EPIDEMIOLOGY
For some time, diabetes mellitus (DM) has been known to be the leading cause of chronic kidney disease (CKD) in the world. Diabetic kidney disease (DKD) is a well-known complication hastened by uncontrolled diabetes, and unfortunately, it is a condition that affects millions around the world. Estimations in the United States demonstrate that diabetes is responsible for approximately 50% of all end-stage kidney disease (ESKD) in the developed world (1). Although the number of ESKD patients due to diabetes continues to rise, with nearly 50,000 new cases in 2011, the incidence has seemed to have plateaued over the last decade (2). This plateau may be attributed, in part, to an increase in education and awareness, easy accessibility to supportive medications, and due diligence in screening on the part of primary care providers. Despite this trend, the prevalence of DKD is increasing in the United States with an increase from 7.4% to 9.6% in adults over the age of 20 years from 1998 to 2006 (3). Differences remain among socioeconomic classes and ethnic subgroups such as African Americans, Hispanics, and Native Americans having a higher rate of ESKD (4). This is likely in response to the increase in incidence of type 2 diabetes and obesity that is plaguing younger generations allowing the expansion of diabetes at younger ages (4).
Current advances in renal replacement therapy, especially dialytic modalities, have slowly decreased mortality rates over the past years. According the United States Renal Data System, mortality rates have declined 26% since 1985 and 21% since 2000 in patients receiving hemodialysis (1). Additionally, 52% and 61% of patients receiving hemodialysis and peritoneal dialysis, respectively, are still alive after 3 years of therapy. Despite these advances, the all-cause mortality rate still remains high in patients receiving dialysis, 6.5 to 7.9 times greater than in the general population (1). Moreover, diabetic dialysis patients have a worse survival than those with other ESKD etiologies with a survival of 34% at 5 years (2). It can be inferred that if this trend continues, then two probable paths will emerge, either the incidence will continue to increase proportionally with an increase in DKD and progression to ESKD or there will be a maintained plateau or even fall in ESKD prevalence with continued focus of prevention of DKD (3). That is why it is imperative that continued education, perseverance of screening, and appropriate pharmacotherapy be implemented.
IMPACT ON PATIENTS AND RESOURCES
DKD continues to have a large impact on patient morbidity and financial institutions. Kidney disease, notably albuminuria, and declining glomerular filtration rates are independent risk factors for cardiovascular disease and noncardiovascular mortality (4). These consequences are related to the micro- and macrovascular sequelae that arise from repeated insults of oxidative stresses and inflammatory mediators as a result of chronic diabetes. Furthermore, ESKD confers a risk of cardiovascular disease about 40 to 50 times greater than the general population (5).
The financial burden of ESKD is high and has been steadily increasing. In 2012, Medicare’s expenses for ESKD had risen 3.2% from the previous year, the highest in the last 20 years, accounting for a total of $28.6 billion and 5.6% of the total Medicare expenditure (1). These expenses cover 5,525,481 ESKD patients in the prevalent Medicare population, along with 111,418 non-Medicare patients, with the latter adding an additional estimated $14.93 billion (1). The total fee-for-service in the hemodialysis population in per person per year (PPPY) were $87,945 in 2011 and for peritoneal dialysis patients $71,630 PPPY (1). These costs have led to significant economic strain on the U.S. health care system especially in light of budget cuts in recent years.
MORTALITY IN PATIENTS WITH DIABETES AND END-STAGE KIDNEY DISEASE
Although there is an increased risk of mortality related to end-stage microvascular events such as progressive nephropathy and retinopathy, cardiovascular events have escalated rates of morbidity and mortality in patients with DM (6). Multiple studies have been conducted to evaluate cardiovascular and all-cause mortality in diabetic patients with comparison between intensive versus conventional/standard glycemic control therapy.
In patients with type 1 DM, Diabetes Control and Complications Trial (DCCT) demonstrated that intensive glycemic control [targeting a glycated hemoglobin (HbA1c) level of less than 7% vs. 9%] delayed the onset of microvascular complications and nephropathy with a 34% and 56% reduction in microalbuminuria and macroalbuminuria over a mean follow-up period of 6.5 years (7). In Epidemiology of Diabetes Interventions and Complications (EDIC), a follow-up study was completed to evaluate cardiovascular outcomes in this population. DCCT could not assess such results because of the younger population demography (ages 13 to 39 years). EDIC monitored patients for an 11-year period and found that intensive glycemic control lowered the risk of a cardiovascular event by 42% and by 57% for a severe cardiovascular event. It was extrapolated that those patients with cardiovascular events also had some level of retinopathy or nephropathy concurrently. Thus, EDIC demonstrated that intensive glycemic control contributed to a decrease in end organ damage, including nephropathy (7).
There are four significant trials that examined cardiovascular events in patients with type 2 DM, in comparing intensive versus conventional glucose control. In the United Kingdom Prospective Diabetes Study (UKPDS), intensive glycemic control (fasting glucose <108 mg/dL, median HbA1c 7.0%) led to a 25% reduction in microvascular complications (mainly retinopathy requiring photocoagulation) in patients with newly diagnosed with type 2 DM. There was also a reduction in myocardial infarctions and all-cause mortality that was ascertained during a 10-year follow-up period (8). This amount of extended follow-up could not be replicated in the other studies analyzing similar variables (8).
Afterward, in the Action to Control Cardiovascular Risk in Diabetes (ACCORD) trial, the initial goal aimed to see if the results from DCCT crossed over to patients with type 2 DM. In ACCORD trial, the intensive glycemic control group had an average HbA1c <6%, while the standard glycemic control group had an average HbA1c of 7% to 7.9%. Diverging from similar studies, ACCORD trial found that patients in the intensive therapy group had increased mortality, with no improvement in cardiovascular risk reduction. The original study was prematurely halted due to this statistically significant and surprising finding. A follow-up of the study illustrated a reduction in nonfatal cardiovascular events (9).
Concurrently, in the Action in Diabetes and Vascular Disease: Preterax and Diamicron Modified Release Controlled Evaluation (ADVANCE) trial, patients with onset of DM after age 30 years were examined. There was a 23% reduction of microvascular events such as nephropathy in the intensive glycemic control (HbA1c less than or equal to 6.5%) study group compared to the standard one at a mean 5-year follow-up period. In contrast to the ACCORD trial, there was not an increased risk of mortality in the intensive glycemic control study group. An additional 5-year follow-up demonstrated similar findings as well (9).
In Glucose Control and Vascular Complications in Veterans with Type 2 Diabetes [Veterans Affairs Diabetes Trial (VADT)], a similar group of patients was found to have no significant improvement in cardiovascular event rate after randomization to intensive versus standard glycemic control study groups. After a 5.6-year follow-up, the constricted group (average HbA1c 6.5%) had a decreased occurrence of microvascular events, in mainly the incidence of nephropathy. However, the number of deaths did not significantly differ between the two study groups (10).
In review of these trials, it is concluded that long-term intensive glucose control (HbA1c around 7%) corresponds to the delay in developing kidney disease secondary to DM. The question of whether CKD itself presents a risk factor for cardiovascular mortality is not addressed in these studies, as most of the selected patients did not have preexisting CKD from another known etiology. Unfortunately, there have been no randomized controlled trials assessing mortality in patients with diabetes and ESKD. Therefore, the clinician’s goal is to aim for optimal glycemic control in patients, with the hope to prevent DKD or delay the progression.
GLUCOSE HOMEOSTASIS IN RENAL DYSFUNCTION
Insulin utilization in muscle and adipose tissue is the rate-limiting step that sets into action specific transporter proteins to translocate glucose into cells (11). These actions are precipitated by the phosphorylation of the insulin receptor substrate 1 (IRS-1), thereby leading to the activation of other downstream proteins, namely, protein kinase C, glycogen synthase, and endothelial nitric oxide synthase, and ultimately leading to the uptake of glucose (11).
In light of renal dysfunction, glucose homeostasis experiences altered functions, with episodes of sustained or periodic hyper- or hypoglycemia (12). Abnormal glucose control has been a known manifestation of renal dysfunction, notably ESKD, for many decades (13). Although diabetes plays a key role, patients with ESKD from other etiologies have also been noted to experience altered glucose metabolism leading to a mild fasting hyperglycemia and episodes of spontaneous hypoglycemia (14).
Insulin Clearance
One of the key abnormal factors that surround glucose homeostasis in ESKD is the clearance of insulin by the kidneys. Insulin clearance is mediated by its uptake and degradation in the peritubular and endothelial cells of the kidney (13). Glomerular filtration has little effect on insulin clearance until the filtration rate drops below 40 mL/min. The uptake and degradation of insulin is increased as the filtration rate decreased, until the filtration rate reaches 15 to 20 mL/min, and then the rate of insulin clearance declines proportionally (13). The rate of insulin uptake and degradation is also affected by other factors that lead to a prolonged half-life of the compound. As kidney function begins to decline, other toxins begin to accumulate and sites of nonrenal insulin degradation such as muscle and liver begin to experience impaired function contributing to a longer circulating time (13).
Hyperglycemia
In the advanced stages of CKD, hyperglycemia and glucose intolerance continue to progress until a decline in insulin secretion and insulin resistance is observed (13). Hyperglycemia, as a manifestation of insulin resistance and glucose intolerance, has also been observed in nondiabetic patients. This suggests that one of the presenting features of ESKD is insulin dysregulation and that it possibly plays a role in the alteration of glucose regulation (13). Studies have looked at insulin receptor sites in uremic patients and through the investigation of muscle biopsies rendered normal insulin binding and activation of downstream proteins (15). This suggests that in uremic patients, the mechanisms are not related to alterations in the protein complexes themselves but may be related to an outside influence. One suggestion is the possibility of oxidative and nonoxidative pathways augmenting insulin resistance (16). Although the exact pathogenesis is not known, it is speculated that accumulation of uremic toxins, such as pseudouridine and asymmetric dimethyl arginine (ADMA), may play a role in insulin resistance, as improvements in sensitivity has been observed when patients began dialysis (17). Additionally, urea, which was never given weight in terms of toxicity, has been shown in animal models to induce reactive oxygen species and lead to insulin resistance (18). Long-standing metabolic acidosis may also play a role in insulin resistance as it has been associated with progressive inflammation and protein-energy malnutrition due to its influence on catabolic effects at the cellular level (19). Administration of bicarbonate has been shown to mitigate its effects with improved insulin sensitivity and insulin secretion in uremic patients (20).
Chronic inflammation, as in ESKD, also leads to impaired glucose uptake due to the release of inflammatory mediators such as interleukin 6 (IL-6), C-reactive protein (CRP), interleukin 1 beta (IL-1-β), and tumor necrosis factor alpha (TNF-α) (21). TNF-α appears to play a major role in insulin resistance through multiple mechanisms. Downstream effects of TNF-α mediation have been shown to induce the phosphorylation of muscle cell IRS-1 that is linked to two main pathways: phosphatidylinositol 3-kinase and protein kinase B (18). These two pathways are metabolically responsible for the majority of glucose transport. With the phosphorylation of IRS-1, downstream effects are activated in the insulin receptor that ultimately leads to a failure of the signaling pathway and disrupts glucose transporter type 4 (GLUT4) translocation, thereby interfering with glucose uptake (22). This was observed after the infusion of TNF-α in healthy humans, where downstream effects lead to the dysfunction in the GLUT4 transporter (23). TNF-α is also responsible for the induction of lipolysis and accumulation of free fatty acids that contribute to insulin resistance through the induction of additional inflammatory markers in the hepatic system (24). IL-6 contributes to insulin resistance by the induction of suppressors of cytokine signaling (SOCS) proteins responsible for feedback regulation (25). These proteins directly affect the insulin receptor and IRS-1 resulting in an inhibition of glucose translocation into muscle and adipose tissue (25).
Other factors have also emerged that lead to the suggestion that alterations in metabolism, primarily adipose tissue, have played a role in insulin resistance. Adipose tissue, accounting for less than 2% of glucose load usage, is now acknowledged as an active organ that secretes numerous enzymes, hormones, and cytokines (26). The tissue is rich in macrophages and adipocytes, and it is speculated that these cells release TNF-α and IL-6 among other cytokines, commonly referred to as adipokines (26). Data suggests that in uremic patient’s adipose tissue, primarily visceral fat, is an important source of these inflammatory molecules (27). The progressive reduction in renal function contributes to the imbalance of these adipokines, along with decreased clearance of proinflammatory cytokines leading to a state of chronic inflammation (26).
Many adipokines play a role in the augmentation of insulin sensitivity. Leptin is a small peptide responsible for energy expenditure and the feeling of satiety that is commonly filtered by the kidney and degraded in the renal tubules (28). This peptide appears to have a correlation with CRP and has been suggested that elevation in its plasma concentration may be a major contributor in uremic cachexia (29). Interestingly, additional studies in animal models have proposed that hyperleptinemia may lead to increased expression of TGF-β and therefore contribute to the development of tubulointerstitial fibrosis and nephron destruction further decreasing renal clearance (30). Although chronic plasma elevations of leptin may contribute to worsening kidney disease, its association with insulin resistance is not quite understood. It has been observed that elevated plasma concentrations of leptin appear to be associated with elevated insulin levels leading to the speculation that resistance is present (31).
Adiponectin is another small adipokine peptide that is predominantly secreted by adipocytes. Unlike other cytokines, adiponectin has many protective biologic roles as it appears to have anti-inflammatory and antiatherogenic properties along with an insulin sensitizing effect by inducing GLUT4 (32). Its actions are primarily responsible for assisting in the production on nitric oxide in the endothelial cells, fatty acid oxidation, and inhibition of hepatic gluconeogenesis (33). Although adiponectin is a desirable peptide, lower levels are a result of chronic inflammation and have been implicated in increasing the risk for cardiovascular disease and the development of insulin resistance (34). Although lower concentrations are associated with resistance, patients undergoing hemodialysis were found to have exceptionally high levels of adiponectin (35). The elevation is likely related to the decrease in renal function; however, there is some minor evidence that there might be an upregulation in adiponectin synthesis in response to the uremic inflammatory state (36). Despite ESKD patients having elevations in adiponectin, it is currently unknown as to how this relates to insulin resistance.
Recent evidence has pointed to the role of vitamin D deficiency and secondary hyperparathyroidism in insulin resistance, suggesting that there is an inverse relationship between serum 25-hydroxyvitamin D and insulin resistance (13). One of the nonclassical effects of vitamin D is the effect on the islet cells in the pancreas and the stimulation of insulin secretion (37). Vitamin D repletion has been shown to increase insulin secretion and improve glucose tolerance in hemodialysis patients without changes in parathyroid hormone concentration (37).
Finally, a major contribution to hyperglycemia in patients undergoing dialysis is the use of larger glucose concentrations in peritoneal dialysis. Glucose concentrations in dialysate can range from 1,360 to 3,860 mg/dL and can contribute to as much as 10% to 30% of a patient’s total caloric intake (38).
Hypoglycemia
Alterations in renal physiology compounded with dialytic therapy have been observed to change the natural course of diabetes, leaving the patient with variability in glucose homeostasis (39). The uremic milieu has been thought to contribute to poor glucose tolerance and a decrease in insulin sensitivity leaving the patient with episodes of hyperglycemia (2). Despite these observations, spontaneous resolution of hyperglycemia, along with an impressive normalization of HbA1c, has been seen in patients undergoing dialysis, regardless of other treatment modalities (39). This phenomenon has been described as “burnt-out” diabetes, where in many diabetic patients undergoing dialysis, the occurrence of glucose normalization with spontaneous hypoglycemia results in the cessation of antidiabetic therapy in hopes of mitigating the potentially fatal episodes (40).
This condition appears to be multifactorial, with numerous factors contributing to glucose normalization. As progression to ESKD occurs, there is an observed reduction in the nephron mass (41). This reduction, likely related to nephron scarring and an attenuation of function, may lead to the reduced renal gluconeogenesis (42). Additionally, the uremic milieu asserts its deleterious effect on hepatic functions by disrupting the mechanisms of insulin clearance, thus contributing to an increase in the half-life of circulating insulin (13). The process of insulin clearance and degradation is affected once the filtration rate declines to below 15 to 20 m/min (39).
Along this thought, the decline in filtration rate leads to the accumulation of multiple uremic toxins (39). Guanidine solutes contribute to a large variety of compounds that make up the uremic milieu (43). Several have been implicated in vascular disease acceleration and have been identified as players in neuronal, cardiovascular, leukocyte, erythrocyte, and platelet dysfunction (43). Biguanides are frequently used to treat type 2 diabetes, and interestingly, their structure is composed of a simple marriage between two guanidine with the loss of an ammonia group (2). It has been suggested that the accumulation of these guanidine groups may contribute to the stabilization of blood glucose and the normalization of HbA1c (2).
These metabolic instabilities have been observed to extend beyond the context of glucose homeostasis. Physical fitness has long been advised a method of glucose control (13). Observations have been made with dialysis patients who participate in a long-term fitness program resulting in improved glucose tolerance, normalizations of glycemic, and exercise capacity (44).
Existing evidence has shown that uremia contributes to muscle protein catabolism and likely other metabolic defects (12). Nondiabetic and nonobese patients undergoing dialysis have also been observed to undergo similar muscle protein catabolism (12). This breakdown has been implicated through the ubiquitin–proteasome pathway due to the suppression of phosphatidylinositol-3 kinase (39).
Glucose utilization may also be improved by the influence of diabetes in the mechanics of gut motility. Diabetic gastroparesis leads to slowing of gut motility leading to early satiety and contributes to poor oral intake and malnutrition (13). Along that thought, distention of the abdominal cavity caused by peritoneal dialysate may also contribute to the delayed gastric emptying and interfere with food intake and utilizations (45). Additionally, there is evidence that suggests that hemodialysis may also lead to dysfunction of myenteric activity, thereby contributing to the gastric dysfunction in ESKD patients (46). Long durations of poor glycemic control has been associated with a higher incidence of gastroparesis; fortunately, prokinetic agents have been shown to ameliorate the effects and lead to improved absorption in this population (47).
MONITORING
Management of diabetic patients undergoing dialysis has numerous challenges as previously stated. The question arises as to how to monitor such a patient to mitigate the complications associated with attempting to achieve glucose homeostasis. Guidelines have proposed the use of HbA1c as a tool with a goal of <7% and a slightly less stringent goal for the elderly (48). Dialysis patients pose a challenge as their comorbidities and metabolic derangements often lead to inaccuracies in laboratory methods. These inaccuracies have led to the investigation of other laboratory modalities, namely, fructosamine and glycated albumin (2).
Fructosamine
Fructosamine is a measurement of nonenzymatically glycated serum proteins that have stable ketoamines (49). This measurement has become increasingly popular as a metric for short-term glucose monitoring in ESKD patients, with an assessment period of about 7 to 14 days (12). Fructosamine has been of interest because of its ability to more accurately predict glycemic control in states of chronic anemia regardless of the use of erythrocyte-stimulating agents (40). Despite these accuracies in altered hemoglobin levels, fructosamine has been observed to underestimate glucose control in states of dysproteinemias, such as malnutrition, protein-energy wasting, hepatic disease, steroid use, and in peritoneal dialysis (50). Although fructosamine’s accuracy offers diminishing returns in states of hypoproteinemia, studies have shown that its use may be beneficial as a predictor of increased hospitalization and infection (51). Additionally, fructosamine has been observed as a marker of cardiovascular mortality, with evidence supporting a doubling of fructosamine correlating with a twofold increase in cardiovascular disease (52).
Glycated Albumin
Similar to the formation of fructosamine, glycated albumin is a nonenzymatic reaction of glucose with albumin that is able to measure glucose control within a similar time frame of 7 to 14 days (53). Studies have proposed that glycated albumin is a better measure of glycemic control in patients with diabetes and CKD, although few studies have looked into its impact on ESKD (12). As with fructosamine, glycated albumin is not influenced by erythrocyte lifespan, anemia, or erythropoietin stimulants but is influenced by abnormal protein turnover, peritoneal dialysis, infections, malnutrition, and steroids (2). Although fructosamine and glycated albumin have many similarities, improved assays have allowed glycated albumin greater accuracy in settings of hypoalbuminemia (54). Glycated albumin has also been noted to be superior to HbA1c as large studies discovered lower values of HbA1c when compared to direct glucose measurements and glycated albumin suggesting a better representation of overall glucose control (54). Additionally, increasing concentrations of glycated hemoglobin were believed to be a strong predictor of hospitalization and overall mortality as compared to glucose levels and HbA1c (55). Despite the limitations of fructosamine and glycated albumin, further research and deeper investigation is warranted into these novel metrics as interest has shown a specific niche and predictive value in patients with ESKD.
Hemoglobin A1c
HbA1c is typically described as a nonenzymatic reaction between hemoglobin β chains and glucose and is typically illustrated as a percentage of the total hemoglobin exposed to glucose over time (39). This measurement offers a rough 120-day timeline of a patient’s average blood glucose concentration. The value is influenced by numerous factors: glucose concentration, anemia, length of glucose exposure, serum pH, and temperature; therefore, it is not unreasonable to expect alterations in HbA1c in dialysis patients with their prevalence of metabolic derangements (50). Exposures to elevated urea concentrations have been shown to lead to the formation of carbamylated hemoglobin, which is indistinguishable from HbA1c in certain assays (2). Furthermore, HbA1c appears to normalize in the dialysis population, and this can be attributed to a number of factors. With progression to ESKD, erythropoietin production diminishes leading to a state of chronic anemia. The uremic milieu also contributes to a decreased erythrocyte lifespan by leading to hemoglobinopathies and an increase in erythrocyte fragility (12). Erythropoietin has thus been frequently utilized as a stimulating agent to increase the production of erythrocytes (56). All of these factors have led to the phenomena of HbA1c normalization. Additionally, chronic anemia and a decreased erythrocyte lifespan have led to an underestimation of HbA1c, compounded by the utilization of erythrocyte-stimulating agents, which lead to the production of new, nonglycated erythrocytes and thus leading to a further decrease in HbA1c (56).
Despite the evidence of uremia and anemia affecting the value of HbA1c, guidelines from the Kidney Disease Improving Global Outcomes (KDIGO) and the Kidney Disease Outcomes Quality Initiative (KDOQI) recommend long-term and routine HbA1c measurements along with home blood glucose monitoring as a staple in the management of diabetes in patients with ESKD (57).
OPTIMUM GLUCOSE CONTROL
Appropriate glucose control in ESKD patients has long been a concern and struggle for health care professionals. Large trials have demonstrated that uncontrolled blood glucose predisposes patients to micro- and macrovascular disease and have stressed the importance of targeting HbA1c to <7% (39). More recent landmark trials such as ADVANCE trial, the VADT, and ACCORD trial pointed out that intensive glycemic control may have no benefit on cardiovascular outcomes and in patients with preexisting cardiovascular disease may even increase the risk of mortality (58). This has led to some concerns as normalization of blood glucose in patients with underlying cardiovascular disease may potentially lead to an increase in mortality (59).
As previously mentioned, ESKD patients are at risk for hypoglycemia and have been observed to normalize their HbA1c over time, with one study suggesting that about 33% of diabetic dialysis patients have an HbA1c of <6% (60). This leads to the uncertainty of an optimal glycemic target in dialysis patients (2). In an attempt to answer this question, there was a small study that showed no correlation between HbA1c and mortality. It was quickly criticized due to the lack of repeated HbA1c measurements and multiple confounders (61). A subsequent study with a longer duration of follow-up initially suggested an increase in mortality with lower HbA1c levels. This study was also criticized when adjusted for other confounders such as malnutrition and inflammation: Higher HbA1c levels correlated with mortality (60). Building on these two studies, subsequent research has pointed out that HbA1c exhibits a J-shaped curve with mortality being prevalent at <6.5% and >11% (62). More recent work has suggested that HbA1c values of <6% and >8% were associated with an increase in mortality (63). Additionally, analysis has also suggested that a lower HbA1c may be a marker for malnutrition and worsening illness (2). Currently, KDIGO and KDOQI recommend that HbA1c in dialysis patients be maintained >7% in those with comorbidities and decreased life expectancy to minimize the risk of hypoglycemia and death (57).
Although no studies have looked at a comparison of hemodialysis to peritoneal dialysis, observational data supports that both modalities might be equally efficacious (12). When diving deeper into recent literature, suggestions have arisen that hemodialysis might have a slightly better advantage in survival, but this appears to be short lived with disadvantages emerging after 2 years of use (64). Further differences appear between the two modalities in regard to diabetic patients with abnormal glucose homeostasis. Patients undergoing peritoneal dialysis, especially diabetic patients, are at risk of developing peritoneal fibrosis at a faster rate and ultimately compromising ultrafiltration (12). Additional challenges in glucose management stem from the high glucose concentration in the peritoneal dialysate. This large glucose load often contributes to the patient’s morbidity and offers additional challenges in achieving glucose control (12). Glucose control may be achieved with the substitution of dextrose with icodextrin. This polymer is advantageous in that it is minimally absorbed across the peritoneal membrane, although lymphatic absorption does occur (65). While safe, its use may lead to confusion and difficulty with glucose management by the development of spurious hyperglycemia. After being absorbed by the lymphatic system, icodextrin is metabolized to maltose metabolites, which some on glucometers will register as significant hyperglycemia and if not educated properly may lead the patient to receive a larger insulin load (65).
THERAPEUTIC MANAGEMENT OF DIABETICS IN END-STAGE KIDNEY DISEASE POPULATION
There is a continuing challenge in treating patients with progressive diabetic nephropathy and specifically ESKD. Not only is mortality linked to medication side effects, namely, hypoglycemia, but cardiovascular risk of death is heightened in this population as well (57). Insulin therapy is mainstay therapy in diabetic patients with ESKD, largely due to contraindication of most oral hypoglycemic agents in severe renal impairment. In general, oral medications are not indicated in this group because of increased drug accumulation with decreased renal clearance leads to adverse drug effects such has hypoglycemia. Dose adjustments are required in CKD and ESKD in those medications that can be used in diabetic patients in ESKD (TABLE 31.1) (66).
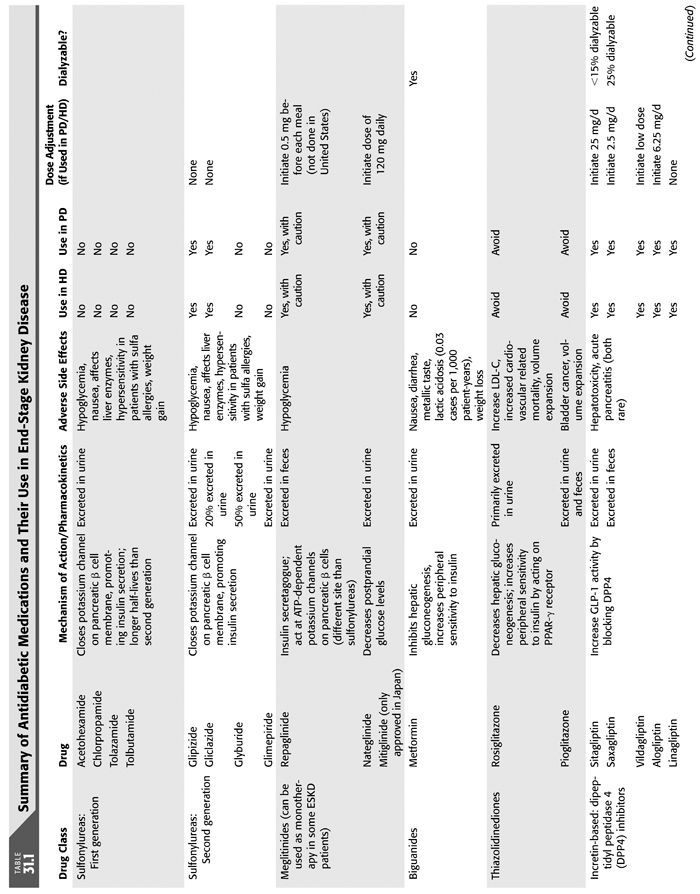
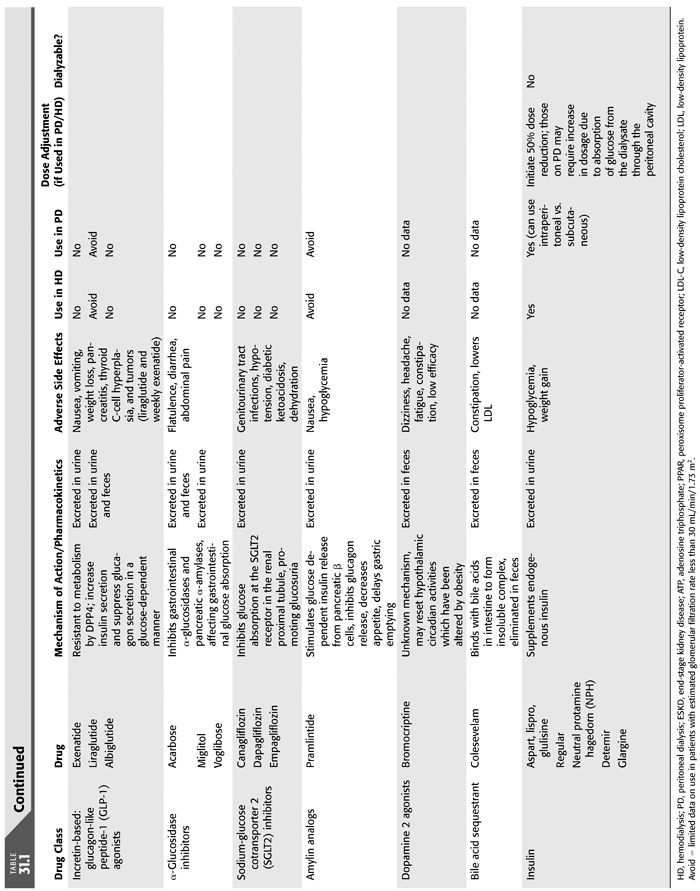
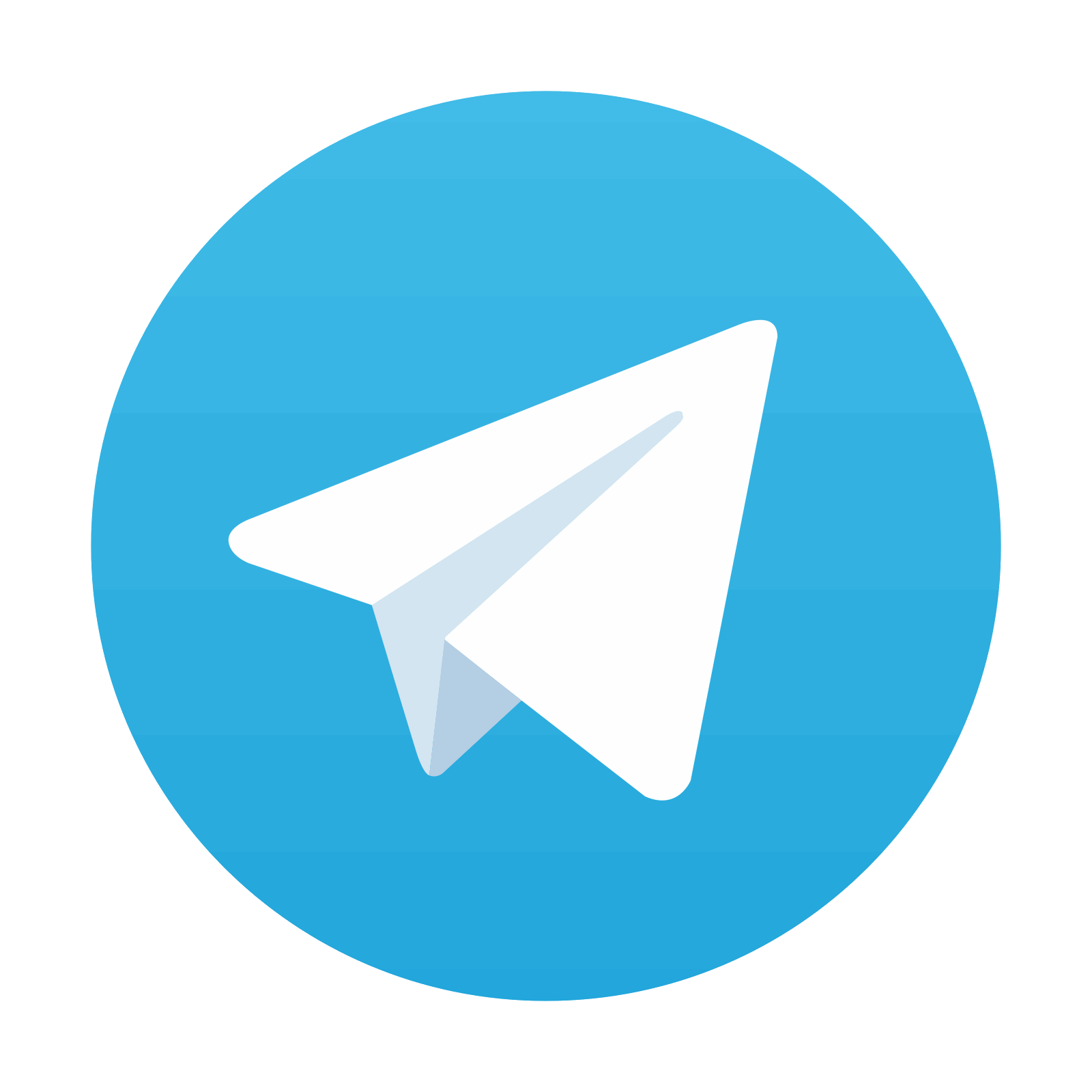
Stay updated, free articles. Join our Telegram channel

Full access? Get Clinical Tree
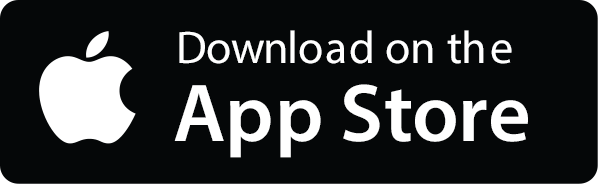
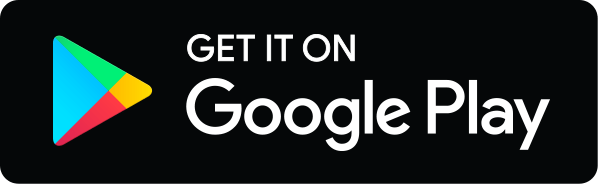