© Springer-Verlag London 2015
Vinod H. Nargund, Derek Raghavan and Howard M. Sandler (eds.)Urological Oncology10.1007/978-0-85729-482-1_22. Experimental Urological Oncology: Cellular, Molecular, and Animal
(1)
Institue of Cancer Science, University of Glasgow, Glasgow, Strathclyde, UK
(2)
Department of Urology, Beatson Institute for Cancer Research, Institute for Cancer Sciences, University of Glasgow, Glasgow, Strathclyde, UK
Keywords
Urological oncologyPre-clinical modelsCell linesXenograftsExperimental Models in Cancer
Cellular In Vitro Models
In vitro cell culture allows cultivation of human- and animal-origin cells for prolonged periods of time. Cancer cell lines, harvested ex vivo from primary or metastatic sites, maintain some of the phenotypic characteristics of the tumour of origin. Cells can be immortalized inadvertently by exposure to environmental carcinogens or by repeated passage, transfection with viral or human oncogenes, and chemical or radiation exposure. Immortalized cancer cell lines constitute an easily-accessible and -usable bank of in vitro models to investigate cancer cell biology and explore the potential efficacy of anti-cancer agents. Establishing cell lines from early or premalignant disease, such as direct primary cell culture, is technically demanding as these cells have a limited lifespan. Immortalised cell lines are vulnerable to significant inter-species and inter-cell-type contamination over time. Therefore, prior knowledge of their origin and genetic abnormalities is required to allow an informed choice of cell line(s) in experiments and interpretation of results.
2-D Culture: (Reviewed in [1])
Typically, cells are grown in monolayer on flat polystyrene or glass dishes in two dimensions (2-D culture) in growth medium at an appropriate temperature and gas mixture (typically, 37 °C, 5 % CO2 for mammalian cells) in an incubator. Growth medium can vary in pH, glucose concentration, nutrients, and growth factors (often derived from calf serum). Adherent cells can be genetically manipulated, treated with drugs, and observed using microscopy. A major criticism of 2-D culture is the assumption that accurate physiology is reproduced in a cell monolayer. Molecular and cellular responses differ significantly from the organ or tissue of origin due to a lack of extracellular matrix (ECM), which mediates cell-cell and cell-matrix communication in vivo.
3-D “Organotypic” Culture: (Reviewed in [2])
Three dimensional (3-D culture) is more physiological allowing cells to grow and respond to environmental cues within an “organotypic” structure resembling ECM (based on collagen, elastin, and other ECM proteins). 3-D spatial cell-cell and cell-matrix interactions emulate in vivo cell growth and response to drug therapies. Spheroid culture is the simplest 3-D culture technique and does not require an ECM scaffold. By single culture or co-culture of more than one cell line (e.g. hanging-drop, rotating culture, and concave plate methods), 3-D cell spheroids can be generated to model solid tumour growth. A number of biocompatible synthetic and naturally-derived scaffolds with specific surface chemical properties can be used to model the ECM environment, and examine in vitro cancer cell biology. Long-term maintenance of large biological cellular structures requires bioreactors, which continuously supply nutrients/oxygen and remove waste products/metabolites. Although, co-culture models facilitate the study of interactions between different cell types (e.g. epithelial-fibroblast interactions), these models lack additional cellular and humoral factors in vivo.
Animal In Vivo Models
Complex interactions between adjacent non-cancer cells (e.g stromal, endothelial and immune cells), blood vessels, and biological micro-environment (e.g. endocrine and paracrine pathways) play an important role in cancer progression. Hence, in vitro cell culture will never fully model the disease spectrum. The use of animal models has revolutionized in vivo cancer investigation and allows the evaluation of interactions between transformed cells (due to oncogenic mutations) and their non-cancer counterparts; termed cell and non-cell autonomous compartments, respectively. The laboratory mouse (Mus musculus) is one of the best cancer models, owing to its small size, propensity to breed in captivity, lifespan of ~3 years, entirely-sequenced genome, and similarities to humans in terms of genetics and physiology [3]. Tumours can be monitored in real-time by ultrasonography and in vivo imaging using tagged reporters. These models may be used for pre-clinical studies of chemopreventative, anticancer, and immunotherapeutic agents.
Xenograft Models
Tumour tissue or immortalised human cell lines from one species can be ectopically (in another tissue or organ) or orthotopically (in organ of origin) transplanted into another species (usually an immune-deficient mouse). Common ectopic sites include subcutaneous flank, mouse tail vein, and renal capsule. Although xenografts allow rapid and facile assessment of in vivo tumour dynamics, these models have several shortcomings: For example, cell lines ectopically-transplanted in immune-compromised hosts tend to grow more rapidly than human cancers, yielding inaccurate modelling of response to anti-cancer agents. Orthotopic transplantation is thought to be more physiological and enhances the chances of metastatic spread. However, ectopically- or orthotopically-transplanted tumours are not genetically heterogeneous (due to clonal expansion or over-representation of a particular cell type) and lack normal architecture or microenvironment observed with autochthonous tumours (reviewed in [3, 4]).
Environmentally-Induced Models
Cancers can be induced in animal models, typically mice, by a variety of environmental carcinogens including radiation, chemicals, and pathogens. Tumour development is highly-reproducible, so these models can be used to screen potential carcinogens and chemo-preventative agents. However, these animals exhibit variable tumour susceptibility (dependent on the genetic background/strain) and latency, and do not always develop the full range of pathological tumour subtypes.
Genetically-Engineered Models: (Reviewed in [3])
Genetically-engineered models allow the spontaneous development of cancer in an immune-competent host, which accurately mimics the molecular, pathological and phenotypic features of human disease. Cancer can be caused by an accumulation of genetic insults over time, and these models allow individual analysis of oncogenic pathways. By integrating (or crossing) different genetically-engineered models, the combinatorial effects of multiple genetic defects can be studied in vivo. These models may also be used to test novel therapies specifically targeted to molecular pathways of interest, representative of genetic changes in human disease.
Syngeneic Models
Cell lines derived from or embryonic tissue is isolated from a particular species is genetically manipulated, and subsequently transplanted ectopically or orthotopically into a genetically identical and immune-competent adult host. Advantages of this model include the genetically-identical backgrounds of graft and host, and an intact immune system required for anticancer immunotherapy experiments.
Transgenic Models
Transgenic animals, usually mice, express oncogenes or dominant-negative tumour suppressor genes (transgenes) under the control of promoter and enhancer elements that drive gene expression in the tissue of interest following random integration into the genome. Specific gene expression within cells constituting the tissue of interest subsequently drives a particular phenotype. By incorporation specific genetic elements (operons) into the transgenic system, transgene expression can be reversibly switched on and off using exogenous ligands (e.g. tetracycline/doxycycline or tamoxifen). The use of synthetic promoters/enhancers and random integration into the genome can result in incomplete penetrance and phenotypic effects that are unrepresentative of endogenous gene. Transgenic animals are typically created by the following methods:
Non-Homologous Recombination
Using pronuclear microinjection, a gene of interest is microinjected directly into the male pronucleus within an oocyte after fertilisation and integrates at random into the genome. The oocyte is transferred to pseudo-pregnant host female. Germline transmission and subsequent inbreeding of heterozygote progeny is necessary for the subsequent generations to be fully transgenic.
Homologous Recombination
The gene of interest is introduced into embryonic stem (ES) cells, and undergoes homologous recombination and random integration into the genome. ES cells expressing the transgene are selected, microinjected into a blastocyst inner cell mass and transferred to a pseudo-pregnant host female, which bear chimeric progeny. Chimeras are bred with wild type animals to yield a heterozygote (containing one transgenic allele), and inbred to generate homozygotes.
Retroviral Transduction
ES cells or early embryos are transduced with the gene of interest using retroviruses, resulting in random integration into the genome. ES cells expressing the transgene are microinjected into a blastocyst and transferred to a pseudo-pregnant host female, which bears chimeric progeny. Chimeras are bred as above to create homozygotes.
Gene-Targeted Models
Gene disruptions, replacements, or duplications are introduced into ES cells via homologous recombination between the endogenous (target) gene and exogenous (targeting) DNA, which possesses high sequence homology. ES cells are then microinjected into a blastocyst and transferred to a pseudopregnant host female, which then bear chimeric progeny. Chimeras are bred as above to establish homozygotes. Where a gene is disrupted or deleted, it is called a knockout; and where it there is new or duplicated gene, it is called a knockin. Where germline biallelic disruption causes embryonic lethality, site-specific recombinase enzymes under the control of tissue-specific promoters can be use to target an organ of interest. Further temporal control can be provided using exogenous ligands (tamoxifen) to regulate expression of Cre-recombinase.
< div class='tao-gold-member'>
Only gold members can continue reading. Log In or Register a > to continue
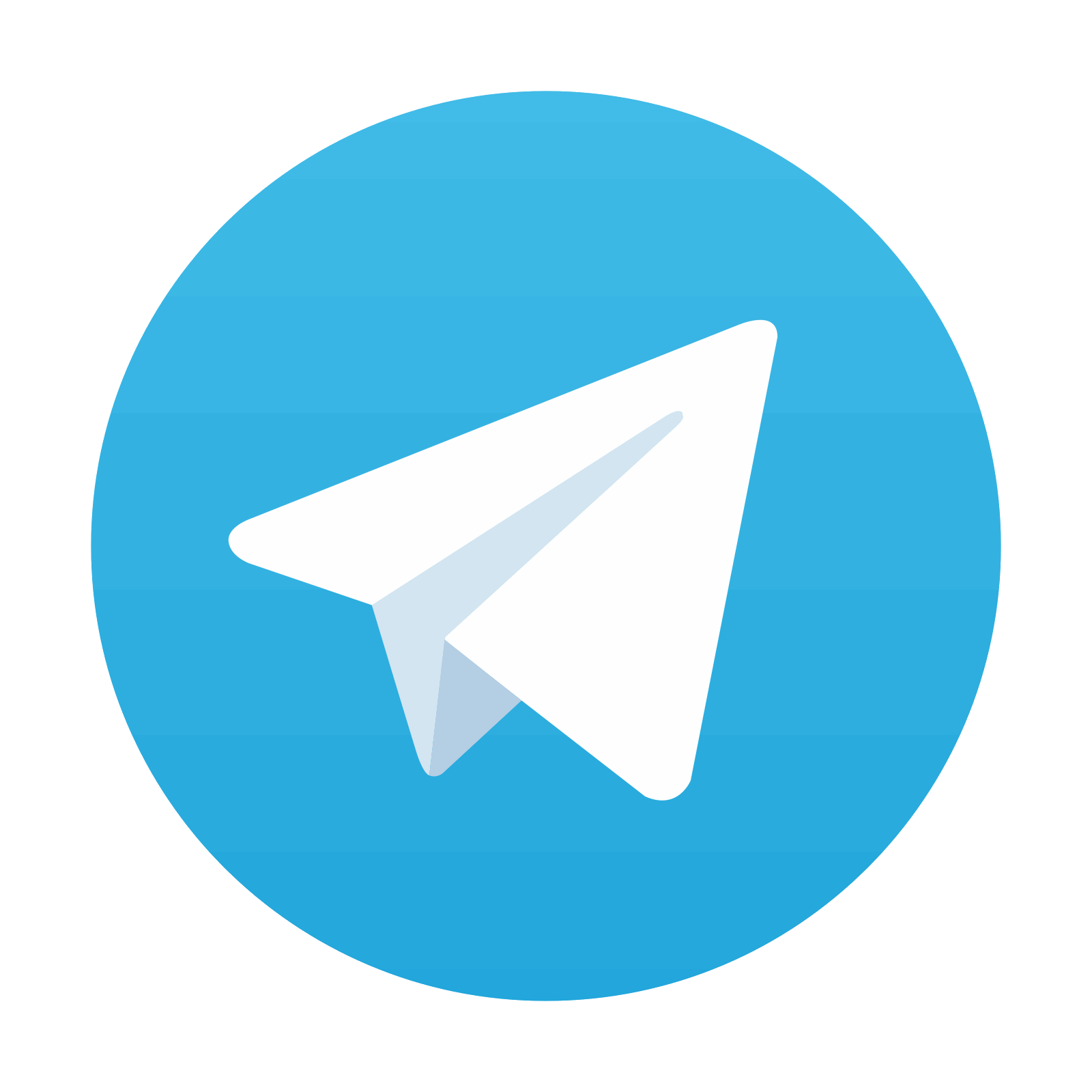
Stay updated, free articles. Join our Telegram channel

Full access? Get Clinical Tree
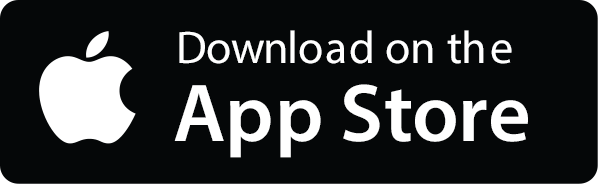
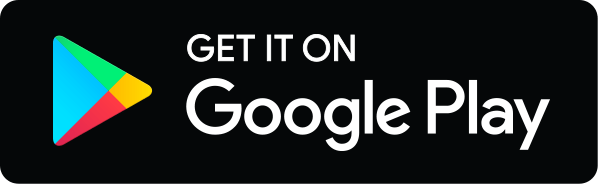