INTRODUCTION
One of the more difficult concepts to grasp in nephrology is that changes in serum sodium concentration result from derangements in water balance, whereas disorders of extracellular fluid (ECF) volume regulation are related to total-body sodium balance. This is best explained by the fact that serum sodium is a concentration term and reflects only the relative amounts of sodium and water present in the sample. Low serum sodium concentration (shown in the equation below) denotes a relative sodium deficit and/or a relative water excess. Sodium concentration is not a measure of total body sodium content.
As seen in the formula above (Rose modification of the Edelman equation) the serum sodium concentration is equal to exchangeable sodium (eNa+) and potassium (eK+) divided by total-body water (TBW). As a result hyponatremia may occur from either a decrease in the numerator or an increase in the denominator. Although one might conclude that hyponatremia more likely results from a decrease in the numerator, in clinical practice, relative water excess most commonly causes hyponatremia. Nonosmotic arginine vasopressin (AVP) release is the key pathophysiologic process in most cases. Regulation of water homeostasis is dependent on (a) an intact thirst mechanism, (b) appropriate renal water handling, and (c) intact AVP release and response.
Renal-free water excretion is the major factor controlling water metabolism and the major factor controlling renal free water excretion is AVP. Above a plasma osmolality (Posm) of 283, AVP increases by 0.38 pg/mL per 1 mOsm/kg increase in Posm. In turn, urine osmolality (Uosm) responds to increments in AVP. A rise in AVP of 1 pg/mL increases Uosm approximately 225 mOsm/kg. The 2 major afferent stimuli for thirst are an increase in Posm and a decrease in ECF volume. Thirst is first sensed when Posm increases to 294 mOsm/kg (the osmolar threshold for thirst). At this osmolality AVP is maximally stimulated (concentration >5 pg/mL) and is sufficient to maximally concentrate urine. AVP and angiotensin II directly stimulate thirst.
Osmolality is an intrinsic property of a solution and is defined as the number of osmoles of solute divided by the number of kilograms of solvent. It is independent of a membrane. Tonicity or “effective osmolality” is equal to the sum of the concentration of solutes with the capacity to exert an osmotic force across a membrane. It is a property of a solution relative to a membrane. The tonicity of a solution is less than osmolality by the total concentration of “ineffective solutes” that it contains. Solutes that are freely permeable across cell membranes such as urea are ineffective osmoles. From a cellular viewpoint, tonicity determines the net osmolar gradient across the cell membrane that acts as a driving force for water movement.
Sodium is the most abundant cation in ECF and its concentration is the major determinant of tonicity and osmolality. Furthermore, water moves freely across cell membranes allowing the maintenance of osmotic equilibrium between various compartments, therefore ECF tonicity reflects tonicity of the intracellular fluid (ICF). Posm is calculated from the following formula:
To calculate tonicity one includes only the sodium and glucose terms in the equation. It is measured directly by freezing point depression or vapor pressure techniques.
Body tonicity, measured as Posm, is maintained within a narrow range (285 to 295 mOsm/kg). This is achieved via regulation of water intake and excretion. Disturbances in body tonicity are reflected by alterations in serum sodium concentration and clinically present as either hypo- or hypernatremia.
KEY POINTS
HYPONATREMIA
Hyponatremia, defined as a serum sodium concentration less than 135 mEq/L, is the most frequent electrolyte abnormality and is seen in up to 15% of hospitalized patients. It is especially common in critical care units. Hyponatremia is caused by either (a) excess water intake (water intoxication) with normal renal capacity to excrete solute-free water or (b) continued solute-free water intake with a decreased renal capacity for solute-free water excretion. It occurs whenever free water intake exceeds free water losses.
In subjects with normal renal function excessive water intake alone does not cause hyponatremia unless it exceeds approximately 1 L/h. As a general rule one’s maximal free water excretion is equal to approximately 10 to 15% of glomerular filtration rate (GFR). With a GFR of 180 L/day, maximal free water excretion equals approximately 24 L/day or 1 L/h. In patients with a normal GFR, hyponatremia caused by excessive water intake is observed only rarely, such as in psychotic patients who drink from faucets or showers. A reduction in GFR, however, will limit free water excretion. An individual whose GFR is 20% of normal will become hyponatremic on drinking more than 4.8 L/day. Often patients with psychogenic polydipsia have some degree of renal impairment.
Almost all hyponatremic patients have impaired renal free water excretion. An understanding of how the kidney excretes free water is critical for understanding the pathophysiology of hyponatremia.
Essential features of renal free water excretion are the following:
1. Normal delivery of tubular fluid to distal diluting segments of the nephron. An adequate GFR without excessive proximal tubular reabsorption is required to deliver tubular fluid to the diluting segments of the kidney (thick ascending limb of the loop of Henle and distal convoluted tubule [DCT]). Although tubular fluid remains isotonic in the proximal tubule, proximal fluid reabsorption is an important determinant of water excretion. Normally 60-70% of glomerular filtrate is reabsorbed in the proximal tubule and the remaining 30-40% is isotonic to plasma as it enters the loop of Henle. Thus, if proximal tubular reabsorption increases, as in volume depletion, free water excretion is limited. To use an extreme example, a patient with acute kidney injury and a GFR of 5 mL/min forms only 7.2 L of glomerular filtrate daily. If 30% is delivered to the diluting segments, that means a total of only 2.2 L is delivered daily. Even if the distal nephron were completely impermeant to water, only 2.2 L of urine is excreted (only part of this total is free water).
2. Normal function of the diluting segments (ascending limb of the loop of Henle and DCT). Tubular fluid is diluted in the water-impermeable ascending limb of the loop of Henle and DCT by the reabsorption of sodium chloride. Sodium is transported on the Na+-K+-2Cl– cotransporter in the thick ascending limb of Henle and the thiazide-sensitive NaCl cotransporter in DCT. It is in the diluting segments, where Uosm declines to less than Posm, that free water is generated.
3. Absence of AVP. Arginine vasopressin must be suppressed so as to prevent solute-free water reabsorption in the collecting duct. This factor is of primary importance because the renal interstitium remains slightly hypertonic even during a water diuresis. Therefore, if the collecting duct were water permeable, osmotic equilibration of fluid between the tubular lumen and interstitium would concentrate the urine and impair water excretion.
AVP is released from the posterior pituitary, enters the bloodstream, binds to its receptor (V2) in the basolateral membrane of the collecting duct, adenylate cyclase is activated, cyclic adenosine monophosphate (AMP) generated, and water channels (aquaporins—AQP2) insert into the apical membrane increasing membrane water permeability. AVP also increases the expression of the UT-1 urea transporter in the inner medullary collecting duct facilitating urea reabsorption and increasing the osmolality of the medullary interstitium.
AVP is released in response to osmotic and nonosmotic stimuli. An increase in ECF osmolality as little as 1% stimulates AVP release and the relationship of AVP to Posm is linear (Figure 3.1). Nonosmotic stimuli are associated with changes in autonomic neural tone such as physical pain, stress, hypoxia, and decreases in effective arterial blood volume. The nonosmotic pathway is less sensitive and requires a 5% to 10% decrement in blood volume to stimulate AVP release. Once the threshold is reached, however, the rise in AVP concentration is geometric (Figure 3.1). Defense of volume has priority. AVP concentration increases and stimulates renal water reabsorption protecting volume at the expense of hyponatremia. It is more important for the body to maintain blood volume than to maintain tonicity. The volume-depleted patient may become profoundly hyponatremic because nonosmotic stimuli for AVP release predominate over osmotic stimuli. AVP also has a pressor effect mediated via the V1 receptor, contributing perhaps 10% to mean arterial pressure during volume depletion. Thus, AVP is normally osmoregulatory, but during stress becomes a volume regulatory hormone. As a general principle the kidney will always act to preserve blood and ECF volume at the expense of electrolyte and acid–base homeostasis. Nonosmotic AVP release is the key pathophysiologic process in the majority of patients with hyponatremia.
4. Adequate solute intake. Although the kidney has an enormous capacity to generate free water, it cannot excrete pure water. The lowest Uosm attainable in humans is 50 mOsm/kg. One of the main roles of the kidney is to eliminate the dietary osmolar load (approximately 10 mOsm/kg). The volume of urine required to achieve this is expressed in the equation below:
FIGURE 3-1. Changes in plasma AVP induced by alterations in osmolality or blood volume. Note that response to changes in osmolality are linear, whereas response to changes in blood volume approximates an exponential curve.
In the steady state, osmolar intake and excretion are equal and either can be used. In theory a 70 kg person with a standard osmolar dietary load and a maximally dilute urine could generate 14 L of urine per day (700 mOsm/50 mOsm). If solute intake is very low, however, as in someone drinking only beer (beer drinker’s potomania), hyponatremia could develop despite the fact that urine is maximally dilute. For example, if solute intake were only 150 mOsm/day with a maximally dilute urine, urine volume would be only 3 L. In this situation water intake could exceed renal free water excretion and hyponatremia could develop.
KEY POINTS
Etiology
Hyponatremia most commonly results from an inability to maximally dilute the urine coupled with continued water intake. Before implicating a defect in renal free water excretion as the cause of hyponatremia, the presence of hypoosmolality must be documented because hyponatremia can occur with an elevated or normal serum osmolality.
Hyponatremia with a normal serum osmolality or “pseudohyponatremia” is a laboratory artifact. Plasma is made up of 2 fractions—an aqueous fraction and a particulate fraction. Pseudohyponatremia results from a decrease in the aqueous fraction. Many modern autoanalyzers dilute an aliquot of serum before measuring sodium concentration (indirect potentiometry). When correcting for this dilution an assumption is made that the sample is 93% aqueous and 7% particulate. If the aqueous fraction is less than 93%, pseudohyponatremia occurs. Conditions that reduce the aqueous fraction below the usual 93% of plasma (the remaining 7% is the particulate fraction made up of proteins and lipids) decrease the total amount of sodium per aliquot of plasma. Sodium concentration, however, in the aqueous fraction is normal. Three conditions that reduce the aqueous fraction are hyperlipidemia, hypercholesterolemia, and hyperproteinemia. This is not a common problem. A clue to the presence of hypertriglyceridemia is a report from the lab of lipemic serum. Lipemic serum means that after centrifugation of whole blood the supernatant is cloudy. For each 460 mg/dL increase in triglyceride concentration the serum sodium concentration falls by 1 mEq/L. Elevations in lipoprotein X concentration do not result in lipemic serum. Lipoprotein X is elevated in patients with intrahepatic and extra-hepatic cholestasis and in those with lecithin cholesterol acyltransferase (LCAT) deficiency. Excess production of paraproteins as in multiple myeloma and administration of intravenous immunoglobulin also increase the particulate fraction and may result in pseudohyponatremia. Immunoglobulin (Ig) G preparations that contain maltose or sucrose may also cause hyponatremia secondary to water translocation from the intracellular space. Measurement of serum sodium concentration by ion-sensitive electrode (direct potentiometry) will yield a normal sodium concentration.
Translocational hyponatremia is caused by water shifting out of cells in response to a nonsodium solute. Serum osmolality is elevated. Water moves down an osmotic gradient from ICF to ECF when nonsodium solute increases ECF osmolality and creates a driving force for water movement. The most common cause is hyperglycemia. Mannitol and glycine infusion also cause translocational hyponatremia. For each increase in serum glucose of 100 mg/dL above its normal concentration, serum sodium concentration falls by 1.6 mEq/L. This is a calculated correction factor. In practice this rule of thumb works well for glucose concentrations up to 400 mg/dL or in patients who are on dialysis with little or no residual renal function. At higher concentrations the correction factor is likely larger (2.0 to 4.0 mEq/L), which may be because of a combination of water translocation out of cells and free water loss in urine. Glycine has been used as an irrigation fluid in patients undergoing transurethral resection of the prostate (TURP). Systemic glycine absorption can result in the post-TURP syndrome. This is characterized by a variety of symptoms including confusion, nausea, vomiting, chest pain, hypotension, paresthesia, and anxiety. Newer bipolar resection systems that permit sodium chloride use as an irrigating solution are associated with a lower incidence of hyponatremia and post-TURP syndrome. For each 100 mg/dL rise in glycine concentration the serum sodium concentration falls by 3.8 mEq/L. An elevated serum osmolar gap may be a clue to the presence of pseudo or translocational hyponatremia.
The remaining causes of hyponatremia alter external water balance and are associated with low serum osmolality (true hyponatremia). True hyponatremia is caused by either (a) excess water intake (water intoxication) with normal renal capacity to excrete free water or (b) continued solute-free water intake with a decreased renal capacity for solute-free water excretion. The most common pathophysiologic mechanism is nonosmotic AVP release that prevents maximal urinary dilution. Rarely, severely depressed urine flow rate, as with low GFR, increased proximal tubule fluid reabsorption, or decreased solute intake limits urine dilution resulting in positive water balance and hyponatremia.
A clue to the source of the increased AVP concentration lies in the evaluation of the patient’s volume status. Common causes are edematous states, extrarenal and renal sodium and water losses, serum inappropriate antidiuretic hormone (SIADH), and psychogenic polydipsia.
The presence of edema is indicative of increased total-body sodium. Hyponatremia results because the increase in total-body water exceeds the increase in total-body sodium. In these circumstances, effective circulating volume is decreased and volume/pressure receptors are activated, releasing AVP (note the similarity to signal for sodium retention). Thus a decreased effective arterial blood volume is sensed despite an absolute increase in total-body salt and water. The increase in AVP is “appropriate” to the sensed signal. Major causes of hyponatremia with increased total-body sodium are congestive heart failure, hepatic cirrhosis, nephrotic syndrome, and advanced chronic or acute kidney injury. The hallmark of these disorders on physical examination is dependent edema.
Renal and extrarenal salt and water losses are characterized by signs and symptoms of decreased ECF volume such as thirst, orthostatic hypotension, tachycardia, and decreased skin turgor. In this setting AVP release is “appropriate” to defend ECF volume. Total-body sodium loss exceeds total-body water loss. Common etiologies of hyponatremia with decreased ECF volume include gastrointestinal losses (excessive salt and water loss causes sufficient hypovolemia to stimulate baroreceptors to increase AVP release); third spacing of fluids; burns; pancreatitis; diuretic overuse or abuse; salt-wasting nephropathy; adrenal insufficiency; osmotic diuresis; and the hyponatremia hypertensive syndrome. Tuberculosis remains the most common cause of adrenal insufficiency worldwide. Adrenal insufficiency often presents with subtle findings and as a result the diagnosis is often delayed. Renal salt wasting has been reported with cisplatinum thought to be a result of proximal tubular injury. The hyponatremia hypertensive syndrome is a result of unilateral renal artery stenosis. It is characterized by hyponatremia, hypertension, hypokalemia, polydipsia, and polyuria. It has been most commonly described in elderly women.
With extrarenal fluid loss the sodium concentration of the lost fluid is less than the serum sodium concentration. If this is the case, how does the patient become hyponatremic? The answer lies in the fact that thirst is intact and that the replacement fluid has a lower sodium concentration than the fluid lost.
Hyponatremia from diuretics is almost always a result of thiazide rather than loop diuretics, as thiazides interfere with urinary dilution but not urinary concentrating ability. By contrast, loop diuretics interfere with both diluting and concentrating ability, and result in medullary washout of solute and diminished AVP-induced free water reabsorption. Diuretic-induced volume depletion decreases GFR and increases proximal tubular salt and water reabsorption, thereby decreasing water delivery to distal segments. Potassium depletion may result in intracellular sodium shifts, and alters the sensitivity of the osmoreceptor mechanism leading to AVP release. Most patients have an associated hypokalemic metabolic alkalosis. Older women are at highest risk, and this generally occurs in the first 2 to 3 weeks of therapy. Mineralocorticoid- and glucocorticoid-deficient states lead to volume depletion with enhanced proximal tubular reabsorption and nonosmotic stimulation of AVP release.
Hyponatremia in the presence of a clinically normal ECF volume is most commonly the result of SIADH, drug-induced euvolemic hyponatremia, or psychogenic polydipsia. The term clinically normal should be stressed. If total-body sodium and total-body water were truly normal, then serum sodium concentration must also be normal. In reality, total-body water is increased as a result of “inappropriate” AVP release. “Inappropriate” implies that AVP is released despite the absence of the 2 physiologic stimuli for its release: increased serum osmolality; and decreased effective arterial blood volume. This state of mild volume expansion results in urinary sodium wasting and a clinically undetectable decrease in total body sodium. SIADH is characterized by hyponatremia, a low serum osmolality, and an inappropriately concentrated urine (less than maximally dilute). Urine sodium concentration is generally increased but it can be low if the patient develops ECF volume depletion. The patient must be clinically euvolemic with no evidence of adrenal, renal, or thyroid dysfunction; and not taking a drug that stimulates AVP release or action. SIADH is caused by malignancies, pulmonary, or central nervous system disease (Table 3.1). The most common tumor-producing SIADH is small cell cancer of the lung. In some patients with small cell lung cancer and hyponatremia, the tumor produces natriuretic peptides and not AVP. This is an important disorder to diagnose because hyponatremia will worsen if normal saline is administered. Why do patients with SIADH continue to drink fluid despite a low serum sodium concentration? One study suggested that the osmotic threshold for thirst was reset downward and that thirst occurred around a lower set-point.
TABLE 3-1. Disease Processes Causing Syndrome of Inappropriate Antidiuretic Hormone
Several disorders may be clinically difficult to distinguish from SIADH. Nephrogenic syndrome of inappropriate antidiuresis is the result of a gain of function mutation in the V2 receptor. It mimics SIADH but when measured, AVP levels are undetectable. Cerebral salt wasting is a disorder associated with a variety of intracranial lesions characterized by a high urine sodium and hyponatremia and could result from disruption of sympathetic input to the kidney or elaboration of natriuretic peptides from the brain. The majority of described cases are more likely related to SIADH than to cerebral salt wasting. The diagnosis of cerebral salt wasting requires ongoing renal salt loses in the presence of hypovolemia. These patients are often administered large volumes of normal saline or 3% saline and in this setting renal excretion of the salt load is expected.
A variety of drugs impair renal free water excretion by potentiating the action or release of AVP and may result in drug-induced euvolemic hyponatremia. Table 3.2 provides a partial list of drugs that induce euvolemic hyponatremia. This is most commonly caused by the SSRI (selective serotonin reuptake inhibitor) group of antidepressants. Although hyponatremia can present more than 3 months after the start of an SSRI, it generally occurs in the first 2 weeks of therapy and the elderly are at increased risk. SSRIs stimulate water reabsorption in the collecting duct via a non-antidiuretic hormone (ADH)-mediated modulation of aquaporin 2. High dose (>50 mg/kg) cyclophosphamide was at one time a common cause of drug-induced euvolemic hyponatremia. Current low-dose regimens (<20 mg/kg) rarely result in hyponatremia. This is likely the result of the metabolites mafosfamide and 4-hydroperoxy-cyclophosphamide that increase interleukin (IL)-1 and tumor necrosis factor (TNF)-α that downregulate the V2 receptor and aquaporin 2. Drug-induced hyponatremia can also occur after desmopressin administration for the treatment of enuresis. The elderly with mild hyponatremia or those taking other drugs that may impair renal water excretion are at increased risk of severe hyponatremia. As a result, several European countries have removed the enuresis indication for intranasal desmopressin.
TABLE 3-2. Drug-Induced Euvolemic Hyponatremia
In hypothyroidism the ability of the kidney to excrete free water is impaired by a decrease in GFR, an increase in proximal tubular reabsorption, and an increase in AVP secretion. In secondary adrenal insufficiency hyponatremia results because glucocorticoids are required to maximally suppress AVP release.
Psychogenic polydipsia or water intoxication is the result of excess water intake with normal renal capacity for free water excretion. It is differentiated from SIADH in that the Uosm is maximally or near maximally dilute. This commonly occurs in patients with psychiatric disease on psychotropic medications that result in dry mouth and increased water intake. Schizophrenia is associated with psychogenic polydipsia more than twice as often as non-schizophrenic psychiatric illnesses. Hyponatremia with a low Uosm is also seen in those with beer drinker’s potomania whose renal free water excretion is limited by solute intake.
KEY POINTS
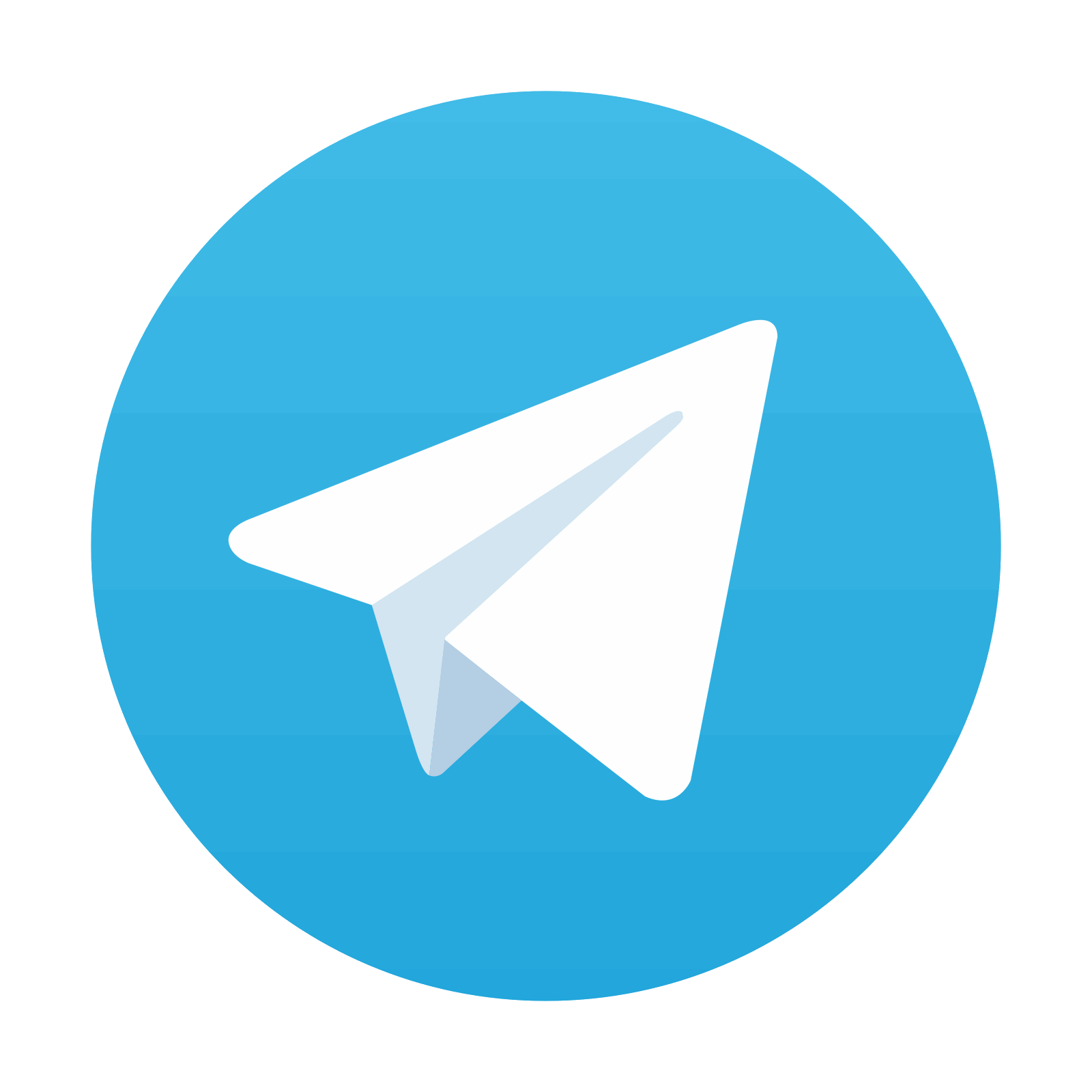
Stay updated, free articles. Join our Telegram channel

Full access? Get Clinical Tree
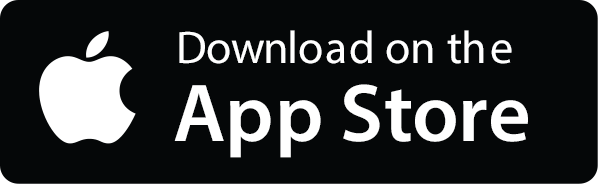
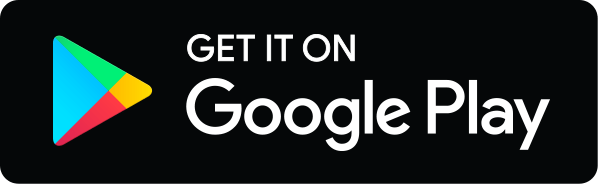