CHAPTER
10
Disorders of Calcium Homeostasis—Hypo and Hypercalcemia
Recommended Time to Complete: 1 Day
REGULATION OF EXTRACELLULAR FLUID IONIZED CALCIUM
Calcium (Ca2+) in the body plays a key structural role in the bony skeleton and as a messenger in extracellular and intracellular signaling. Of the 1 kg of Ca2+ present in the body, 99% is in bones and teeth. The remaining 1% is present in the ECF whose concentration is tightly regulated. Normal plasma concentration is 8.8 to 10.3 mg/dL (2.2 to 2.6 mM or 4.4 to 5.2 mEq/L). Sixty percent of ECF calcium is ultrafilterable (50% free and 10% complexed to anions) and the other 40% is bound to plasma proteins (mainly albumin). Ionized calcium concentration ([iCa2+]), normally between 1.05 and 1.23 mM, is regulated by 3 hormones (PTH, calcitriol, and calcitonin), 3 target organs (kidney, bone, and parathyroid gland), and a sensor (calcium-sensing receptor) to detect ECF Ca2+ concentration. The vast majority of total-body calcium exists as hydroxyapatite in bone (99%). This reservoir is so large that one cannot become hypocalcemic without a decrease in bone calcium release as a result of a defect in either PTH or calcitriol concentration or action. The intracellular iCa2+ concentration (0.1 μM) is maintained at very low levels by sequestering Ca2+ into the endoplasmic reticulum and mitochondria, or actively extruding Ca2+ across plasma membranes by the Ca2+-adenosine triphosphatase (ATPase) pump (present in all cells) and the sodium-calcium exchanger (NCX) (present in certain tissues).
Figure 10.1 illustrates average daily calcium fluxes between ECF and organ systems involved in its regulation (bone, intestine, and kidney). The average adult takes in 1000 mg and absorbs approximately 20% in intestine (primarily passive process). In the steady state, intestinal absorption is matched by urinary Ca2+ excretion. Most of the calcium absorption in the small intestine (primarily duodenum) is passive, with a small fraction absorbed by active transport. The kidney excretes approximately 2% (200 mg) of the filtered calcium load and acts as a principal regulatory organ for extracellular Ca2+ balance. Approximately 65% of the filtered Ca2+ load is reabsorbed in proximal tubule. The majority occurs across the paracellular space with a small active component. Twenty-five percent is reabsorbed in the thick ascending limb (TAL), driven by the lumen-positive potential difference generated by the actions of ROMK, as well as claudin-16 and -19. The remaining 8% is actively reabsorbed transcellularly in the distal convoluted tubule and connecting tubule. Calcium entry into the distal nephron is mediated via TRPV5 (transient receptor potential vanilloid-5) channels that are regulated by secreted klotho and PTH. Once calcium enters the cell in kidney and duodenum, it binds to calbindin (intracellular shuttling proteins) and is extruded across the basolateral membrane by NCX1. Fractional excretion of Ca2+ is 1% to 2%, but even small changes in distal nephron reabsorption lead to large effects on the final amount of Ca2+ in urine.
FIGURE 10-1. Calcium homeostasis. Daily calcium fluxes between ECF, intestine, kidney, and bone are shown. In the steady state, net intestinal absorption and renal calcium excretion are equal. The majority of calcium in the body is in bone. (With permission from Schrier RW, ed. Manual of Nephrology. Philadelphia, PA: Lippincott Williams & Wilkins; 2000.)
The calcium-sensing receptor (CaSR), a G-protein coupled receptor, is expressed in the plasma membrane of the parathyroid gland and the basolateral membrane of the TAL of Henle in kidney. In the collecting duct, the CaSR is expressed in the apical membrane. Its activation stimulates urinary acidification and reduces water reabsorption via aquaporin-2 (AQP2) downregulation. In the parathyroid gland, it couples changes in ECF [iCa2+] to the regulation of PTH secretion mediated by activating phospholipase C (PLC) and reducing intracellular cyclic adenosine monophosphate (cAMP) levels. High [iCa2+] activates the CaSR and inhibits PTH release, whereas low [iCa2+] has opposite effects on PTH secretion within minutes of the changes. There is an inverse sigmoidal relationship between [iCa2+] and PTH secretion (Figure 10.2). The CaSR knockout mouse demonstrates marked parathyroid hyperplasia, suggesting that the receptor also plays a role in parathyroid cell growth and proliferation. A rise in PTH induces 25-hydroxyvitamin D-1α-hydroxylase, which increases serum calcitriol levels and induces intestinal Ca2+ absorption. Hypercalcemia induces thyroid C-cells to secrete calcitonin, a hormone that inhibits osteoclast mediated bone resorption.
FIGURE 10-2. PTH-calcium response curve. There is an inverse sigmoidal relationship between ionized calcium concentration and PTH release from the parathyroid gland. The set-point is that ionized calcium concentration at which PTH release is inhibited by 50%. The minimum arrow illustrates that there is a basal level of PTH release even at high calcium concentration.
Furthermore, extracellular Ca2+ has hormone-like action on the kidney mediated by the CaSR expressed on the basolateral membrane of TAL. Activation of CaSR by high [iCa2+] results in inhibition of apical sodium entry via the furosemide-sensitive Na+-K+-2Cl– cotransporter. Simultaneously, inhibition of the apical membrane potassium channel (ROMK) occurs by activating PLC, cAMP and arachidonic acid intermediates, resulting in a reduction of the lumen-positive voltage that drives paracellular calcium transport. This results in increased urinary calcium excretion. In addition, the ability of the kidney to concentrate urine is also impaired as a consequence of inhibition of the Na+-K+-2Cl– cotransporter. Hypercalciuria, also, induces collecting tubule insensitivity to vasopressin-mediated water reabsorption via a reduction of aquaporin channels in the luminal membrane.
In the distal nephron, secreted klotho cleaves the sialic acid present on galactose residues of the N-terminus of the TRPV5 channel, which acts as a binding site for galectin-1, resulting in increased TRPV5 channels in the apical membrane because of reduced caveolae-mediated endocytosis. PTH via the PTH 1 receptor inhibits the sodium-proton exchanger (NHE3) in proximal tubule and reduces bicarbonate reabsorption. PTH increases TRPV5 gene expression in the distal nephron. PTH also increases TRPV5 activity via cAMP-protein kinase A (PKA)-mediated phosphorylation. TRPV5 activity is pH sensitive. Increases in intracellular pH increase open probability of TRPV5 resulting in channel recruitment to the apical membrane. Reductions in pH have opposite effects.
Calcitriol increases calcium and phosphorus availability for bone formation and prevents hypocalcemia and hypophosphatemia. In intestine and kidney, calcitriol plays an important role in stimulating calcium transport via increasing calcium binding protein (calbindin) expression. Calbindins bind calcium and shuttle it from the apical to the basolateral membrane, thereby allowing calcium to move through the cell without an increase in free intracellular calcium concentration. Calcitriol increases sodium phosphate cotransporter expression in intestine. In bone, calcitriol has a variety of effects: (a) potentiation of PTH effects; (b) stimulation of osteoclast-mediated reabsorption; and (c) induction of monocyte differentiation into osteoclasts. In parathyroid gland, calcitriol binds to its receptor in the cytoplasm and forms a heterodimer with the retinoid X receptor and is translocated to the nucleus. The complex binds to the PTH gene promoter and decreases PTH expression, as well as inhibits parathyroid growth.
Chronic thiazide administration causes hypocalciuria thought to be mediated by a combination of increased proximal and distal Ca2+ reabsorption. This process is similar to that seen in patients with Gitelman syndrome. The highest incidence of thiazide-induced hypercalcemia is observed in 70- to 79-year-old women after a mean of 6 years of thiazide ingestion. Interestingly, many of these patients are eventually diagnosed with primary hyperparathyroidism (PHPT) as the hypocalciuric effects of the thiazide are thought to uncover mild PHPT. If hypercalciuria persists after thiazide diuretics are stopped, a search for a parathyroid adenoma should be undertaken.
KEY POINTS
HYPERCALCEMIA
Etiology
Hypercalcemia results from increased calcium absorption from gastrointestinal (GI) tract, increased bone resorption, or decreased renal calcium excretion (Table 10.1).
TABLE 10-1. Etiologies of Hypercalcemia
Increased GI calcium absorption is important in hypercalcemia that results from the calcium-alkali syndrome (formerly called the milk-alkali syndrome), vitamin D intoxication, and granulomatous diseases. Calcium-alkali syndrome results from excessive intake of calcium and bicarbonate or its equivalent. In addition, alkalosis stimulates calcium reabsorption in the distal nephron. Suppression of PTH secretion by hypercalcemia further increases proximal tubular bicarbonate reabsorption. The most common cause of the calcium-alkali syndrome in the past was milk and sodium bicarbonate ingestion for therapy of peptic ulcer disease, hence the former name milk-alkali syndrome. Today, the most common clinical setting is an elderly woman with history of hypertension, chronic kidney disease (CKD), osteoporosis, and an upper GI disorder, who is treated with diuretics and calcium-vitamin D supplementation. Typically, PTH and calcitriol levels are suppressed in such patients, despite the presence of CKD. Alkalosis, renal dysfunction, and hypercalcemia maintain a vicious cycle. ECF volume depletion maintains alkalosis that triggers increased GI absorption and decreased urinary calcium excretion (TRPV5 is pH sensitive), resulting in hypercalcemia. Hypercalcemia triggers volume depletion, free water loss, and nausea, perpetuating metabolic alkalosis. Bulemics taking supplemental calcium or on a highcalcium diet are also at high risk for the calcium-alkali syndrome. Treatment of these patients is often complicated by rebound hypocalcemia as a result of sustained PTH suppression from hypercalcemia. This is more likely in patients who are treated with bisphosphonates. For this reason bisphosphonates in patients with calcium–alkali syndrome should only be used in those with severe hypercalcemia resistance to more conventional therapies (volume expansion, loop diuretics, calcitonin). Disorders that may increase the likelihood of hypocalcemia, such as hypomagnesemia, should be corrected. Typically, patients are managed by volume resuscitation and small doses of loop diuretics for calciuresis. Bisphosphonates are not used as a first line of therapy because of the risk of rebound hypocalcemia; they are best reserved for resistant cases. Concomitant metabolic abnormalities, such as vitamin D deficiency and hypomagnesemia, should be corrected to prevent rebound hypocalcemia.
Hypercalcemia from increased calcium ingestion (reported ingestion between 1 and 9 g) alone rarely occurs in the absence of renal dysfunction or vitamin D supplementation (reported ingestion of as little as 400 to 800 units per day or calcitriol 0.25 μg per day). Vitamin D intoxication also causes hypercalcemia. Figure 10.3 shows vitamin D metabolism and formation of calcitriol in the body. Calcitriol stimulates calcium absorption in small intestine; however, bone calcium release may also play an important role in these patients. An outbreak of hypercalcemia was reported as the result of overfortification of milk from a home-delivery dairy. Other milk-associated outbreaks have resulted from the inadvertent addition of calcitriol to milk. Increased GI calcium absorption and hypercalcemia occur with granulomatous disorders, such as sarcoidosis, Mycobacterium tuberculosis, and Mycobacterium avium in patients with human immunodeficiency virus (HIV) infection. Macrophages express 1 α-hydroxylase when stimulated and convert calcidiol to calcitriol. Hypercalcemia may be the initial manifestation of extrapulmonary sarcoid. Hypercalciuria may precede hypercalcemia in sarcoid. Lymphomas can produce hypercalcemia via the same mechanism. The source of calcitriol with lymphomas may be from macrophages adjacent to the tumor and not the malignant cells themselves. Lymphomas may also cause hypercalcemia via cytokine-induced activation of osteoclasts and osteolysis.
FIGURE 10-3. Vitamin D metabolism. The metabolic pathway is illustrated.
Increased bone calcium resorption is the most common pathophysiologic mechanism leading to hypercalcemia. This plays a primary role in the hypercalcemia of PHPT, malignancy, hyperthyroidism, Paget disease, and immobilization. Burn patients who are immobilized for prolonged periods (≥3 weeks) are at high risk for hypercalcemia. The 2 most common causes of hypercalcemia are PHPT and malignancy.
PHPT occurs in as many as 2 per 10,000 people in the general population. In the 1970s and 1980s the incidence was as high as 8 per 10,000 people, but has been steadily declining since. It is speculated that therapeutic radiation of the neck for thymic enlargement and acne in the 1940s and 1950s may have contributed to the higher incidence. The pathologic lesion in 90% is a solitary adenoma. Multiple adenomas can occur and parathyroid carcinoma is very rare (<1%). Of the remaining, as many as 10%, have diffuse hyperplasia and some of these have the inherited familial syndrome multiple endocrine neoplasia (MEN). MEN type I is associated with pituitary adenomas and islet cell tumors. It has an estimated prevalence of 1 per 50,000 in the general population. PHPT is the initial manifestation, occurring in general by age 40 to 50 years. The mutation resides in the menin gene. Menin is a tumor suppressor expressed in the nucleus that binds to JunD. Menin mutations occur in approximately 15% of sporadic adenomas. MEN type II is associated with medullary carcinoma of the thyroid and pheochromocytoma. It is subdivided into MEN IIa that is associated with parathyroid hyperplasia and type IIb that is not. MEN type II is caused by mutations in the RET protooncogene that is a tyrosine kinase. In developing tissues, including neural crest, kidney, and ureter, RET is a receptor for growth and differentiation.
Hypercalcemia in PHPT is the combined result of increased bone calcium resorption, increased intestinal calcium absorption, and increased calcium reabsorption in kidney. In PHPT, hypercalcemia is mild (< 11.0 mg/dL), and often identified on routine laboratory testing in the asymptomatic patient. Patients present most commonly between the ages of 40 and 60 years and women are affected 2 to 3 times more often than men. The majority of patients are postmenopausal women. There are some case reports of normocalcemic PHPT, which presents with osteoporosis and/or fragility fracture with nephrolithiasis, despite the absence of hypercalcemia. Such patients have elevated serum calcitriol levels.
Secondary hyperparathyroidism-induced hypercalcemia occurs in 2 clinical settings. In the renal transplantation patient, although renal function improves, PTH concentration remains elevated as a result of increased parathyroid gland mass. Hypercalcemia generally does not persist more than a year. In the patient with end-stage renal disease and secondary hyperparathyroidism, hypercalcemia can occur with calcium and/or vitamin D supplementation. This occurs primarily in patients with low-turnover bone disease (adynamic bone disease).
Malignancy results in hypercalcemia from production of parathyroid hormone-related peptide (PTHrP), local bone resorption in areas of metastasis (cytokine mediated), or calcitriol production (lymphomas). Breast cancer, squamous cell lung cancer, multiple myeloma, and renal cell carcinoma are the most common malignancies associated with hypercalcemia. Hypercalcemia secondary to PTHrP is known as humoral hypercalcemia of malignancy (HHM). A large variety of tumors can produce PTHrP. A partial list includes; squamous cell cancers of the head, neck, and lung; breast cancer; pancreatic cancer; transitional cell carcinomas; and germ cell tumors. The first 13 amino acids of PTHrP are highly homologous to PTH, resulting in PTHrP binding to the PTH 1 receptor and affecting renal tubular Ca2+ reabsorption via the cAMP and PLC-mediated pathways. PTHrP may be the fetal PTH. PTH is not secreted by the parathyroid gland in utero and does not cross the placenta.
Humoral hypercalcemia of malignancy typically presents with severe hypercalcemia (calcium concentration >14 mg/dL). At the time of initial presentation the cancer is usually easily identified. PTHrP is immunologically distinct from PTH and as a result is not detected by PTH assays. An assay for PTHrP is commercially available. In patients with HHM PTH concentration will be low. Hypercalcemia from PHPT and HHM can rarely be seen in the same patient. Osteolytic metastases produce a variety of cytokines resulting in calcium release from bone. Tumor necrosis factor (TNF) and interleukin (IL)-1 stimulate the differentiation of osteoclast precursors into osteoclasts. IL-6 stimulates osteoclast production.
Approximately one-third of patients with multiple myeloma will develop hypercalcemia. Multiple myeloma presents with anemia, hypercalcemia, and localized osteolytic lesions. Release of calcium from bone results from cytokine release (IL-6, IL-1, TNF-β, macrophage inflammatory protein [MIP]-1α and MIP-1β). Myeloma cells can disturb the balance between osteoprotegerin (a decoy receptor for RANKL (receptor activator of nuclear factor kappa-B)) and RANK. This balance plays a critical role in bone remodeling and maintaining the ratio of osteoclast to osteoblast activity. The balance tips in favor of bone resorption when RANKL expression increases and osteoprotegerin decreases. Lytic bone lesions are characterized by increased osteoclast resorption without new bone formation. This is in contradistinction to bone metastases with breast and prostate cancer where areas of lysis are surrounded by new bone formation. As a result, radionuclide bone scans will show uptake at sites of metastasis but not at sites of bone involvement with multiple myeloma.
Increased bone turnover and mild hypercalcemia occur in 5% to 10% of patients with hyperthyroidism. Hyperthyroid patients may also have an increased incidence of parathyroid adenomas. Immobilization and Paget disease can cause hypercalcemia. Immobilization leads to imbalance between the rate of bone resorption and bone formation leading to hypercalcemia within days to weeks of the start of complete bed rest. It is marked by low PTH and serum calcitriol levels.
Lithium administration may cause mild hypercalcemia that results from interference with calcium sensing by the CaSR. The CaSR also binds lithium, which acts as an antagonist. Hypercalcemia is generally mild, clinically insignificant, and resolves with discontinuation of the drug. In some cases it persists and may be associated with clinical signs and symptoms. Pheochromocytoma, primary adrenal insufficiency, and familial hypocalciuric hypercalcemia (FHH) are additional rare causes of hypercalcemia. Pheochromocytoma may produce hypercalcemia via its association with MEN IIa or by the production of PTHrP. Catecholamines are also known to increase bone resorption. FHH is inherited in an autosomal dominant fashion. The mutation occurs in the CaSR, and results in a receptor that has decreased calcium affinity. As a result, elevated calcium concentrations are required to suppress PTH. It presents with mild hypercalcemia at a young age, decreased urinary calcium excretion, and a high normal or slightly elevated PTH concentration. Notably signs or symptoms of hypercalcemia are often absent. FHH is important because it can be misdiagnosed as PHPT and result in unnecessary parathyroid surgery. Patients with FHH often do not have clinical sequelae of excessive PTH activity such as hyperparathyroid bone disease or mental status changes. The presence of hypercalcemia in family members, a lack of previously normal serum calcium measurements, and low urinary calcium suggest FHH. In one study, 25% of patients that underwent unsuccessful parathyroid surgery were diagnosed with FHH. Some authors advocate using fractional excretion (FE) of calcium to distinguish FHH from PHPT with values below 1% suggestive of FHH. This is not recommended, however, given that 25% of patients with PHPT have a fractional calcium excretion below 1%.
KEY POINTS
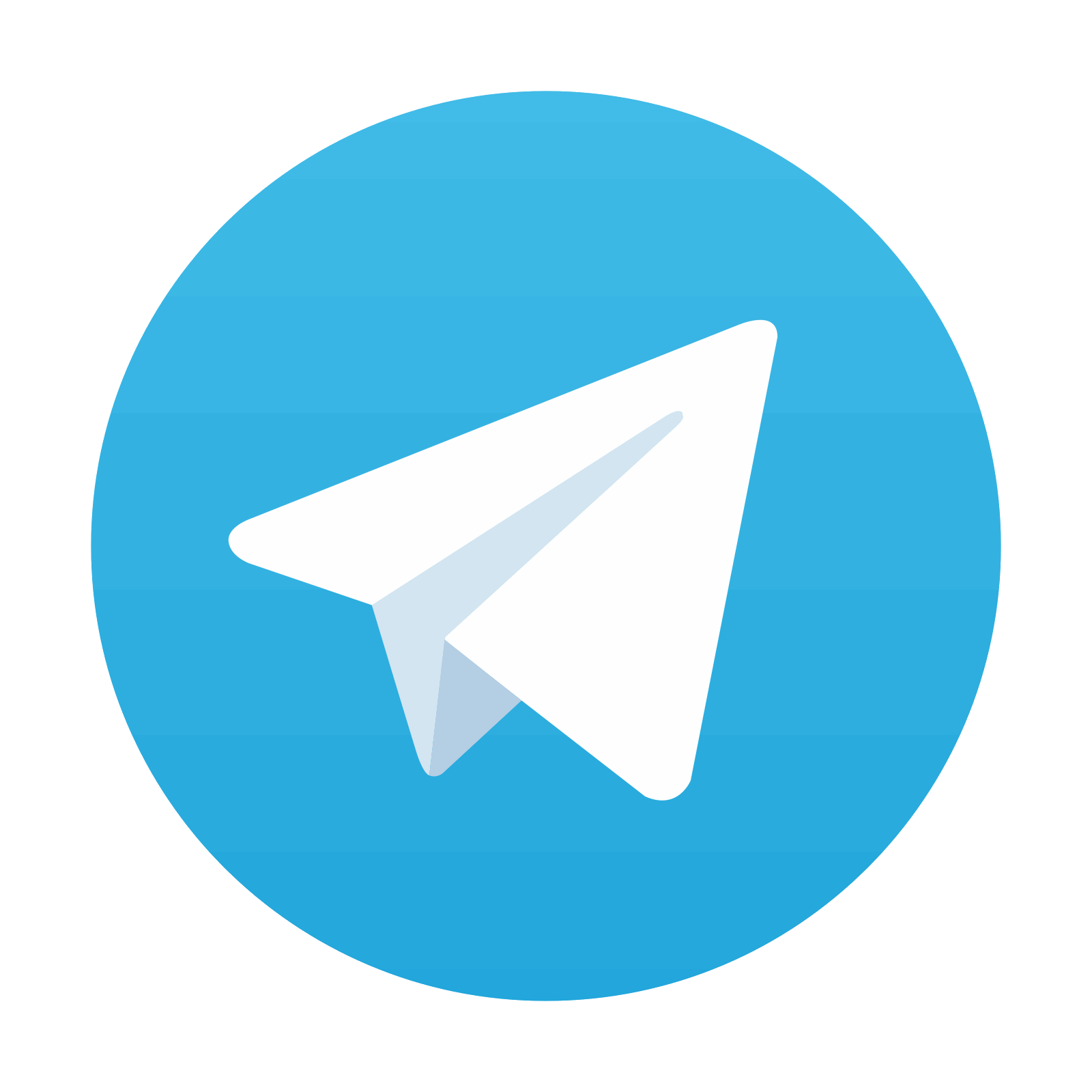
Stay updated, free articles. Join our Telegram channel

Full access? Get Clinical Tree
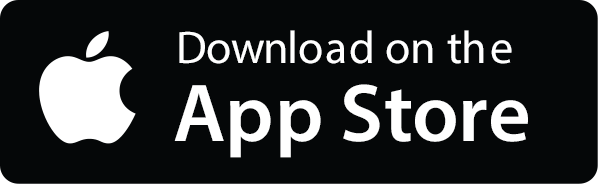
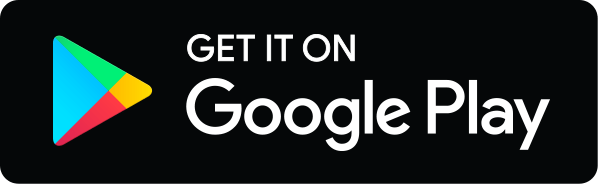