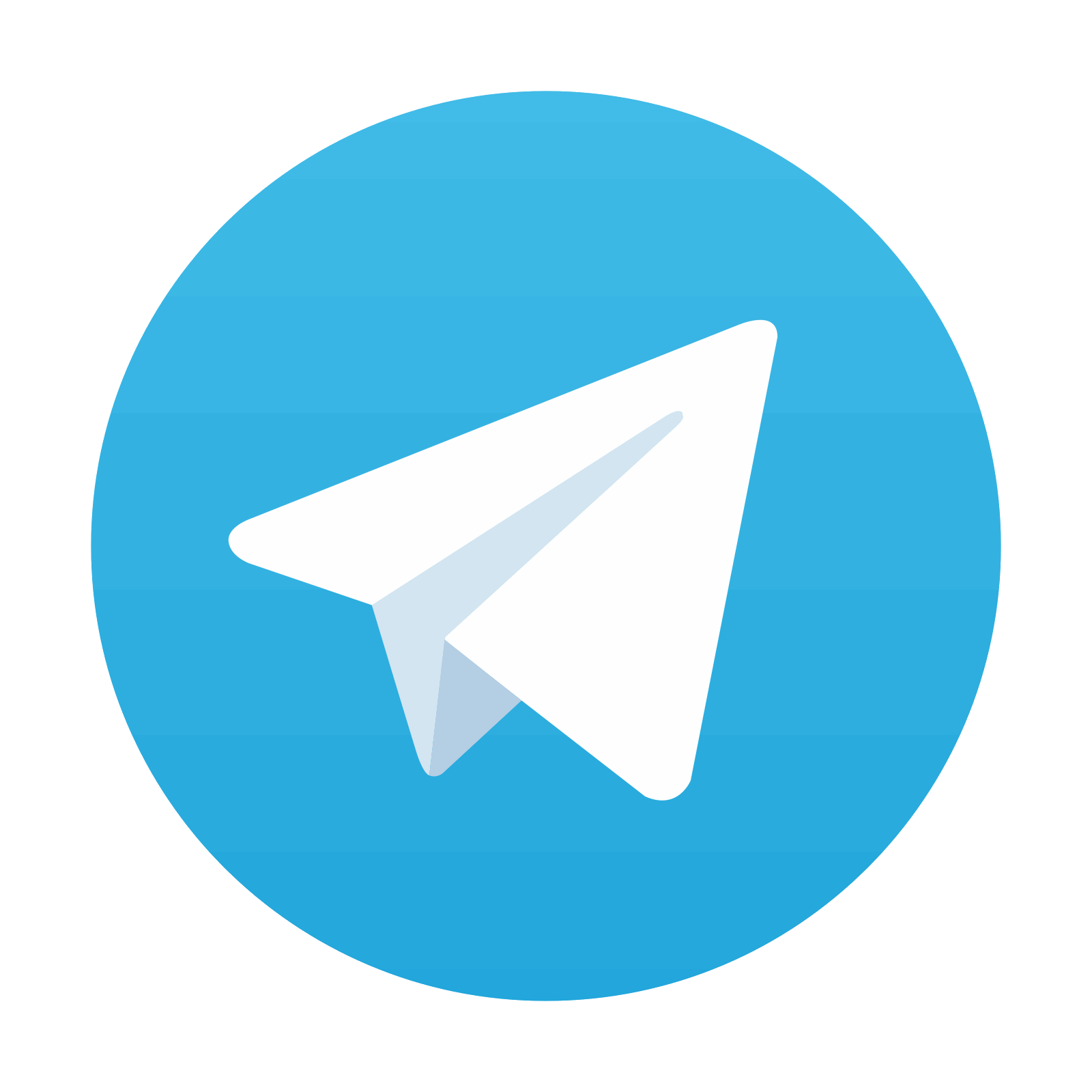
Stay updated, free articles. Join our Telegram channel

Full access? Get Clinical Tree
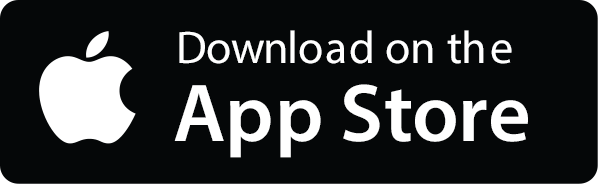
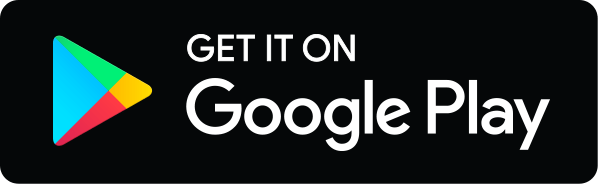