Endocrine therapy for male infertility is broadly categorized as specific or nonspecific therapy. Although uncommon, primary endocrine diagnoses in infertile men are amenable to targeted therapy. The efficacy of empiric endocrine therapy for idiopathic male infertility, however, has not been demonstrated conclusively by clinical trials. With better understanding of the underlying pathophysiology of idiopathic male infertility, careful evaluation of endocrine therapy in well-selected treatment groups and well-designed randomized, controlled trials is warranted. Although empiric endocrine therapy for idiopathic male infertility has been largely replaced by assisted reproductive techniques, both treatment modalities could play a role, perhaps as combination therapy.
Enthusiasm for hormonal therapy for male infertility has waned in recent years, especially with the advent of assisted reproductive techniques (ART). Given the important role of the hypothalamic-pituitary-gonadal (HPG) axis and its associated hormones in the growing economy of assisted reproduction with ovarian hyperstimulation, one cannot miss the irony of the marginal role that hormones play in the treatment of infertile men. Current clinical practice reflects two obvious realities. Endocrine therapy for male infertility has been disappointing despite many years of experimentation. With occasional exceptions, the promise of endocrine manipulation of male infertility never has been fulfilled. The second point is just as simple: ART delivers results. Not even its critics dispute the success rates of ART. Careful consideration must be taken before applying this technology indiscriminately for male infertility, however. ART requires treatment of the normal partner instead of the affected man, and there are other safety and cost considerations. In an ideal world, more effort would be devoted to identifying the underlying disorders in men who have idiopathic infertility and to reducing the need for so-called “empiric therapy.” Despite current clinical practice, it is important not to dismiss endocrine therapy of male infertility as a topic of merely academic interest. Although it now occupies a relatively minor position, hormonal therapy may assume a more prominent role in the future with continued identification of genetic mutations associated with infertility. Although these disorders are rare, mutations, small deletions, or polymorphic expansions of genes involved in the endocrine regulation of sexual development may impair male fertility . Point mutations and small deletions of the androgen receptor gene resulting in androgen insensitivity syndromes are good examples of genetic endocrine disorders affecting fertility . At the very least, endocrine therapy may serve as an adjunct aiding in the sperm retrieval process and thus allowing successful ART.
The most important distinction for endocrine therapy for male infertility is between specific and nonspecific treatment. Men who have specific diagnoses such as hypogonadotropic hypogonadism (HH) are treated appropriately and successfully with targeted therapy. About 1.7% of infertile men have clinically significant endocrine diagnoses . These men are an important subset of fertility patients because specific medical therapies are available for their conditions, and there is very little dispute about the role of diagnosis-specific treatment. Given this small proportion of men who have specific diagnoses, one study found no prognostic value of routine examination of the male partner of infertile couples . This approach is problematic because of the lost opportunity to apply diagnosis-specific therapy and because serious medical illnesses beyond infertility could remain undiagnosed. In one study, significant medical pathology was detected in 6% of men presenting for an infertility evaluation . Endocrine disorders such as diabetes mellitus, genetic abnormalities such as cystic fibrosis mutations, and testicular tumors were among the medical problems detected.
The empiric use of hormones for idiopathic male infertility is an entirely different matter, and it is this application that has earned hormonal therapy its dubious reputation. When an infertile couple presents with a male component, as indicated by abnormal semen analyses, and there are no known male diagnoses such as obstruction and varicoceles, clinicians have used endocrine agents, usually with little or no success. Idiopathic male infertility, the presumed target of endocrine therapy, is complex and can have various causes. In this article, the label “idiopathic infertility” is applied to men who have abnormal semen parameters without an identifiable cause based on history, physical examination, and currently available laboratory studies and radiographic examinations. Nieschlag evaluated a cohort of 10,469 consecutive infertile men. Thirty-one percent were diagnosed as having idiopathic infertility, the most common diagnosis among infertile men. This series underscores the limitations in the understanding of the pathophysiology of male infertility, despite remarkable technological advances in the field.
Clinical studies have referred to “idiopathic infertility” and “subfertility.” These terms have been determined based on semen analysis parameters . This point highlights another potential pitfall in the discussion of hormonal therapy for idiopathic male infertility. The lack of standardization of terminology, study design, and methodology makes it difficult to accumulate sufficient data for evidence-based assessment of efficacy. Without a standardized, well-defined diagnosis, it is difficult to select appropriate study assays and outcome measures. Difficulty in defining this heterogeneous group of disorders probably contributes to the often equivocal and disappointing results in many of the clinical trials. Both specific and nonspecific endocrine therapies of male infertility are discussed in this article.
The hypothalamic-pituitary-gonadal axis
Reproductive hormones are under the control of the hypothalamus. Gonadotropin-releasing hormone (GnRH) is released in a pulsatile fashion, on average every 90 to 120 minutes, from the preoptic and arcuate nuclei of the hypothalamus. GnRH enters the portal hypophyseal venous system to stimulate the anterior pituitary gland, which releases luteinizing hormone (LH) and follicle-stimulating hormone (FSH). LH stimulates the production of testosterone by Leydig cells, and FSH supports spermatogenesis in Sertoli cells. Androgens and estrogens inhibit LH release. Sertoli cells produce inhibin that decreases FSH release . Disruption at any point along the HPG axis may result in fertility problems. Patients who have these disorders can undergo targeted (specific) endocrine therapy.
Specific endocrine therapy
Targeted endocrine therapy may be applied to patients who have altered levels of GnRH, gonadotropins, androgens, and associated hormones such as prolactin, growth hormone, and thyroid-stimulating hormone. Systemic illnesses, medications, and environmental factors such as chemotherapy can impair fertility by disrupting the HPG axis; specific endocrine therapies potentially can modulate the effects of these disorders on fertility . Specific disorders can be congenital or acquired and can be categorized by their defect along the HPG axis.
Gonadotropin-releasing hormone release and receptor defects
Whether the lesion is centered in the hypothalamus or in the pituitary, one main subset of male infertility associated with endocrine factors is defective action of GnRH. This category can be represented broadly by HH, perhaps the best example of a family of disorders amenable to specific endocrine therapy for male infertility. HH may be acquired, congenital, or idiopathic. One cause of HH is GnRH receptor 1 mutations in the pituitary, but the best-characterized inherited form is Kallmann’s syndrome, which is HH associated with midline defects. This genetic disorder may be transmitted in an X-linked ( KAL gene) autosomal dominant or autosomal recessive pattern . Kallmann’s syndrome is a failure of GnRH secretion secondary to failure of the olfactory axons to establish connections with the developing olfactory bulb, hence the association with anosmia. GnRH neurons are dependent on proper olfactory nerve migration for their own travel to the forebrain . These patients usually present with delayed pubertal development. Androgen therapy initiates and sustains virilization in these patients, but additional gonadotropin therapy is required for spermatogenesis.
Several treatment regimens are available for patients who have HH and who desire fertility. Human chorionic gonadotropin (hCG) can be administered intramuscularly or subcutaneously two to three times per week, at doses ranging from 1000 to 2500 IU, in combination with human menopausal gonadotropin (hMG), 75 to 150 IU three times a week. If the patient has received only androgens in the past, hCG monotherapy is given until normal serum testosterone levels are achieved. Months of hCG administration may be required before the addition of hMG. Spermatogenesis is observed in more than 90% of patients on this regimen . Alternative regimens include the use of intermittent injections or pulsatile infusion of GnRH (in men who have intact pituitary function) and highly purified FSH or recombinant FSH (rFSH). Those who do not respond to one regimen may benefit from another. Currently available gonadotropin preparations include hMG, urinary FSH (uFSH), highly purified urinary FSH (uFSH-HP), and rFSH . rFSH is derived from genetically modified cells, whereas as the other gonadotropins are prepared from urine from postmenopausal women. Unlike the others, hMG contains both FSH and LH. HMG and uFSH are less than 5% pure, whereas uFSH-HP and rFSH achieve greater than 95% and 99% purity, respectively . The purity of urine-derived hormones is compromised primarily by undefined urinary protein contaminants. Given the range in cost of these preparations, several studies have evaluated relative efficacies of the available gonadotropins, predominantly in women undergoing ovarian hyperstimulation. One main comparison group is hMG versus FSH, because hMG contains both FSH and LH. In women, the precise role of LH in follicular growth and maturation is uncertain . In one meta-analysis a significantly higher clinical pregnancy rate was achieved with the use of FSH for ovarian stimulation during in vitro fertilization cycles . A subsequent meta-analysis detected no difference between FSH and hMG, but the validity of this study has been questioned because of problems with methodology . In a Cochrane review comparing hMG and rFSH for ovarian stimulation in ART cycles, Van Wely and colleagues found insufficient evidence of a difference between the two gonadotropins on ongoing pregnancy or live birth. The relative efficacy of commercially available gonadotropins has not been studied as extensively in men, but, as in women, a clear advantage of one gonadotropin over another has yet to be determined.
Gonadotropin release and gonadotropin receptor defects
Loss-of-function mutations can occur in genes encoding for gonadotropin and gonadotropin receptors. Hypogonadism can result from defects in the beta subunit of LH and the LH receptor . LH receptor gene mutations can result in Leydig cell hypoplasia and undermasculinization in men . Defects in the beta subunit of FSH and the FSH receptor also have been reported in the literature. The patient who had the defect in the beta subunit of FSH in the 1998 report by Phillip and colleagues presented with delayed puberty and had low serum testosterone and FSH but high LH concentrations. The diagnosis was made by DNA analysis. Treatment of these patients varies depending on the severity of hypogonadism, which can range widely. Therefore treatment regimens for these uncommon disorders are not standardized and should be tailored to individual clinical presentation. Unfortunately, receptor defects often are untreatable.
Androgen release and androgen receptor defects
Because androgens inhibit gonadotropins via the feedback loop of the HPG axis, exogenous androgen excess can result in hypogonadism and fertility problems. Exogenous androgen excess from anabolic steroid use impairs spermatogenesis by suppressing FSH levels and by depressing intratesticular testosterone . The seminiferous tubules are exposed to testosterone concentrations 20 to 100 times higher than serum levels, because androgens normally are produced locally by the Leydig cells . Testosterone supports spermatogenesis and maintains the tubular microenvironment . Androgen excess also can result from endogenous sources. The most common cause is congenital adrenal hyperplasia, which results from defects in cortisone synthesis. Low levels of cortisone cause increased release of adrenocorticotropic hormone, which in turn stimulates adrenal androgen production. Men who have congenital adrenal hyperplasia may or may not have fertility problems . Adrenal or testicular tumors also can produce excess endogenous androgens. Defects in androgen production, in the conversion of testosterone to dihydrotestosterone (5-alpha reductase deficiency), and in the androgen receptor also can cause infertility.
Numerous mutations of the androgen receptor gene, collectively described as “androgen insensitivity syndromes,” can disrupt normal virilization and potentially impact fertility, . A relatively rare condition, androgen insensitivity syndromes affect about one in 20,000 to 64,000 live male births . More than 300 mutations of the androgen receptor gene have been documented so far . The phenotype of affected individuals depends on the severity of the mutation. Complete androgen insensitivity syndrome (testicular feminizing syndrome) results in the complete feminization of genetically male individuals . Less severe mutations that cause partial androgen insensitivity can present with various degrees of ambiguous genitalia, including partial labial-scrotal fusion, hypospadias, bifid scrotum, and gynecomastia . Mild androgen receptor gene mutations with minimal androgen insensitivity can manifest with impaired spermatogenesis without associated deficiencies of secondary male sexual development . Although the phenotypic presentation varies with the severity of the gene defect, most individuals who have androgen insensitivity have similar hormone profiles . Serum testosterone and LH concentrations are at or above the normal male range during the first 3 months of life and normalize during the prepubertal period . In adult patients who have in situ testes, LH concentrations usually are elevated because of the reduced sensitivity of the hypothalamus and pituitary to feedback inhibition by sex steroids; testosterone and FSH concentrations are normal or elevated . In partial androgen insensitivity an hCG test is used to confirm normal testosterone and dihydrotestosterone production, which distinguishes this diagnosis from defects in testosterone biosynthesis and 5 alpha-reductase deficiency .
Other defects
Disturbances in levels of other hormones such as estrogens, prolactin, thyroid hormone, and glucocorticoids are other endocrinologic causes of infertility that potentially are amenable to directed therapy. Diabetes mellitus is a systemic endocrinopathy that can impair fertility at many levels, including spermatogenesis and ejaculatory and erectile dysfunction .
Specific endocrine therapy
Targeted endocrine therapy may be applied to patients who have altered levels of GnRH, gonadotropins, androgens, and associated hormones such as prolactin, growth hormone, and thyroid-stimulating hormone. Systemic illnesses, medications, and environmental factors such as chemotherapy can impair fertility by disrupting the HPG axis; specific endocrine therapies potentially can modulate the effects of these disorders on fertility . Specific disorders can be congenital or acquired and can be categorized by their defect along the HPG axis.
Gonadotropin-releasing hormone release and receptor defects
Whether the lesion is centered in the hypothalamus or in the pituitary, one main subset of male infertility associated with endocrine factors is defective action of GnRH. This category can be represented broadly by HH, perhaps the best example of a family of disorders amenable to specific endocrine therapy for male infertility. HH may be acquired, congenital, or idiopathic. One cause of HH is GnRH receptor 1 mutations in the pituitary, but the best-characterized inherited form is Kallmann’s syndrome, which is HH associated with midline defects. This genetic disorder may be transmitted in an X-linked ( KAL gene) autosomal dominant or autosomal recessive pattern . Kallmann’s syndrome is a failure of GnRH secretion secondary to failure of the olfactory axons to establish connections with the developing olfactory bulb, hence the association with anosmia. GnRH neurons are dependent on proper olfactory nerve migration for their own travel to the forebrain . These patients usually present with delayed pubertal development. Androgen therapy initiates and sustains virilization in these patients, but additional gonadotropin therapy is required for spermatogenesis.
Several treatment regimens are available for patients who have HH and who desire fertility. Human chorionic gonadotropin (hCG) can be administered intramuscularly or subcutaneously two to three times per week, at doses ranging from 1000 to 2500 IU, in combination with human menopausal gonadotropin (hMG), 75 to 150 IU three times a week. If the patient has received only androgens in the past, hCG monotherapy is given until normal serum testosterone levels are achieved. Months of hCG administration may be required before the addition of hMG. Spermatogenesis is observed in more than 90% of patients on this regimen . Alternative regimens include the use of intermittent injections or pulsatile infusion of GnRH (in men who have intact pituitary function) and highly purified FSH or recombinant FSH (rFSH). Those who do not respond to one regimen may benefit from another. Currently available gonadotropin preparations include hMG, urinary FSH (uFSH), highly purified urinary FSH (uFSH-HP), and rFSH . rFSH is derived from genetically modified cells, whereas as the other gonadotropins are prepared from urine from postmenopausal women. Unlike the others, hMG contains both FSH and LH. HMG and uFSH are less than 5% pure, whereas uFSH-HP and rFSH achieve greater than 95% and 99% purity, respectively . The purity of urine-derived hormones is compromised primarily by undefined urinary protein contaminants. Given the range in cost of these preparations, several studies have evaluated relative efficacies of the available gonadotropins, predominantly in women undergoing ovarian hyperstimulation. One main comparison group is hMG versus FSH, because hMG contains both FSH and LH. In women, the precise role of LH in follicular growth and maturation is uncertain . In one meta-analysis a significantly higher clinical pregnancy rate was achieved with the use of FSH for ovarian stimulation during in vitro fertilization cycles . A subsequent meta-analysis detected no difference between FSH and hMG, but the validity of this study has been questioned because of problems with methodology . In a Cochrane review comparing hMG and rFSH for ovarian stimulation in ART cycles, Van Wely and colleagues found insufficient evidence of a difference between the two gonadotropins on ongoing pregnancy or live birth. The relative efficacy of commercially available gonadotropins has not been studied as extensively in men, but, as in women, a clear advantage of one gonadotropin over another has yet to be determined.
Gonadotropin release and gonadotropin receptor defects
Loss-of-function mutations can occur in genes encoding for gonadotropin and gonadotropin receptors. Hypogonadism can result from defects in the beta subunit of LH and the LH receptor . LH receptor gene mutations can result in Leydig cell hypoplasia and undermasculinization in men . Defects in the beta subunit of FSH and the FSH receptor also have been reported in the literature. The patient who had the defect in the beta subunit of FSH in the 1998 report by Phillip and colleagues presented with delayed puberty and had low serum testosterone and FSH but high LH concentrations. The diagnosis was made by DNA analysis. Treatment of these patients varies depending on the severity of hypogonadism, which can range widely. Therefore treatment regimens for these uncommon disorders are not standardized and should be tailored to individual clinical presentation. Unfortunately, receptor defects often are untreatable.
Androgen release and androgen receptor defects
Because androgens inhibit gonadotropins via the feedback loop of the HPG axis, exogenous androgen excess can result in hypogonadism and fertility problems. Exogenous androgen excess from anabolic steroid use impairs spermatogenesis by suppressing FSH levels and by depressing intratesticular testosterone . The seminiferous tubules are exposed to testosterone concentrations 20 to 100 times higher than serum levels, because androgens normally are produced locally by the Leydig cells . Testosterone supports spermatogenesis and maintains the tubular microenvironment . Androgen excess also can result from endogenous sources. The most common cause is congenital adrenal hyperplasia, which results from defects in cortisone synthesis. Low levels of cortisone cause increased release of adrenocorticotropic hormone, which in turn stimulates adrenal androgen production. Men who have congenital adrenal hyperplasia may or may not have fertility problems . Adrenal or testicular tumors also can produce excess endogenous androgens. Defects in androgen production, in the conversion of testosterone to dihydrotestosterone (5-alpha reductase deficiency), and in the androgen receptor also can cause infertility.
Numerous mutations of the androgen receptor gene, collectively described as “androgen insensitivity syndromes,” can disrupt normal virilization and potentially impact fertility, . A relatively rare condition, androgen insensitivity syndromes affect about one in 20,000 to 64,000 live male births . More than 300 mutations of the androgen receptor gene have been documented so far . The phenotype of affected individuals depends on the severity of the mutation. Complete androgen insensitivity syndrome (testicular feminizing syndrome) results in the complete feminization of genetically male individuals . Less severe mutations that cause partial androgen insensitivity can present with various degrees of ambiguous genitalia, including partial labial-scrotal fusion, hypospadias, bifid scrotum, and gynecomastia . Mild androgen receptor gene mutations with minimal androgen insensitivity can manifest with impaired spermatogenesis without associated deficiencies of secondary male sexual development . Although the phenotypic presentation varies with the severity of the gene defect, most individuals who have androgen insensitivity have similar hormone profiles . Serum testosterone and LH concentrations are at or above the normal male range during the first 3 months of life and normalize during the prepubertal period . In adult patients who have in situ testes, LH concentrations usually are elevated because of the reduced sensitivity of the hypothalamus and pituitary to feedback inhibition by sex steroids; testosterone and FSH concentrations are normal or elevated . In partial androgen insensitivity an hCG test is used to confirm normal testosterone and dihydrotestosterone production, which distinguishes this diagnosis from defects in testosterone biosynthesis and 5 alpha-reductase deficiency .
Other defects
Disturbances in levels of other hormones such as estrogens, prolactin, thyroid hormone, and glucocorticoids are other endocrinologic causes of infertility that potentially are amenable to directed therapy. Diabetes mellitus is a systemic endocrinopathy that can impair fertility at many levels, including spermatogenesis and ejaculatory and erectile dysfunction .
Idiopathic infertility
More than 30% of infertile men have no identifiable cause for their abnormal semen analyses in the setting of a completely normal work-up (with the possible exception of mild FSH elevation) . In these men who have “idiopathic” infertility, semen analyses may reveal oligo-, astheno-, or teratospermia or a combination of these conditions. Greater understanding of the factors that control spermatogenesis probably will reveal the underlying pathology of many of these disorders. Genetic mechanisms, especially autosomal disorders, may account for many cases heretofore labeled “idiopathic” . Interest has been renewed in genetic studies to determine underlying causes of idiopathic male infertility, but the overwhelming trend has been to sidestep improvements in diagnostic evaluation in favor of a more expensive, albeit efficacious option, ART. Ideally, cause-specific therapy of male-factor infertility would minimize the use of ART, avoiding the costs, complications, and treatment of the unaffected female partner.
Empiric endocrine therapy
One approach for treating male-factor infertility has been empiric medical therapy. In fact, medical treatment for idiopathic male infertility was used for many years before the introduction of ART. Unfortunately, the efficacy of empiric therapy has been underwhelming in most clinical trials. Evaluating empiric therapy for idiopathic infertility is difficult for many reasons. Who is being treated, and what is being treated? What are the inclusion and exclusion criteria? Which treatment(s) and which dosing regimen are used? What are the outcome measures? Why perform a clinical trial when antecedent studies have borne disappointing results and with the availability of ART? Perhaps because of these obstacles, many trials conducted to date have been nonrandomized and uncontrolled. In a previous review of medical management of infertility, Siddiq and Sigman point out the significant background pregnancy rate (26%) for untreated couples with abnormal semen parameters . The frequency of treatment-independent pregnancies brings into question the results of previous clinical trials that were conducted without appropriate control data.
Gonadotropin-releasing hormone agonists
One strategy of empiric therapy is to stimulate native gonadotropin secretion with GnRH agonists, which must be administered in a pulsatile manner. Very few controlled studies have been conducted. Most published trials have small sample sizes without a control arm and have varying outcome measures including gonadotropin, androgen, and estrogen levels, semen parameters, and pregnancy rate. Both GnRH analogues and synthetic luteinizing hormone–releasing hormone (LHRH) have been evaluated. The dosing regimens of hormonal agents for several trials discussed are listed in Table 1 . A few randomized, controlled trials have been completed. In 1988, Badenoch and colleagues randomly assigned 19 men who had idiopathic oligozoospermia to receive one of two different dosages of buserelin (D-Ser(TBU)6-GnRH ethylamide) for 12 weeks. No effect was noted for gonadotropin levels or sperm concentrations with therapy. Crottaz and colleagues subsequently reached a similar conclusion. Twenty-eight men who had idiopathic normogonadotropic oligoasthenozoospermia (INOA) were assigned randomly to received GnRH or placebo. GnRH treatment did not improve sperm quality. Matsumiya and colleagues noted modest but statistically significant improvement in sperm parameters with buserelin therapy for men who had INOA, but more important outcome measures such as pregnancy rate were not evaluated. Although a few studies report improved semen parameters and even successful pregnancies on therapy, most trials do not have sufficient power to demonstrate a significant benefit of these medications for idiopathic therapy. There is consensus that GnRH and LHRH agonists have no role in empiric therapy.
Trial | Year | Preparation Used | Dose | Duration |
---|---|---|---|---|
GnRH/LHRH | ||||
Schwarzstein et al | 1975 | LHRH | Mean dose 500 μg/d IM | 100–135 days |
Aparicio et al | 1976 | LHRH | 100–500 μg /d IM | Long term (60 days or more) or short term (30-day intervals) |
Schwarzstein et al | 1982 | LHRH | 5 μg IM every 2 days or | 90 days |
10 μg IM daily or | ||||
10 μg IM every 2 days | ||||
Fauser et al | 1985 | LHRH | 5 μg or 20 μg SC every 90 minutes | 3 months |
Honigl et al | 1986 | LHRH | 5 μg SC every 2 hours | 6 weeks |
Badenoch et al | 1988 | Buserelin | 1 μg or 10 μg twice weekly | 12 weeks |
Aulitzky et al | 1989 | LHRH | 4 μg SC every 120 min | 6 months |
Bals-Pratsch et al | 1989 | GnRH | 5 μg SC infused for 1 minute every 120 minutes or | 12 weeks |
5 μg SC infused for 1 minute every 90 minutes | 24 weeks | |||
Crottaz et al | 1992 | GnRH | 0.2 mg every 2 hours IN from 8 am to 8 pm | 3 months double-blind, then 1 month no treatment, then 3 months open |
Matsumiya et al | 1998 | Buserelin acetate or | 15 μg once daily IN | 3 months |
Clomiphene citrate | 50 mg once daily | 3 months | ||
Gonadotropins | ||||
Knuth et al | 1987 | hMG & hCG | 150 IM 3 times per week & 2500 IM twice weekly, respectively | 13 weeks |
Kamischke et al | 1998 | rFSH | 150 IU SC daily | 12 weeks |
Ashkenazi et al | 1999 | purified FSH | 75 IU FSH (<1 IU LH) | 50–71 days |
Baccetti et al | 2004 | uFSH-HP | 150 IU SC daily | 12 weeks |
Foresta et al | 2005 | rFSH | 100 IU IM on alternate days | 3 months |
Androgens | ||||
Aafjes et al | 1983 | Mesterolone | 75 mg daily | 6 months, then cross-over |
Wang et al | 1983 | Clomiphene citrate | 25 or 50 mg daily or | 6 months |
Mesterolone | 100 mg daily or | 6 months | ||
Testosterone enanthate | 100 or 250 mg on alternate weeks | 4 months | ||
Hargreave et al | 1984 | Mesterolone | 100 mg daily | 9 months |
Pusch | 1989 | Testosterone undecanoate | 120 mg daily | 100 days |
World Health Organization | 1989 | Mesterolone | 75 or 150 mg daily | 6 months |
Comhaire | 1990 | Testosterone undecanoate | 240 mg daily | 3-month double-blind period followed by 3 month open phase |
Gerris et al | 1991 | Mesterolone | 150 mg daily | 12 months |
Comhaire et al | 1995 | Testosterone undecanoate | 120 mg daily | 3 months |
Antiestrogens | ||||
Ronnberg | 1980 | Clomiphene citrate | 50 mg daily | 3 months (cross-over trial) |
Abel et al | 1982 | Clomiphene citrate | 50 mg daily | 6 months |
Micic and Dotlic | 1985 | Clomiphene citrate | 50 mg daily | 6–9 months |
Torok | 1985 | Tamoxifen citrate | 20 mg daily | 3 months |
AinMelk et al | 1987 | Tamoxifen citrate | 20 mg daily | 6 months |
Sokol et al | 1988 | Clomiphene citrate | 25 mg daily | 12 months |
World Health Organization | 1992 | Clomiphene citrate | 25 mg daily | 6 months |
Adamopoulos et al | 2003 | Tamoxifen citrate and testosterone undecanoate | 20 mg daily and 120 mg daily, respectively | 6 months |
Hussein et al | 2005 | Clomiphene citrate | Dose titrated to achieve serum testosterone levels between 600 & 800 ng/dL | 3–9 months |
Aromatase inhibitors | ||||
Clark and Sherins | 1989 | Testolactone | 2 g daily | 8 months, then cross-over for another 8 months |
Pavlovich et al | 2001 | Testolactone | 50–100 mg orally twice daily | 5 months mean |
Raman and Schlegel | 2002 | Testolactone or | 50–100 mg twice daily | 6 months mean |
Anastrozole | 1 mg daily | 4.7 months mean | ||
Oxytocin | ||||
Byrne et al | 2003 | Oxytocin | 0.75 IU | Two separate occasions |
Special uses | ||||
Cryptorchidism | ||||
Hadziselimovic and Herzog | 1997 | Buserelin acetate | 10 μg IN every other day | 6 months |
Huff et al | 2001 | Naferelin | 200 μg IN biweekly | 6 months |
Cancer therapy | ||||
Johnson et al | 1985 | LHRH | 50 μg daily SC | Started median 5 days before chemotherapy and continued for at least 1 week following completion of chemotherapy |
Fossa et al | 1988 | Medroxyprogesterone acetate | 500 mg daily | Started on day 1 of the first chemotherapy cycle |
Kreuser et al | 1990 | LHRH | 0.4–0.5 mg three times/d | Started 14 days before chemotherapy and continued for 15 days after completion of chemotherapy |
Masala et al | 1997 | Testosterone (blend of enanthate and propionate esters) | 100 mg IM every 15 days | Started 30 days before cyclophosphamide therapy and continued for duration of therapy |
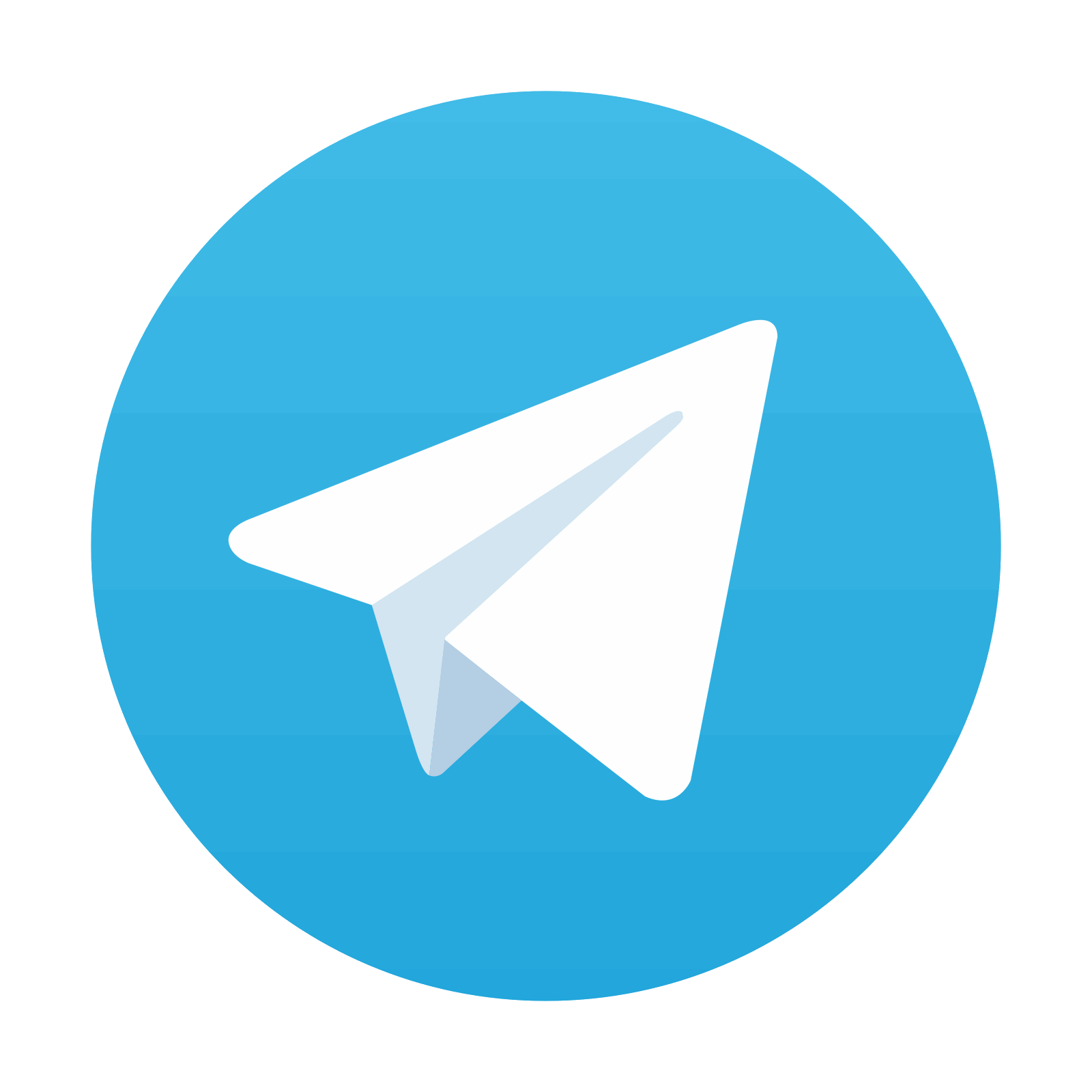
Stay updated, free articles. Join our Telegram channel

Full access? Get Clinical Tree
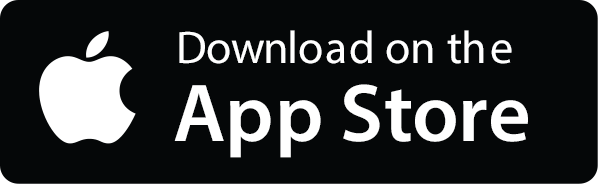
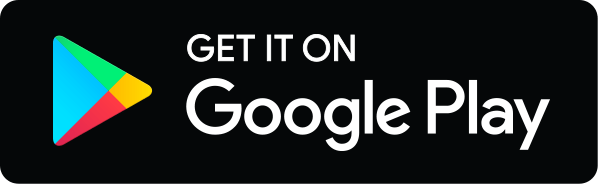
