Sandip P. Vasavada, MD, Raymond Robert Rackley, MD For the past century, the development of electrical neurostimulation and neuromodulation to alter physiologic processes responsible for lower urinary tract symptoms and dysfunctions has largely been the result of important landmark discoveries in the clinical application of electricity and in the understanding of neuromuscular physiology. In neurostimulation the use of electrical stimuli on nerves and muscles has mainly been developed to achieve immediate clinical responses in neurogenic conditions of pelvic organ dysfunction; in neuromodulation the application of electrical stimuli to nerves has been developed to alter neurotransmission processes in non-neurogenic and neurogenic conditions. In this chapter the focus is on reviewing the application (Table 70–1) and clinical outcomes of neurostimulation and neuromodulation therapies for pelvic organ dysfunction with respect to the development of our knowledge of pelvic neuromuscular physiology and its role in translational innovations. Table 70–1 Potential Applications of Electrical Stimulation in the Treatment of Voiding Dysfunction
PURPOSE | SITES OF STIMULATION | MECHANISM |
---|---|---|
Facilitate Filling-Storage | ||
Inhibit detrusor contractility Increase bladder capacity Decrease urgency and frequency | V, A, SP, PT CP, SR, IV | Neuromodulation |
Decrease nociception | V, A, SP, SR | Neuromodulation |
Increase outlet resistance | V, A, SR | Direct stimulation (efferent nerves or roots) |
Facilitate Emptying | ||
Stimulate detrusor contraction (spinal cord–injured patient) | SAR | Direct stimulation (efferent nerves or roots) |
Restore micturition reflex (idiopathic retention) | SR, IV | Neuromodulation |
A, anal; CP, common peroneal; IV, intravesical; PT, posterior tibial; SAR, sacral anterior (ventral) roots; SP, suprapubic; SR, sacral roots; V, vaginal.
History of Electrical Stimulation
Although the field of electrical stimulation of nerves to achieve muscle contractions was not truly realized until the early 1800s, its roots can be traced into the 1700s, when inadvertent electrical impulses were found to generate strong muscle convulsions (Galvani, 1786; Bell, 1811). Magendie (1822) was one of the first to conduct physiologic investigations of the spinal nerve roots, documenting in young dogs that transection of the posterior (dorsal) segments resulted in a lack of sensation but persistence of motor function, whereas anterior (ventral) root transection yielded preservation of sensation yet abolishment of motor function. These important findings created the foundation for our understanding of basic neurophysiology of micturition and led to further discoveries on bladder function in the setting of selective rhizotomy of both the pelvic and hypogastric nerves (Giannuzzi, 1863; Langley, 1895). Ultimately, Saxtorph in 1878 used these principles to directly stimulate the bladder in patients with urinary retention via a metal transurethral catheter (Madersbacher, 1999). His early findings allowed others to develop enthusiasm for direct stimulation of the bladder through both transurethral and direct detrusor routes (see section on direct stimulation).
Because Saxtorph had significant influence on the idea that direct bladder stimulation may lead to bladder contractility, McGuire used some of these same principles to perform direct bladder stimulation in dogs (Boyce et al, 1964). He concluded that multiple pairs of electrodes were required to achieve a more uniform pressure rise within the bladder during the stimulated contraction. Research continued throughout the late 1900s and moved toward development of new electrodes (Susset and Boctor, 1967) and differing wire configurations (Timm and Bradley, 1969; Tscholl et al, 1971).
Direct pelvic nerve stimulation and pelvic floor muscle stimulation were not well-known or well-studied concepts until the mid 1900s because emphasis was clearly on different areas of direct stimulation (i.e., bladder). Dees (1965) studied the bladder contraction state after stimulation to the pelvic nerve. He achieved contraction of both the detrusor muscle and urethral sphincter in a cat model and produced pain and hind leg contraction as well. Others demonstrated similar findings of nonspecific contraction of the bladder and pelvic floor and other areas with direct pelvic nerve stimulation and ultimately realized that direct pelvic nerve stimulation may not be suitable for treatment of bladder dysfunction (Burghele et al, 1962; Hald et al, 1966; Holmquist and Olin, 1968). It appears that direct pelvic nerve stimulation elicits pudendal nerve activity such that outlet resistance is increased, as is pain, through simultaneous hypogastric nerve stimulation. Thus, with the limited-potential clinical utility of the pelvic nerve for stimulation, efforts focused on the pelvic floor muscles, spinal cord, and sacral roots. Caldwell first reported experience with pelvic floor muscle stimulation with the goal of improving fecal continence and later urinary incontinence (Caldwell, 1963; Caldwell et al, 1965). Subsequently, interest increased and efforts toward external stimulation were described by anal, vaginal pessary, and direct vaginal stimulation (Hopkinson and Lightwood, 1967; Alexander and Rowan, 1968; Erlandson et al, 1977; Fall et al, 1977).
Spinal cord stimulation, by attempting to directly activate the micturition center, was thought to be a promising avenue of therapy (Nashold et al, 1971; Jonas et al, 1975; Jonas and Tanagho, 1975). Still, as in pelvic nerve stimulation, voiding was initiated; however, simultaneous sphincter activity precluded proper emptying. The subsequent series of developments pursued therapy for incomplete emptying by affecting not only bladder stimulation but, in some way, sphincter relaxation as well.
In 1972, Brindley began experimentation of sacral root stimulation that led to implantation of sacral anterior root stimulators in paraplegic patients with urinary incontinence (Brindley, 1972, 1974, 1977). Evolving data showed that, for optimal bladder emptying to be achieved, sacral anterior root stimulation with posterior rhizotomies of S2, S3, and S4 would be required (Sauerwein, 1990). The posterior rhizotomy would decrease the reflex activity of the detrusor and improve bladder compliance. Tanagho and coworkers then began further examining the sacral roots and their individual contributions to bladder and outlet function. In 1982, Tanagho and Schmidt presented initial experience in sacral root stimulation in paraplegic dogs. In this initial study they realized the design of a spiral electrode to minimize nerve damage and fixed the lead wire to the sacral lamina, thereby preventing tension on the electrode itself. They ultimately attained good bladder contraction in these dogs, with minimal sphincteric response. From these initial good results, Tanagho and coworkers then began human trials and characterized the sacral root stimulation patterns and the corresponding muscle responses (Table 70–2). In the course of the neurostimulation developments these researchers realized that sphincteric contraction abolished detrusor activity, and the role of the pudendal nerve and its modulation of bladder capacity began to evolve (Tanagho and Schmidt, 1988; Schmidt, 1989). Thus, neuromodulation was introduced as a concept by which activation of the sacral roots may, in fact, modulate external sphincter function and in turn inhibit detrusor activity as a normal reflex. These early discoveries created the platform for the current and future concepts and technologies that are used for neurostimulation and neuromodulation.
Table 70–2 Sacral Nerve Responses
SACRAL NERVE | MOTOR AND SENSORY RESPONSE |
---|---|
S2 | Motor: Plantarflexion of the entire foot with lateral rotation and clamp movement of the anal sphincter |
Sensory: Sensations in the leg and buttock | |
S3 | Motor: Dorsiflexion of the great toe and bellows reflex (anal wink) |
Sensory: Paresthesias or sensation of pulling in the rectum, scrotum, or vagina | |
S4 | Motor: Bellows reflex only |
Sensory: Sensation of pulling in the rectum only |
Neurophysiology of Electrical Stimulation for Storage and Emptying Disorders
Normal detrusor function appears to be a sacral balance under suprasacral influences of the sympathetic and parasympathetic nervous systems in their respective abilities to maintain continence. The sympathetic tone, for the most part, is dominant for the majority of time and thus provides continence or storage of urine; the parasympathetic nervous system allows detrusor contractions for emptying of the bladder. Thus, the micturition reflex pathway is activated by initial bladder afferent excitation that then results in a bladder efferent excitation leading to a detrusor muscle contraction. The acquired and unique ability to void volitionally is due to either negative feedback (inhibition of voiding) or positive feedforward (induction of voiding) influences of supraspinal inputs from the pontine micturition center on this sacral micturition reflex pathway. Any loss of either central supraspinal inhibitory influences or increased sensitization of bladder afferent signaling can lead to unmasking of involuntary voiding. As such, this has been proven only in animals and, by deference, relates then to humans. There may be primitive reflexes that reside within the spinal cord that can be “awakened” by somatic and afferent nerve stimulation and may have something to do with the mechanism of action of neuromodulation (de Groat, 1975, 1976; de Groat and Ryall, 1968a, 1968b).
Bladder afferent nerve signaling sends information about pain and bladder fullness to the brain that will in turn initiate the micturition reflex. Bladder overactivity may be in part mediated by the loss of voluntary control of the voiding reflex and, furthermore, emergence of primitive voiding reflexes. In certain states of neurologic or inflammatory disease of the bladder, the previously silent C fibers may emerge and trigger the micturition reflex. Accordingly, blockade of this pathway by electrical neuromodulation, similar to pharmacologic blockade by capsaicin (a C-fiber blocker), may suppress detrusor overactivity (Maggi and Meli, 1988; Chen et al, 1993).
Reflexes That Promote Bladder Storage
Two important reflexes may play an important role in modulation of bladder function: the guarding reflex and the bladder afferent loop reflex. Both reflexes promote urine storage (guarding reflex under somatic influence and bladder loop reflex under sympathetic tone). The guarding reflex guards or prevents urine loss from times of cough or other physical stress that would normally trigger a micturition episode. Suprapontine input from the brain turns off the guarding reflex during micturition to allow efficient and complete emptying. The bladder afferent reflex works through sacral interneurons that then activate storage through pudendal nerve efferent pathways directed toward the urethral sphincter. As such, the activity has truly been realized only in cats but has been postulated to exist in humans and to function the same. Similar to the guarding reflex, the bladder afferent reflex promotes continence during periods of bladder filling and is quiet during micturition (Fig. 70–1).
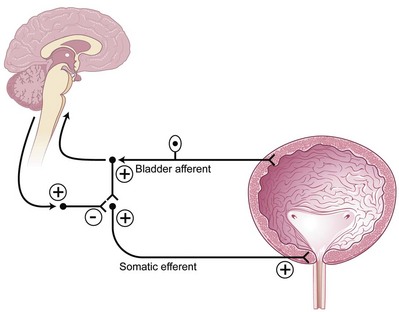
(Modified from Leng WW, Chancellor MB. How sacral nerve stimulation neuromodulation works. Urol Clin North Am 2005;32:11–8.)
Reflexes That Promote Bladder Emptying
Signals from the bladder that may modulate the need to void with fullness, pain, pressure, or stretch may elicit bladder afferent activity through the Aδ or even C fibers. These bladder afferent nerve fibers then synapse with both parasympathetic efferents (bladder-bladder reflex) and parasympathetic urethral efferents (bladder-urethral) reflex. The urge to void may then be translated as an initial activity (inhibitory) of the bladder-urethral reflex to allow the pressure in the urethral outlet to drop immediately before a bladder contraction ensues and simultaneously permit the bladder-bladder reflex to allow a smooth bladder contraction to occur as the reflex is maintained throughout the entire void (de Groat, 1978; de Groat et al, 1981, 1996).
Putative Mechanism of Action of Sacral Neuromodulation
Although our knowledge of how neuromodulation works is evolving, two main theories exist: (1) direct activation of efferent fibers to the striated urethral sphincter reflexively causes detrusor relaxation and (2) selective activation of afferent fibers causes inhibition at spinal and supraspinal levels. Accumulating evidence suggests that activation of somatic sacral afferent inflow at the sacral root level that in turn affects the storage and emptying reflexes in the bladder and central nervous system accounts for the positive effects of neuromodulation on both storage and emptying functions of the bladder (Yoshimura and de Groat, 1992, 1997; Leng and Chancellor, 2005). Malaguti and coworkers (2003), using detection of somatosensory evoked potentials during sacral neuromodulation, concluded that sacral neuromodulation therapy works by sacral afferent activity and concomitant activation of the somatosensory cortex. Because sacral neuromodulation has been clinically proven for both storage (urgency/frequency and urgency urinary incontinence) and emptying (nonobstructive urinary retention) dysfunctions of the bladder, isolating the mechanism of action to the micturition reflex pathway of sacral afferent and efferent pathways alone is challenging. However, by understanding the reflexes that influence the promotion of urine storage or emptying of the sacral micturition reflex pathway one begins to realize how neuromodulation may affect these reflexes and elicit symptomatic and functional improvement of voiding function (Fowler et al, 2000). Animal models of sacral neuromodulation for detrusor overactivity are now being developed and may shed more light on how this technology achieves its benefits (Riazimand and Mense, 2004; Vignes et al, 2009)
Putative Mechanism of Action of Sacral Neuromodulation in Overactive Bladder
The bladder storage and emptying reflexes are modulated by several centers in the brain and may be altered by neurologic injury that effectively unmasks involuntary bladder contractions. Thus, sacral neuromodulation of these primitive reflexes may restore normal micturition (de Groat, 1976). Animal data exist to support the fact that somatic afferent input to the spinal cord can affect the guarding and bladder-bladder reflexes (de Groat, 1978; Chen et al, 1993). It is believed that suppression of interneuronal transmission in the bladder reflex pathway may be how sacral neuromodulation affects detrusor overactivity (de Groat, 1976; Kruse and de Groat, 1993; Leng and Chancellor, 2005). The inhibition by electrical neuromodulation may, in part, modulate the sensory outflow from the bladder through the ascending pathways to the pontine micturition center, thereby preventing involuntary contractions by modulating the micturition reflex circuit but allowing voluntary voiding to occur (Fig. 70–2). The preservation of voluntary voiding may be due to selective avoidance of normal sensory ascending outflow pathways of the bladder from Aδ fibers to the pontine micturition center as well as initiation of the descending pathways from the pontine micturition center to sacral efferent outflow pathways. Therefore, as is seen in clinical practice, sacral neuromodulation may affect and improve the abnormal bladder sensations, involuntary voids, and detrusor contractions but still maintain normal bladder sensations and voluntary voiding patterns.
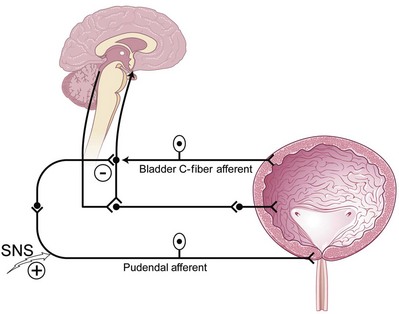
(Modified from Leng WW, Chancellor MB. How sacral nerve stimulation neuromodulation works. Urol Clin North Am 2005;32:11–8.)
Putative Mechanism of Action of Sacral Neuromodulation in Urinary Retention
Sphincteric activity can be turned off by brain pathways to allow efficient and complete bladder emptying. If the suprasacral pathways are altered, the guarding and urethral reflexes still exist and cannot be turned off. This may cause retention, as in the spinal cord–injured patient who in turn has detrusor-sphincter dyssynergia resulting in urinary retention. Thus, inhibition of the guarding reflexes may allow urinary retention states to be improved (Fig. 70–3). Originally for urinary retention due to functional outlet obstruction, sacral neuromodulation was thought to directly turn off excitatory flow to the urethral outlet and facilitate bladder emptying. This may be an efferent effect of the primary afferent mechanism of sacral neuromodulation as reported by Dasgupta and colleagues (2005), who described novel neuroimaging evidence for the existence of abnormal interaction between the brainstem and cortical centers in women with urinary retention due to Fowler syndrome or overactive sphincteric activity. The direct therapeutic effect of sacral neuromodulation in patients with sphincter overactivity or functional outlet obstruction appears to be in the afferent restoration of a normal pattern of midbrain activity and decreased cortical activity leading to inhibition of the guarding reflexes.
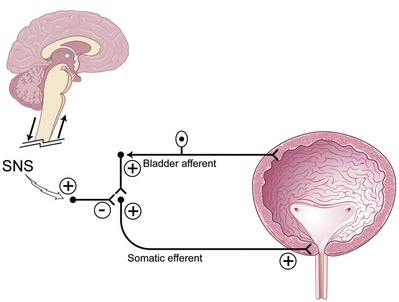
(Modified from Leng WW, Chancellor MB. How sacral nerve stimulation neuromodulation works. Urol Clin North Am 2005;32:11–8.)
Electrical Stimulation for Storage Disorders
Criteria for Selection of Patients
Because many lower urinary tract symptoms and dysfunctions are secondary to a neuromuscular etiology, a thorough history and physical examination will often reveal the nature (acute vs. chronic) and help classify the cause (neurogenic, anatomic, postsurgical, functional, inflammatory, or idiopathic). In addition to the unique evaluation of pelvic floor muscle dysfunction (Siegel, 2005), a urinalysis is routinely performed; urine cytology should be considered in patients who present with refractory symptoms of dysuria, urgency, or frequency of urination, because carcinoma in situ and bladder tumors may present as irritative bladder symptoms without hematuria. Further assessment of the bladder function, urodynamic studies including cystometrography, pressure-flow studies, and electromyography of sphincters and pelvic floor muscles are performed on a selected basis, because most non-neurogenic assessments are completed routinely with the use of a voiding diary and a focused physical examination of the pelvis. Electromyography is recommended in suspected cases of neurogenic bladder dysfunction, detrusor-sphincter dyssynergia, or Fowler syndrome and may be considered for evaluation of inappropriate pelvic floor muscle behavior (Dasgupta and Fowler, 2003). As of now, urodynamics have not yielded adequate predictive factors that allow enhanced selection for patients undergoing sacral neuromodulation (Groenendijk et al, 2007). The characteristics of neurogenic bladders, as seen in patients with multiple sclerosis and spinal cord injury, can change with time and disease progression. Therefore, reevaluation with urodynamics and assessment of the upper urinary tracts may be needed when symptoms change despite active medical intervention.
Relative contraindications for patients who may be considering or who have an implantable electrical stimulation device are magnetic resonance imaging (MRI) and pregnancy. Magnetic fields produce currents in neuroelectrodes, and there is some concern that the magnetic field from MRI may damage the pulse generator, as discussed later. Many radiologists are reluctant to provide MRI services for patients with implantable electrical stimulation devices despite the anecdotal evidence that no adverse event has occurred when MRI has inadvertently or purposefully been done for emergent reasons or in small trials (Hassouna and Elkelini, 2005). For patients who have InterStim devices in place (see later), the authors advocate removal of the device in preparation for elective MRI based on current manufacturer recommendations. After the MRI procedure, a new neuroelectrode and generator may be placed. Anecdotal reports of patients safely undergoing the study (MRI) with the InterStim implant in place have occurred, but patients should have the devices turned off in anticipation. Because of the potential for teratogenicity or abortion from the effect of electrical stimulation, electrical stimulation has been considered contraindicated in pregnant women with various voiding dysfunctions. However, whether electrical stimulation can cause abortion or malformation is not known. Wang and Hassouna (1999) reported no adverse effects of electrical stimulation on pregnant rats and that termination of pregnancy is not advised for prospective mothers when electrical stimulation has been performed unknowingly in early pregnancy. Women with electrical stimulation devices for pelvic health conditions who become pregnant may simply turn off their devices during pregnancy.
Electrical Stimulation of the Bladder
Transurethral electrical bladder stimulation (TEBS) has been pursued not only for initiating sensory awareness of bladder filling and stimulating detrusor contractility (see later section) but also for increasing bladder capacity at low pressure in pediatric patients with myelomeningocele (Kaplan et al, 1989; Decter et al, 1994). The authors in these two cited references carried on an interesting point-counterpoint discussion in publications regarding the practical benefit of TEBS (Kaplan, 2000; Decter, 2000), which remains controversial.
Both authors seem to be saying the same thing with respect to results but attach a totally different significance to the practical implications of the results. Kaplan (2000) points out that when the procedure was initiated the goal was to provide children with neurogenic bladder dysfunction, mostly secondary to spina bifida, enough sensation to detect a filling or full bladder and to have them synergistically void or catheterize in a timely manner. As the results of treatment have been evaluated over the years, the real benefit of this program, to Kaplan at least, was the potential to increase bladder capacity while maintaining or decreasing end-filling bladder pressure (in essence, improving compliance). Decter (2000) gives what seems to be a reasonable summation of the largest multi-institutional report of this therapy involving 568 patients who underwent TEBS at 11 institutions, only 335 of whom had adequate pretreatment and post-treatment urodynamics for evaluation. Bladder capacity increased by 20% or more in 56% of the 335 patients, whereas pressure at bladder capacity decreased by 25% or more in 16% of those in whom the bladder capacity increased. Decter (2000) calculated that only 30 of 335 patients had both a 20% or more increase in bladder capacity and a 25% decrease in compliance. He reports his experience with 25 patients during a 4-year period as showing that bladder capacity increased more than 20% (values referred to a comparison to age-adjusted bladder capacity) and end-filling bladder pressures showed clinically significant decreases in 29% of patients. Putting this in perspective, he believes “the practical benefits our patients derive seem limited…the urodynamic improvements we achieved after stimulation did not materially alter daily voiding routine (i.e., clean intermittent catheterization) of these children.” Pugach and associates (2000) also reported the results of TEBS in a group of pediatric patients; only 7 of 44 (16%) had safe storage pressures with continence after treatment. There exist limited new data since 2000 on this treatment modality probably owing to other better potential nerve targets.
Sacral Rhizotomy
Although technique refinements, such as percutaneous radiofrequency selective sacral rhizotomy and cryoneurolysis, have occurred, there is still controversy about the role of anterior rhizotomy procedures within a treatment plan for detrusor overactivity. Torrens (1985) summarized successful results in collected groups of patients that ranged from 48% for idiopathic overactivity to 81% for patients classified as having a “paraplegic bladder.” However, as he astutely pointed out, the definition of success varies from one series and from one patient to another. When these procedures are used, they should certainly be preceded by urodynamics and urologic evaluation of the effects of selective nerve blocks before performance, especially in patients without fixed neurologic disease or injury. Even then, unintended effects on pelvic and lower extremity sensory or motor functions may occur with disastrous medical and legal sequelae.
Both Tanagho and Schmidt (Tanagho and Schmidt, 1988; Tanagho et al, 1989) and Brindley (Brindley and Rushton, 1990) have popularized the concept of sensory deafferentation by dorsal or posterior rhizotomy to increase bladder capacity as part of their overall plan to simultaneously rehabilitate storage and emptying problems in patients with significant spinal cord injury or disease. These are patients in whom electrical stimulation also was used to alleviate emptying deficits (see section on emptying disorders). McGuire and Savastano (1984) also mentioned dorsal root ganglionectomy alone in such patients to increase bladder capacity.
Gasparini and colleagues (1992) reported durability of the deafferentation response to selective dorsal sacral rhizotomy up to 64 months after section. The technique involves selecting nerve roots whose intraoperative stimulation provokes an adequate detrusor response. The dorsal and ventral components of these roots are then separated and the dorsal root or roots severed. An increase in bladder capacity of 259 to 377 mL was noted in 16 of 17 patients studied (24 in the original series), with an increase in the volume to the first contraction of 99 to 270 mL. Fourteen patients were cured of incontinence, and 2 improved; the technique failed in 1 patient. Of seven potent men, two experienced a decrease in erectile frequency but were still able to achieve penetration. Bowel and sphincter function were unaffected. Koldewijn and associates (1994) reported on the effects of intradural bilateral posterior root rhizotomies from S2 to S5 with implantation of an anterior root stimulator in a group of patients with suprasacral spinal cord injury. All showed persistent detrusor areflexia afterward, although 2 required subsequent secondary rhizotomy at the level of the conus. A majority showed decreased bladder compliance up to 5 days postoperatively, followed by a rapid increase thereafter.
Brindley (1994) summarized the advantages of bilateral posterior sacral rhizotomy in treatment of voiding dysfunction after spinal cord injury as abolishing reflex incontinence, improving compliance, and abolishing striated sphincter dyssynergia without altering resting tone. Partial or selective procedures are considered only in such patients who retain some sensation or have excellent reflex erections. Madersbacher (2000) comments that posterior sacral rhizotomy for sacral deafferentation of the bladder is best achieved by the intradural approach, which has the advantage that, in this location, motor and sensory fibers can easily be separated, whereas distal to the spinal ganglion, motor and sensory fibers are intermingled and a clear separation is no longer possible. If an intradural procedure is not possible, he believes that a deafferentation at the level of the conus medullaris is preferable to an extradural sacral approach. He mentions that he has treated 65 tetraplegic or paraplegic patients with post–spinal cord injury reflex urinary incontinence who were resistant to all other means of conservative treatment. Incontinence was abolished in 90% of these patients.
Sacral Neuromodulation
Technique
Previous lead placement required a more time-consuming surgical dissection of the layers above the sacral foramen and unreliable lead fixation with anchors. Recent technical advances have made implantation of the percutaneous lead easier and less prone to migration. Spinelli and colleagues (2003) were the first to present the advantages of the tined lead that used a percutaneous approach for placement and fixation (Fig. 70–4). Subsequent large-scale clinical experience worldwide confirms that the tined lead is less prone to migration and has decreased false-negative results with the screening trial. Furthermore, the false-positive rate of the screening trial is reduced when placement of a permanent lead with reliable fixation during the screening trial ensures that the same location of stimulation is achieved when the IPG is implanted. With a percutaneous nerve evaluation or similar temporary lead electrode during the screening trial, a different clinical outcome may occur when the permanent lead is placed at the time of the IPG implantation.
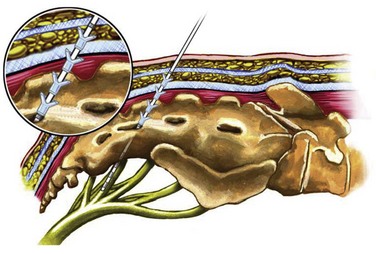
(Courtesy of Medtronic, Minneapolis, MN.)
The location of the S3 foramen is approximated by measuring 9 cm cephalad to the dropoff of the sacrum and 1 to 2 cm lateral to the midline on either side. Alternatively, the site may also be localized by palpating the cephalad portions of the sciatic notches bilaterally and drawing a connecting line that intersects the midline of the sacrum; one fingerbreadth on either side of the midline of the sacrum at this intersection will define the location of the S3 foramen (Fig. 70–5). The foramen needle is then inserted into the S3 foramen. The pelvic plexus and pudendal nerve run alongside the pelvis, and therefore the needle should be placed just inside the ventral foramen. The position of the needle is confirmed by fluoroscopy. The nerve is tested for the appropriate motor response, which is dorsiflexion of the great toe and bellows contraction of the perineal area, which represents contraction of the levator muscles (bellows reflex). Simultaneous sensory responses determined at the time of lead placement help optimize positioning. Although this is controversial, if one can localize the stimulation at the time of lead positioning to the vagina-rectum juncture in females and perineoscrotal area in males this is proper localization of the device. The foramen needle stylet is then removed and replaced with the introducer sheath. The distal aspect of the lead consists of four electrodes numbered 0 through 3. The lead is placed into the introducer sheath as directed to expose the electrodes. Typically, electrodes are positioned such that electrodes 2 and 3 straddle the ventral surface of the sacrum (Fig. 70–6). Test stimulation is repeated on each electrode, and the responses are observed. An S3 response should be noted on at least two of the electrodes (see Table 70–2). Once the surgeon is satisfied with the position, the sheath is removed, releasing the tines that anchor the lead. A sensory response, sensation of stimulation in the perineum, is not needed to confirm proper placement if the correct S3 motor response is observed. However, when a motor response is absent, raising the conscious level of the patient during the procedure and detecting the correct sensory response will confirm proper localization, and a clinical response may still be obtained during the screening trial period despite the absence of the motor response.
A stage II procedure entails placement of the IPG. No fluoroscopy is required during stage II when a permanent neuroelectrode has been placed for the stage I procedure; however, if a percutaneous nerve evaluation was performed for stage I, fluoroscopic confirmation of the neuroelectrode placement is advised. The patient may be placed in the prone position or a lateral position with the site of the previous lateral incision for the lead connections placed upward (Fig. 70–7). The lateral position may improve ventilation during sedation. The previous buttock incision overlying the lead connections is opened, the percutaneous extension wire is removed, and the extension lead is secured to the permanent lead and subsequently to the IPG. A pocket is made in the subcutaneous tissue that is large enough to avoid tension on closure and at a depth to provide a covering layer of subcutaneous tissue anterior to the pulse generator to prevent erosion.
Outcomes
Schmidt and coworkers (1999) reported on SNS therapy in 76 patients with refractory urgency urinary incontinence from 16 centers worldwide randomized to active or delayed therapy (control group) during the study period of 6 months. Of the 34 patients receiving active SNS therapy compared with the delayed group, 16 (47%) were completely dry and an additional 10 (29%) demonstrated more than 50% reduction in incontinence episodes. Complications were IPG site pain in 16%, implant infections in 19%, and lead migration in 7%.
In a similar study design, Hassouna and colleagues (2000) reported the outcomes of SNS on refractory urgency/frequency conditions in 51 patients randomized from 12 centers during an initial 6-month period that was extended to 2 years. Outcomes at 6 months in the active SNS group showed improvement in the number of daily voids (16.9 ± 9.7 to 9.3 ± 5.1), volume voided (118 ± 74 mL to 226 ± 124 mL), degree of urgency (rank score of 2.2 ± 0.6 to 1.6 ± 0.9), and SF-36 quality of life measures. At 6 months after implantation, stimulators in the active group were turned off and urinary symptoms returned to baseline values. After reactivation of SNS, sustained efficacy was documented at 12 and 24 months.
Limited but conformational results of the earlier randomized trials have been obtained from prospective series (Shaker and Hassouna, 1998; Siegel et al, 2000; Janknegt et al, 2001) and registry studies (Spinelli et al, 2001; Hedlund et al, 2002) evaluating efficacy, safety, and quality of life measures. The results for the registration trial that led to approval by the U.S. Food and Drug Administration (FDA) for SNS (Pettit et al, 2002) reveal that 37 of 62 patients (60%) with refractory urgency/frequency or urgency urinary incontinence achieved an improvement of 50% or more in their condition. van Kerrebroeck and coworkers (2007) have reported on a 5-year prospective worldwide series for patients with the InterStim device for varying indications and for the urgency/frequency and urgency urinary incontinence populations, and good success was achieved in all groups. Of note is that the analysis was of patients including those in the initial registration trial who then “crossed over” to the prospective 5-year trial. This study, unfortunately, was influenced by several patients who did not attend all follow-up visits and, therefore, had missing data points, a problem reflective of this challenging subgroup. Outcomes data were based on “last observation carried forward,” which is of concern for not being statistically optimal, but nonetheless these are still the longest-term data this technology has available.
Special Populations
Neurogenic Bladder
Multiple Sclerosis
No prospective randomized trials exist on the use of neuromodulation in management of MS-related bladder dysfunction. Small series with encouraging results in patients with MS demonstrate that neuromodulation may have a role in treatment of MS-related voiding dysfunction (Bosch and Groen, 1996; Wallace et al, 2007). It appears that the best candidates are ones with mild, nonprogressive MS, with few functional issues, who also have detrusor overactivity or even retention but not areflexia. One of the major issues that arises in the patient with MS in particular is the potential change in the disease state that may be potentiated by neuromodulation. Although this may be theoretical, it was one of the factors cited by the FDA in the original sacral neuromodulation trials and precluded MS patients from being enrolled (Hassouna et al, 2000).
A secondary issue that may arise if a patient with MS is implanted with the lead and IPG system is the potential need for MRI in the future. One should maintain close contact with the patient’s neurologist as to the decision to place a neuromodulatory device to prevent any future need for MRI or selection of a patient in an active phase of disease who requires routine MRI. The main concern with MRI and implantable stimulator or pacemaker-type devices is that heating of the leads has been demonstrated in vivo and in vitro (Roguin et al, 2004; Martin, 2005). Whereas some question the clinical significance of the small temperature changes with the leads, the potential exists to elicit nerve damage with heating of the lead, and the magnetic field may change the generator itself (Gimbel and Kanal, 2004). At present, it is contraindicated to perform MRI of a patient with an implantable neurostimulator system.
