Diagnostic Angiography and Therapeutic Endovascular Intervention of the Renal Circulation
Alex Perino
Rajan Gupta
Ivan P. Casserly
The last decade has seen a dramatic paradigm shift in the diagnostic evaluation of pathology of the renal arterial and venous circulations. Noninvasive angiographic techniques, notably computed tomography (CT) and magnetic resonance (MR) angiography, have advanced to the degree that the diagnostic accuracy of these noninvasive imaging modalities rivals that of invasive angiography.1,2 In fact, in the renal venous circulation, these modalities are typically superior to invasive angiography. Hence, invasive angiography of the renal circulation is now generally performed to confirm the findings of noninvasive studies of the renal artery for the purpose of vascular intervention. The result is a more targeted and individualized approach to angiography that answers a clinical question or helps direct an endovascular therapy.
In contemporary practice, the majority of pathologies involving the renal circulation that require intervention are treated using an endovascular as opposed to a surgical approach. The majority of procedures are performed in the renal artery, with the primary pathologies being treated including atherosclerosis and fibromuscular dysplasia (FMD). Novel endovascular treatments are also emerging for the treatment of resistant hypertension (HTN). Renal venous interventions are rare.
This chapter outlines the angiographic anatomy of the renal arterial and venous circulations, describes the technique of renal artery angiography, and provides an overview of the most commonly performed interventions in the renal circulation.
ANGIOGRAPHIC ANATOMY
Renal Artery
In most individuals, a single right and left renal artery arises from the abdominal aorta at the level of the L2 vertebra (Fig. 12.1). Although both renal arteries arise at a similar level from the abdominal aorta, the right renal artery typically has an anterior origin, whereas the left renal artery has a posterior origin (Fig. 12.2).3 In addition, owing to the inferior location of the right kidney, the right renal artery has a downward course, whereas the left renal artery has a more horizontal course (Fig. 12.1B). Each renal artery divides at the hilum of the kidney into a posterior branch that runs posterior to the renal pelvis and supplies the majority of the posterior portion of the kidney and an anterior branch that typically divides into four segmental branches (apical, upper, middle, and lower) (Fig. 12.3).4,5,6 The upper and lower poles of the kidney are supplied by the apical and lower anterior segmental branches, with the upper and middle segmental branches supplying the remainder of the anterior surface of the kidney. Each segmental branch divides into lobar and subsequently into interlobar branches that course between the renal pyramids. At the corticomedullary junction, each interlobar artery divides into two arcuate arteries that terminate in interlobular arteries, which supply the inflow to the afferent arterioles in the glomeruli.
Variation in number of renal arteries (Fig. 12.4): Multiple renal arteries are very common, with 30% of patients having multiple renal arteries to a single kidney, and 10% of patients with multiple renal arteries to both kidneys. Some authors divide these “extra” renal arteries into two groups based on the course of the artery: accessory arteries course along the route of the main renal artery and enter the kidney at the hilum, whereas aberrant renal arteries have a distinct course to the main renal artery and enter the kidney from the renal capsule away from the hilum. Accessory and aberrant renal arteries typically arise from the lower thoracic and abdominal aorta, or iliac arteries. Less common sites of origin include the lumbar or mesenteric vessels. Accessory or aberrant renal arteries most commonly supply the upper or lower poles of the kidney.
Variation in branching pattern (Fig. 12.5): Prehilar branching of the main renal artery is seen in approximately 12% of individuals. This anatomic variant may complicate efforts to provide embolic protection during interventional procedures at the ostium of the main renal artery.
![]() FIGURE 12.1 A,B: Abdominal aortograms from two separate patients showing origin of renal arteries from L2 level of abdominal aorta. Typical inferior course of right renal artery shown in Figure 12.1B. White arrow, right renal artery; black arrow, left renal artery. |
Renal Vein
The venous drainage of each kidney generally parallels the arterial pattern. A single renal vein drains blood from each kidney in most individuals (Fig. 12.6). The renal vein lies anterior to the renal artery at the hilum and drains to the inferior vena cava (IVC), which is located to the right of the abdominal aorta. As a result, the length of the left renal vein is significantly longer than the right renal vein (6-10 cm versus 2-4 cm). In addition, the left renal vein must course between the superior mesenteric artery and the abdominal aorta in order to reach the IVC (Fig. 12.6). In contrast to the right renal vein, the left renal vein receives several tributaries, including the adrenal, gonadal, and lumbar veins.
Anomalous anatomy of the renal veins is common. The most common variations include:
Variation in number of renal veins: Multiple right renal veins have been reported in 15% to 30% of individuals.
Variation in course of renal veins: This variation is typically seen in the left renal vein. The most common variation is a circumaortic course, where the left renal vein divides into anterior and posterior limbs that encircle the abdominal aorta, and is estimated to occur in ˜15% of individuals. Less commonly, the left renal vein has a completely retroaortic course, which is seen in 3% of individuals.
INVASIVE ANGIOGRAPHY OF THE RENAL CIRCULATION
Prior to reviewing the specifics of the technique of renal artery angiography, it is worth discussing the essential elements that are common to all renal angiographic procedures including the use of contrast agents and contemporary imaging systems for peripheral angiography.
Contrast Agents
The contrast agent most commonly used in current practice for renal artery angiography is Iodixanol (Visipaque, GE Healthcare). This agent has an osmolality that is similar to that of blood
(i.e., 300 mOsm per kg H2O), which differentiates it from low (620-790 mOsm per kg H2O) and high (> 1,700 mOsm per kg H2O) osmolar agents and results in significantly improved tolerance by patients.7 Although Iodixanol has a lower iodine concentration compared to low and high osmolar contrast agents, the impact on the imaging characteristics achieved with this agent is negligible, especially when contemporary imaging equipment is utilized. Iodixanol has the highest viscosity of all the available contrast agents which can make intravascular injections difficult, especially when performing hand injections using small caliber catheters. This impact can be minimized by warming the agent to 37°C prior to injection, which is standard practice in most angiographic laboratories, and using automated systems for contrast injection
(e.g., ACIST CVi, ACIST Medical Systems, Eden Prairie, MN; and Avanta, MEDRAD Inc., Warrendale, PA).
(i.e., 300 mOsm per kg H2O), which differentiates it from low (620-790 mOsm per kg H2O) and high (> 1,700 mOsm per kg H2O) osmolar agents and results in significantly improved tolerance by patients.7 Although Iodixanol has a lower iodine concentration compared to low and high osmolar contrast agents, the impact on the imaging characteristics achieved with this agent is negligible, especially when contemporary imaging equipment is utilized. Iodixanol has the highest viscosity of all the available contrast agents which can make intravascular injections difficult, especially when performing hand injections using small caliber catheters. This impact can be minimized by warming the agent to 37°C prior to injection, which is standard practice in most angiographic laboratories, and using automated systems for contrast injection
(e.g., ACIST CVi, ACIST Medical Systems, Eden Prairie, MN; and Avanta, MEDRAD Inc., Warrendale, PA).
![]() FIGURE 12.4 Multiple renal arteries. A: Abdominal aortogram from patient with bilateral accessory renal arteries. Black arrow, right lower accessory renal artery; white arrow, left upper accessory renal artery. B: Abdominal aortogram from patient with aberrant left renal artery arising from lower segment of abdominal aorta just above the aortoiliac bifurcation (black arrow). White arrowhead, occluded right renal artery; black arrowhead, severe stenosis at origin of left main renal artery. C: Abdominal aortogram from patient with aberrant right renal artery arising from lower segment of abdominal aorta just above the aortoiliac bifurcation (white arrow). Interrupted white arrow, right main renal artery. D: Selective angiogram of aberrant right renal artery from patient shown in Figure 12.4C. |
![]() FIGURE 12.5 A,B: Angiograms from two patients showing prepolar branching of the left main renal artery (inferior division of main renal artery indicated by white arrow, stenosis at origin of inferior division of main renal artery in Fig. 12.5A indicated by arrowhead, extrarenal branch of left main renal artery in Fig. 12.5A indicated by black arrow). |
Alternate contrast agents have been used for patients with severe renal insufficiency in whom the risk of contrast-induced nephropathy is significant and in those with an allergy to iodinated contrast agents, Carbon dioxide (CO2) has been used as an intra-arterial contrast agent since the early 1970s. However, it wasn’t until the introduction of digital subtraction angiography (DSA) (see later text) in 1980 that CO2 angiography became a useful diagnostic tool (Fig. 12.7).8 In general, CO2 angiography is well suited to imaging of large caliber vessels below the level of the diaphragm such as the abdominal aorta, aortoiliac segment, and proximal segments of major branches from the abdominal aorta (including renal arteries).9 Imaging of smaller caliber vessels is problematic, and images must be acquired at a high frame rate to ensure acquisition of the image before dissipation of the gas.
The use of gadolinium-based contrast agents for intra-arterial angiography has largely been abandoned in current practice due to the emergence of nephrogenic systemic fibrosis as a potential complication of exposure to this agent in patients with significant renal insufficiency.10,11,12 Similar to CO2 angiography, angiography with gadolinium provides reasonable diagnostic data in larger caliber vessels and requires a high frame rate for image acquisition.
Imaging Systems
The basic image equipment requirements for acquisition of angiographic images of the peripheral circulation (including renal circulation) includes an image chain that consists of a generator and X-ray tube located beneath the table on which the patient is positioned, and an image intensifier, optical distributor, and camera located above the patient (Fig. 12.8). Real-time presentation of the angiographic image is generated on a monitor located within view of the operator. Over the last decade, a number of advancements in the imaging chain, most notably the introduction of flat panel technology in image intensifiers and the digitization of image processing, have enhanced the quality of the images generated.
In addition to this basic imaging chain, the technology of digital subtraction angiography (DSA) is fundamental to imaging of the cardiovascular system. Although this technique was developed in the 1970s, continued refinement of the technology used to generate DSA imaging has occurred providing additional improvements in image quality and functionality. In essence, the technique of DSA involves the acquisition of X-ray images before the administration of the contrast agent and during opacification of the vessel of interest following the intra-arterial injection of contrast proximal to the vessel segment of interest.9,13 Processing of the images is then performed that involves subtracting the background X-ray image (referred to as the “mask” image) that displays bony and soft tissue structures from the X-ray image during vessel opacification, resulting in an image that displays vessel opacification alone (Fig. 12.9). The technique requires that no significant motion (e.g., patient motion, respiratory motion, peristalsis) occurs between the acquisition of the background image and vessel opacification. DSA represents the gold standard for imaging of the vascular tree, including the renal circulation.
Renal Artery Angiography Technique
With the advances in CT and MR angiography, diagnostic angiography of the renal arteries is typically performed to confirm the diagnostic findings of these noninvasive anatomic imaging modalities prior to planned interventional procedures.1,2 However, in clinical practice, there remains a subset of patients with significant renal insufficiency who require
invasive angiography for diagnostic purposes due to thresholds of serum creatinine (Cr) and eGFR (i.e., Cr > 1.5 mg per dl, eGRF <60 mL per min) utilized by most radiology departments for exposure of patients to iodinated contrast and gadolinium-based contrast agents that are used for CT and MR angiography, respectively.
invasive angiography for diagnostic purposes due to thresholds of serum creatinine (Cr) and eGFR (i.e., Cr > 1.5 mg per dl, eGRF <60 mL per min) utilized by most radiology departments for exposure of patients to iodinated contrast and gadolinium-based contrast agents that are used for CT and MR angiography, respectively.
![]() FIGURE 12.6 Venography of right and left renal veins. A: Right renal vein (black arrow). Hepatic vein indicated by white arrow. IVC, inferior vena cava. B: Left renal vein. |
A necessary preamble to renal artery angiography is the establishment of arterial access. In most circumstances, this is obtained in the right or left common femoral artery (CFA). Less commonly, the brachial or radial artery may be used. The advantages and disadvantages to these various access sites are outlined in Table 12.1.
Depending on the clinical situation (e.g., preprocedural imaging, baseline renal function, procedural indication), abdominal aortography may be performed prior to selective renal artery angiography. This is achieved by placing a flush catheter with multiple side holes at the level of the T12 vertebra and injecting contrast at 20 mL per sec for a total of 40 mL (Fig. 12.10). The abdominal aortogram provides information about the location of the renal artery ostia and the condition of the abdominal aorta (e.g., presence of atherosclerosis) and its major branches.
TABLE 12.1 Advantages and Disadvantages of Various Arterial Access Sites for Renal Artery Angiography and Intervention | ||||||||||||
---|---|---|---|---|---|---|---|---|---|---|---|---|
|
Selective angiography of the renal arteries is performed by using catheters with preformed shapes to facilitate selective engagement of the renal artery ostia (Fig. 12.11). A variety of catheters are available for this purpose. The choice of a specific catheter is determined by the anatomy of the abdominal aorta, the location of the renal artery ostium, the course of the proximal segment of the renal artery, the arterial access site used, and the experience of the operator. In the authors’ practice, the most commonly used catheters when approaching the renal artery from the CFA access site are the
Sos, Simmons, Cobra, and JR4 catheters. When approaching from the upper extremity access sites, the most commonly used catheter is the multipurpose catheter (Fig. 12.12).
Sos, Simmons, Cobra, and JR4 catheters. When approaching from the upper extremity access sites, the most commonly used catheter is the multipurpose catheter (Fig. 12.12).
Images are acquired using DSA. Typically, adequate imaging of the renal arteries is achieved by injecting contrast at 4 mL per sec for total of 8 mL through the catheter. Due to the anterior and posterior location of the right and left renal ostia, respectively, the ostia and proximal segments of both renal arteries are best visualized with the image intensifier in the left anterior oblique projection.3 Delayed imaging is generally performed to allow visualization of the nephrogram, which provides an estimate of the renal size and the contour of the kidney (Fig. 12.13). In certain clinical situations (e.g., aneurysmal disease), imaging in multiple planes or rotational angiography may be required to elucidate the precise relationship of the pathology to branch points, which can influence treatment decisions.
RENAL ARTERY INTERVENTION
In current practice, the majority of renal artery intervention is performed for the treatment of atherosclerotic disease, and to a lesser extent FMD, and will thus constitute the major
focus of this section. Less commonly treated pathologies are briefly discussed. Finally, the emerging therapy of renal artery denervation is outlined as there is considerable excitement about the potential of this therapy in the treatment of an array of medical renal disorders.
focus of this section. Less commonly treated pathologies are briefly discussed. Finally, the emerging therapy of renal artery denervation is outlined as there is considerable excitement about the potential of this therapy in the treatment of an array of medical renal disorders.
Atherosclerotic Disease
Atherosclerotic disease of the renal arteries is a common entity, particularly in patients with HTN (prevalence of 1% to 5%) and in those with documented atherosclerotic disease in other vascular territories (prevalence of 30% to 40%). Roughly 90% of all cases of renal artery stenosis (RAS) are due to atherosclerotic disease, which is characterized by a typical location at the renal artery ostium or proximal segment of the main renal artery (Fig. 12.14). This is due to the fact that renal artery atherosclerosis typically reflects an extension of atherosclerosis involving the abdominal aorta (Fig. 12.15).
![]() FIGURE 12.12 Selective angiography of the right and left renal arteries using upper extremity access and multipurpose catheter (white arrows). |
The most challenging component of renal artery intervention for atherosclerotic RAS is case selection, rather than the execution of the intervention itself. Ischemic nephropathy is a complex entity, and considerable controversy exists over the appropriateness of renal revascularization for this disease.14,15,16 Unfortunately, randomized trials comparing medical therapy with renal artery intervention have not proved to be particularly helpful in defining the patient populations that benefit from revascularization. In the absence of such guidance, the authors attempt to identify those patients who are most likely to benefit from revascularization for atherosclerotic RAS by asking two fundamental questions:
Is the patient’s clinical condition being caused by atherosclerotic RAS?
If so, is there a reasonable chance that revascularization will have a meaningful clinical benefit?
The answer to the first question is typically answered by the nephrologist taking care of the patient and is provided by matching the patient’s clinical presentation (e.g., resistant HTN, acute renal failure with initiation of angiotensin converting enzyme inhibitor, flash pulmonary edema in the setting of uncontrolled HTN, acute or subacute renal failure) with the anatomy of the RAS (unilateral vs. bilateral, severity of stenosis) and anatomic and functional data regarding the parenchymal function and perfusion of each kidney.
The second question is typically arrived at following a discussion between the nephrologist and interventionalist. In general, it is felt that the more severe the RAS, and the less severe the parenchymal disease in the affected kidney, the more likely that revascularization of the main renal artery is likely to have a clinical impact on the patient. The variables that are used to help make this determination and their impact on clinical outcomes following renal revascularization are summarized in Table 12.2. It should be accepted that these variables are most useful when the goal of intervention is to preserve renal function. When the goal of intervention is to improve the control of HTN,
decision-making is more complicated, as it can be very difficult to predict the effect of revascularization on renin output from a severely ischemic kidney that may have little parenchymal function.
decision-making is more complicated, as it can be very difficult to predict the effect of revascularization on renin output from a severely ischemic kidney that may have little parenchymal function.
Execution of Intervention for Atherosclerotic RAS
Arterial access is obtained in the common femoral artery (CFA) or upper extremity artery. A guide catheter is used to engage the ostium of the culprit renal artery (Fig. 12.16). Guide catheters are significantly stiffer than diagnostic catheters and have a greater potential for causing injury to the aorta or renal artery ostium. Because atherosclerotic disease of the renal artery is typically a manifestation of aortic atherosclerosis that encroaches on the renal artery, great care with manipulation of guide catheters in the abdominal aorta is warranted in order to prevent atheroembolism to the lower extremity, mesenteric, and/or renal arteries. A variety of techniques (e.g., no-touch technique) have been described that minimize this manipulation.17
Once the guide is engaged at the ostium of the renal artery, an interventional wire is advanced into one of the segmental vessels. In current practice, 0.014-inch wires with atraumatic coil tips are typically used.18 The stenosis is dilated with an angioplasty balloon with a balloon-artery ratio of ˜0.8. Regardless of the initial result with angioplasty, the lesion is then typically stented using a balloon-expandable stent (Fig. 12.17). This is based on two considerations: (1) stenting achieves a highly predictable acute result by overcoming the issue of elastic recoil and treatment of any dissections that can lead to subsequent abrupt vessel occlusion, and (2) stenting reduces the high rate of restenosis that is seen with angioplasty alone.19 Most of these stents are composed of stainless steel, but a small minority are of composed of cobalt-chromium. Balloon-expandable stents are
used in this location because of their high radial strength, which is an important consideration at the ostial location. Positioning the stent such that the ostium of the renal artery is covered by stent is a critical component of renal artery stenting. This will generally result in 1 to 2 mm of the stent projecting out into the abdominal aorta. Failure to achieve this objective is associated with high rates of restenosis. The stent is typically postdilated using a noncompliant balloon with a balloon-artery ratio of ˜ 1.0.
used in this location because of their high radial strength, which is an important consideration at the ostial location. Positioning the stent such that the ostium of the renal artery is covered by stent is a critical component of renal artery stenting. This will generally result in 1 to 2 mm of the stent projecting out into the abdominal aorta. Failure to achieve this objective is associated with high rates of restenosis. The stent is typically postdilated using a noncompliant balloon with a balloon-artery ratio of ˜ 1.0.
The use of embolic protection during renal artery intervention is inconsistent in current practice. In theory, these devices should help prevent atheroembolism to the kidney as a result of angioplasty and stenting, and thus improve the safety of renal artery intervention by minimizing any adverse impact on renal function. Filter-type embolic protection devices have been used with good clinical success.20,21,22 A broad range of these devices are available for use, based on U.S. Food and Drug Administration (FDA)approved indications for use in saphenous vein graft intervention in the coronary circulation,23,24,25
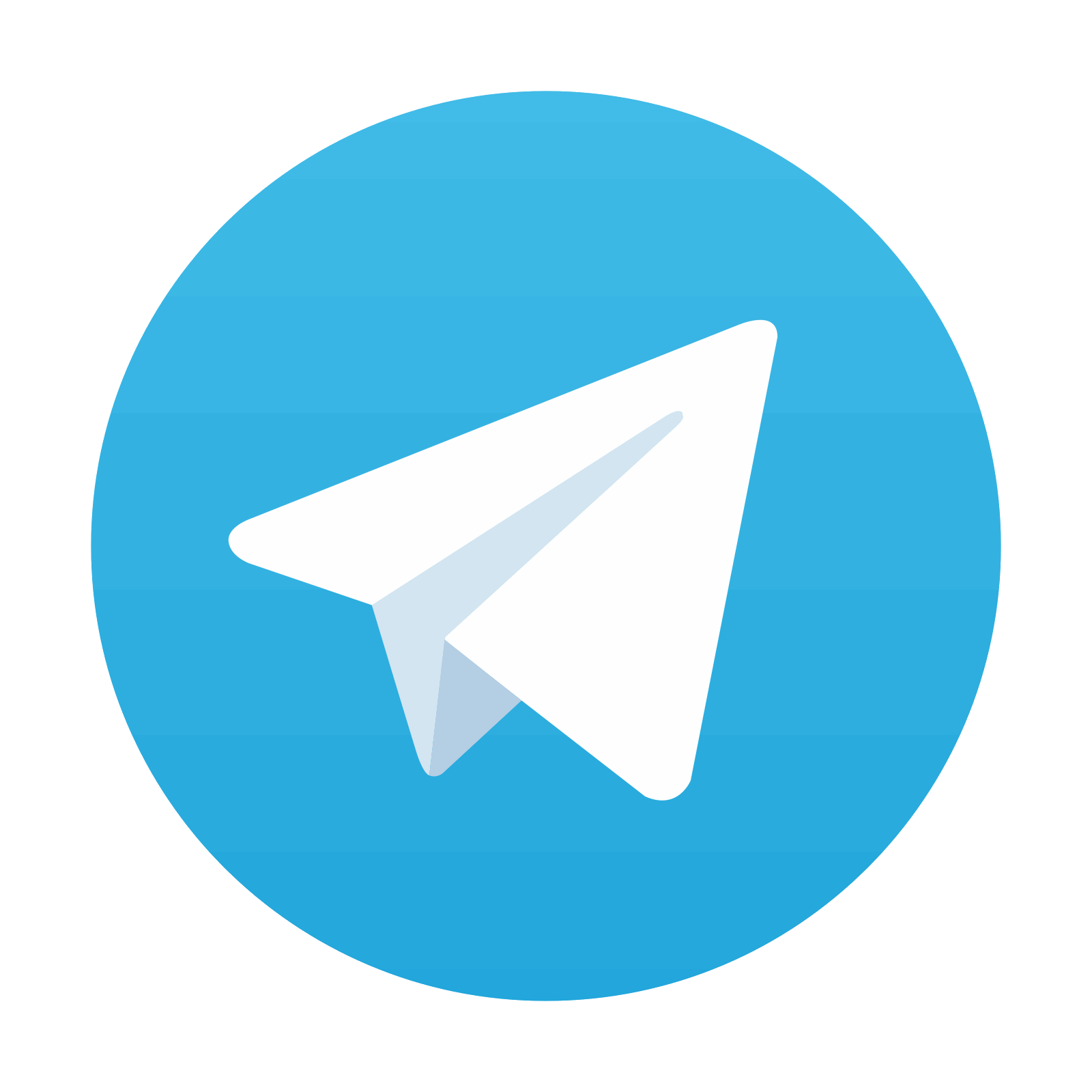
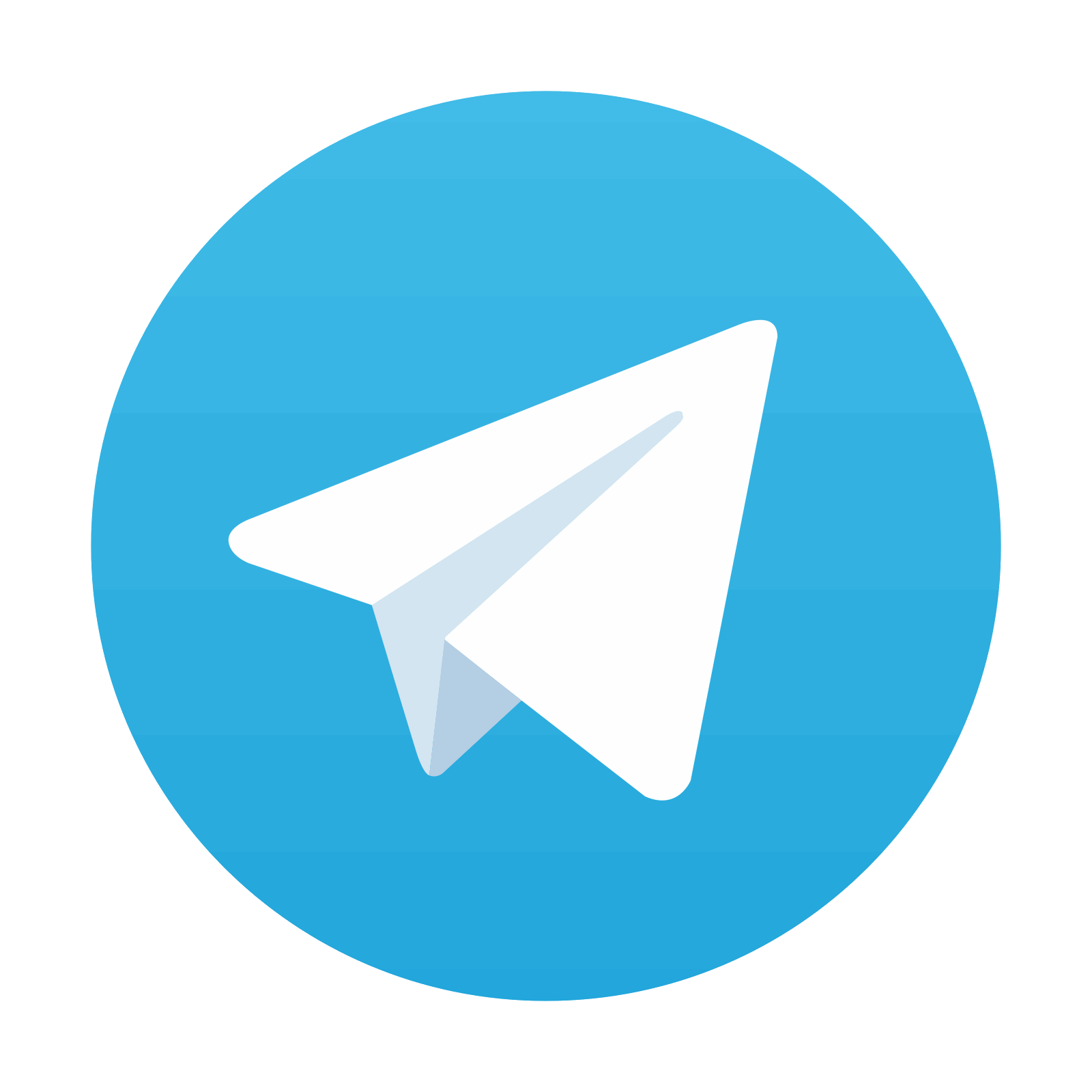
Stay updated, free articles. Join our Telegram channel

Full access? Get Clinical Tree
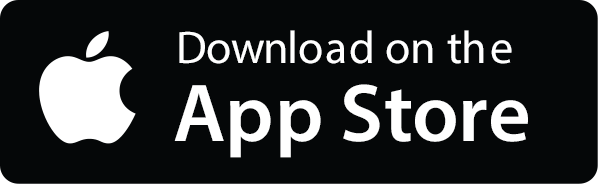
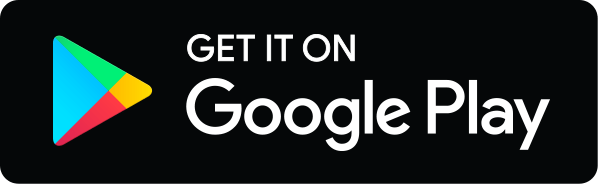
