Advantages
Disadvantages
Increases display dynamic range (increased sensitivity)
Susceptible to tissue motion artifacts
No multiple angle artifact
No direction information
No aliasing
No velocity information
Limited angle dependence
Limited temporal resolution
Better functional lumen/vessel wall definition
3 Intravenous Contrast Agents
3.1 History
Echocardiographers recognized by chance in the 1960s that the tiny air bubbles incidentally introduced into the circulation after intravenous injections produce transient echo enhancement in the right side of the heart. Such bubbles do not pass through the normal pulmonary circulation, but systemic enhancement effects can be used to test for a right-to-left shunt (Blomley and Cosgrove 1997).
The first microbubble agents were described by Gramiak and shah in 1968. They were discovered during the injection of indocyanine green as M-mode echocardiography was being developed. More precisely, their first encounter with the contrast phenomena came in late April 1967 in the cardiac catheterization laboratory during study of a patient with aortic regurgitation. Contrast injections were decisive in identifying the walls of the aortic root, distinguishing it from the left ventricular outflow tract and detailing normal cusp motion. The resulting publication was the first report of the use of ultrasonic contrast agents (Gramiak and Shah 1968). Serious attempts to develop intravenous contrast agents for ultrasound began in the late 1970s and early 1980s. It was subsequently surmised that bubbles were responsible for the contrast effect. In the early 1980s, clinical echocardiography was performed with a sector scanner, a stand-alone Doppler unit capable of pulsed and continuous-wave mode operation, a spectral analyzer with high temporal resolution and an in-house “black box” which allowed EKG display along with the spectra (Gramiak and Holen 1984). Due to the fact that small gas bubbles dissolve rapidly in blood, the next aim was the production of agents small enough to traverse the pulmonary capillary bed.
Smith et al. (1984, 1989) used a galactose-based contrast agent called SHU-454 (Echovist, Schering AG, Berlin, Germany), whereas successful transpulmonary delivery of microbubbles was achieved by using an agent similar to SHU-454 with the addition of palmitic acid surfactant—called SHU-508A (Levovist, Schering AG, Berlin, Germany; Smith et al. 1984, 1989).
In the late 1980s, the systematic development of contrast media started. Nearly all of them consisted of gas bubbles, since gas has, for physical reasons, the optimum prerequisites for an ultrasound contrast agent. Due to the high efficacy and low specific density of gas, only small amounts of foreign substance have to be administered (Ophir and Parker 1989). To make the contrast-giving gas bubbles suitable for transpulmonary passage after intravenous injection, the bubbles were surrounded with a thin film to prevent dissolution of the bubble in the blood stream. The material of the protective layer around the microbubble is in most cases a highly flexible structure which does not reduce the acoustic response by limiting the oscillation of the bubble. Transpulmonary passage was now possible and, therefore, the arterial part of the vascular system could be assessed. For imaging of different organs, contrast agents which are concentrated in organs were necessary. Therefore, there was a need for agents which can survive cellular uptake without losing their acoustic properties.
Although backscatter is highly dependent on bubble size, microbubbles must have a limited size range. If the bubble is too small, it will be short-lived (i.e. it will collapse under cardiac and systemic pressures). If the bubble is too large (> than 10 μm), the pulmonary capillaries will trap it or the bubbles may transiently obstruct the capillaries and act as gas emboli. Therefore, microbubble size is limited from 1 to 5 μm in diameter. At present, all microbubble contrast agents which have been developed for ultrasound are small gas filled microbubbles, about 3 μm in size and all commercial ultrasound contrast media for human applications are encapsulated microbubbles. So, microbubbles are too large to escape the capillaries and, contrary to most X-ray and MR contrast media, which are rapidly distributed to the extravascular, extracellular space, most microbubbles are confined to the vascular bed. The increase in Doppler signal intensity they produce is linearly proportional to relative microbubble concentration.
3.2 Types
Levovist ® (Schering AG, Berlin, Germany) is an agent like those described above, made of galactose microparticles and palmitic acid. The microparticles adsorb the gas dissolved in the water, forming micrometer-sized air bubbles that remain stable for several minutes, thanks to the palmitic acid additive. After transit through the pulmonary circulation, the bubbles reach the systemic circulation where they enhance the backscattering of signals from blood vessels (Bouakaz et al. 1998).
The main mechanisms for signal enhancement are backscattering, bubble resonance and bubble rupture. These mechanisms are highly dependent on the acoustic power of the transmitted ultrasound, which is reflected by the mechanical index (MI). Backscattering means that ultrasound is reflected whenever there is a change in acoustic impedance. The larger the change, the more ultrasound is reflected. Bubble resonance means that at intermediate acoustic power (MI between 0.1 and 0.5) gas microbubbles may show strong oscillatory motion, provided the frequency of the ultrasound beam is close to the resonant frequency of the microbubbles. Small bubbles (< than 5 μm) will have resonance frequencies in the frequency range used in medical ultrasound imaging. The bubbles are more easily expanded than compressed, and this nonlinear behavior creates harmonics, which are exploited in nonlinear imaging. At a MI > 0.5, the microbubble resonance frequency will cause the bubbles to rupture.
In summary, microbubbles work by resonating in an ultrasound beam, rapidly contracting and expanding in response to the pressure changes of the sound wave. By a coincidence, they vibrate particularly strongly at the high frequencies used for diagnostic ultrasound imaging. This makes them several thousand times more reflective than normal body tissues. In this way, they enhance both grayscale images and Doppler signals (by raising the intensity of weak signals to a detectable level; Blomley et al. 2001).
SonoVue ® (Bracco, Milan, Italy) is a second generation ultrasound contrast agent. It contains sulfur hexafluoride (SF6), a gas with low solubility in blood for the gaseous phase of the microbubbles, and of a phospholipidic monolayer for the shell. Due to the high flexibility of the shell, the microbubbles are strongly echogenic in a wide range of frequencies and acoustic pressure. The mean microbubble diameter is 2.5 μm. The concentration of the microbubbles is between 100 and 500 million per milliliter. Contrast agents of the second generation, e.g., SonoVue®, are of diagnostic value even at very low transmission power (MI < 0.3) because of their physical behavior during insonation. The high molecular weight gas with low solubility in water was selected since laboratory tests showed that it confers to the bubbles a good resistance to pressure changes such as those that occur in the left ventricle, in the pulmonary capillaries, or in the coronary circulation (Schneider 1999), therefore, continuous real-time sonography can be performed without destruction of the microbubbles, which is a benefit for the dynamic analysis of contrast enhancement in the early phase. The microbubbles circulate in vessels, crossing the pulmonary and systemic capillary circulation. During low-MI imaging, the SonoVue® microbubbles have a much higher nonlinear behavior than native tissue, resulting in detectable echoes. Due to the long persistence of the microbubbles, SonoVue® is also potentially useful in the assessment of myocardial perfusion, as well as microcirculatory disorders. Real-time observation of microbubbles allows the detection of all parts of the vascular system. Even single microbubbles slowly traversing tissue, which is indicative of the presence of capillaries, can be shown. In comparison with Levovist®, SonoVue® has the advantage that its microbubbles are stable against the ultrasound beam, which allows continuous real-time observation of microbubble signals (Von herbay et al. 2004). In contrast, analysis of Levovist® enhancement has to be performed with the interval delay technique because the rupture of Levovist microbubbles does not allow real-time scanning.
3.3 Indications/Applications
Levovist® as an intravenous microbubble contrast agent is particularly useful in detection of small vessel flow, especially in those areas in which Doppler sensitivity limits performance, including Doppler examinations, in which the signal-to-noise ratio determines detectability. Intravenous administration of Levovist is an effective method of markedly enhancing cardiac, femoral arterial, and transcranial Doppler signal intensity (Campani et al. 1998; Meairs et al. 2000), in the evaluation of breast tumors (Duda et al. 1993), and in the detection of liver metastases (Blomley et al. 1999). Concerning the role of microbubble contrast agents in cardiology, they help in assessing the left ventricular function, and they can estimate the ejection fraction, assess the wall motion, and detect left ventricular thrombus. Emerging roles of microbubbles in cardiology include assessing myocardial perfusion (Mulvagh et al. 2000). Contrast agents represent a useful and safe method in the case of an insufficient native signal in transcranial Doppler investigation, where the scull greatly attenuates the ultrasound signal (Ries et al. 1993). Microbubbles can also be administered into body cavities, allowing simple functional tests to be performed. For example, vesicoureteric reflux in children can be revealed by injecting them into the bladder cavity and scanning the kidneys and ureters (Darge et al. 1999). Color-mode sonography can show the angioarchitecture of superficial lymph nodes to detect vessel abnormalities, a possible feature of malignancy. Although more intranodal flow patterns can be detected in such lymph nodes with contrast-enhanced sonography, the use of these agents does not improve the diagnostic accuracy in identifying malignant lymph nodes (Schulte-Altedorneburg et al. 2003). Contrast-enhanced sonography is used in several other clinical settings, to the point of assessing ophthalmic tumor perfusion (Schlottmann et al. 2005a).
Perhaps, the most promising clinical application of contrast-enhanced sonography is the imaging of the liver. The main practical importance is that many focal liver lesions, particularly metastases and hepatocellular carcinoma, appear as defects. Scanning in pulse-inversion mode after Levovist improves the detection of liver metastases and reveals more lesions of smaller size than conventional ultrasonography and computed tomography (Harvey et al. 2000). The method seems particularly useful in detecting small lesions (<1 cm diameter), for which all imaging methods lack sensitivity. Contrast agents may also increase specificity in liver imaging since some lesions can be characterized by their enhancement patterns. Because the bubbles are destroyed by ultrasound, e.g., liver enhancement lasts for 1 or 2 frames as the transducer is swept across the bubble-filled liver. Because space-occupying lesions lack sinusoids and reticulo-endothelial cells, they appear hypoechoic relative to the enhanced background of the liver. Even biopsies of liver lesions under real-time continuous contrast harmonic imaging at low MI are feasible (Schlottmann et al. 2004); however, one limitation of this imaging modality is the difficulty in accessing the whole of the liver.
Concerning the bowel wall, contrast enhancement of defined layers of the wall can be shown even though B-mode visualization of small vessels in the bowel wall is impossible. The more or less homogenous enhancement of particular layers will increase with the level of hyperperfusion of the intestinal wall (Schlottmann et al. 2005b).
In general, the nonlinear harmonic echoes generated by the SHU 508 bubbles are visualized at high transmit power. This high MI technique is used more widely to analyze tissue perfusion and hepatic metastases rather than perfusion of visceral vessels (Schlottmann et al. 2005b).
Using the contrast agent SonoVue®, the microbubbles act as reflectors for the ultrasound beam enhancing backscatter beam. The agents remain in the circulation and, according to the perfusion phase (arterial, capillary, portal venous), lead to enhancement of different structures. This imaging modality allows an analysis of tumor perfusion under real-time conditions.
3.4 Safety
Injecting a gas into the circulation may seem potentially hazardous, but extensive clinical experience has shown that the tiny volume of the air or gas given (<200 μl) is not dangerous, and the safety of microbubbles compares well to that of conventional agents in radiography and MRI (Nanda and Carstensen 1997). Ionescu reported a clinical case of a patient who developed a rare but dramatic sequence of reversible complications (anaphylactic shock, threatened myocardial infarction, and transient renal failure) from exposure to SonoVue® (Ionescu 2009). In 2009, Geleijnse et al. (2009) described side effects (from mild allergic reactions to nonfatal shock) in 2 % of their patients (n = 352) (Geleijnse et al. 2009). At the moment, the use of SonoVue® is not permitted in mechanically ventilated intensive care unit patients and in patients with heart failure or severe hypertonus.
4 Contrast Harmonic Imaging
The observation that insonation of microbubbles induces nonlinear harmonic echoes caused by the oscillation or disruption of the microbubbles was followed by the development of imaging techniques which visualized the nonlinear harmonic signals of bubble oscillation and disruption (contrast harmonic imaging, CHI). Contrast harmonic imaging (CHI) utilizes the nonlinear properties of contrast agents by transmitting at the fundamental frequency but receiving at the second harmonic. The advantage of this processing scheme lies in the difference in backscattering for tissue and contrast agent at two frequencies. The extent of the backscattered contrast-enhanced signal at the harmonic frequency is much greater than that of the tissue. This technology works in grayscale imaging as well as in Doppler sonography. The harmonic response of the agent is strong and, in combination with the high stability of the microparticles, blood flow in small vessels can be directly visualized. This method is not influenced by attenuation.
5 Instrumentation Technology
The other area of significant expansion in this field was brought by the instrumentation technology, where specific pulsing and post-processing techniques have been developed to take advantage of the presence of microbubbles in the circulation.
Pulse-inversion sonography (also called second harmonic) is a technique that works by sending two separate pulses, 180° out of phase, and summing the reflected echoes to form the final sonographic signal.
Increasingly sophisticated, broadband or multiple focusing transducers also play an important role. Contrast-enhanced sonography can be performed not only with 3.5 MHz transducers usually used in abdominal ultrasound, but also with 5–12 MHz transducers which offer a higher spatial resolution.
6 Intravenous Contrast-Enhanced Bowel Sonography
6.1 Inflammatory Bowel Diseases
Crohn’s disease (CD) manifests with highly variable signs and symptoms, and the assessment of the status of the disease, in the single patient, can be difficult. Being a chronic inflammatory bowel disease, it is characterized by an inflammatory process that involves the full thickness of the bowel wall, including the mesentery and the lymph nodes. Many clinical and laboratory parameters have been proposed to indicate disease activity; however, none of these indices can provide a fully reliable assessment of disease status. As mentioned above, in recent years, transabdominal bowel sonography has become increasingly important as a reliable and non-invasive tool in the diagnosis and follow-up of patients with CD disease. Ultrasonography is able to diagnose and locate transmural bowel inflammation in CD, discriminate it from ulcerative colitis (UC) and detect complications such as fistulas, strictures, and abscesses. However, small intraperitoneal abscesses may remain unidentified (Kolkman et al. 1996). Nevertheless, the early detection of intestinal complications in Crohn’s disease is probably one of the most important aspects of the management of these patients. The detection of such complications in the initial stages of the disease allows effective medical therapy to control their progression, thus avoiding surgical treatment in some cases.
Intestinal hyperemia is a sign of active disease in the inflamed intestine which can be detected by Doppler sonography. Tissue motion artifacts (peristalsis) sometimes make the assessment difficult, but visualization of vessels by using contrast harmonic imaging at a low MI is not restricted by peristalsis (Fig. 1).
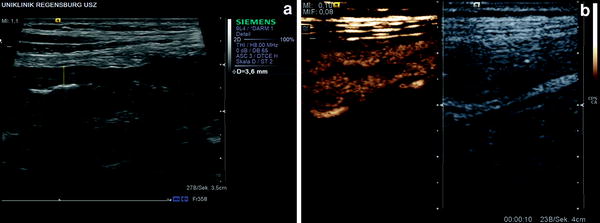
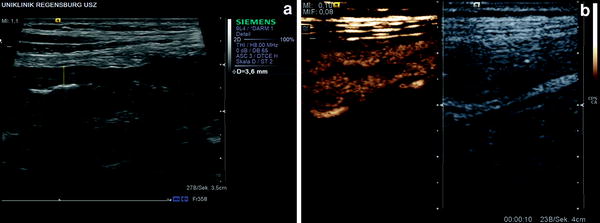
Fig. 1
25-year-old male patient with ulcerative colitis. a B-mode sonography shows a thickened bowel wall (about 4 mm). b Contrast-enhanced sonography shows a rapid and increased bowel wall perfusion—10 s after injection of 2.4 ml SonoVue®
Esteban et al. (2003) have demonstrated for the first time the usefulness of contrast agents in the detection and follow-up of inflammatory abdominal masses associated with CD. Grayscale, Doppler, and pulse-wave ultrasound was undertaken with additional contrast-enhanced power Doppler examination. These findings were correlated with each other and with contrast-enhanced CT as the gold standard. After Levovist® injection, all the lesions showed intense vascularization in the interior and/or the peripheral soft tissue. The authors could show that contrast-enhanced power Doppler sonography demonstrated more abdominal masses than CT (Esteban et al. 2003).
Several studies were performed to detect bowel-wall hyperemia in inflammatory bowel disease (Fig. 2).
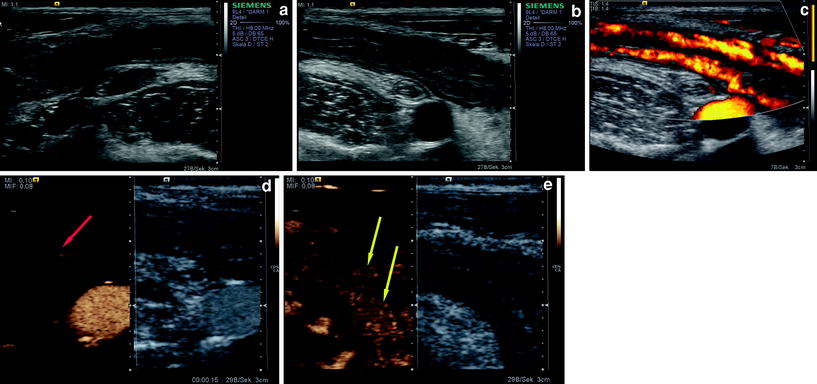
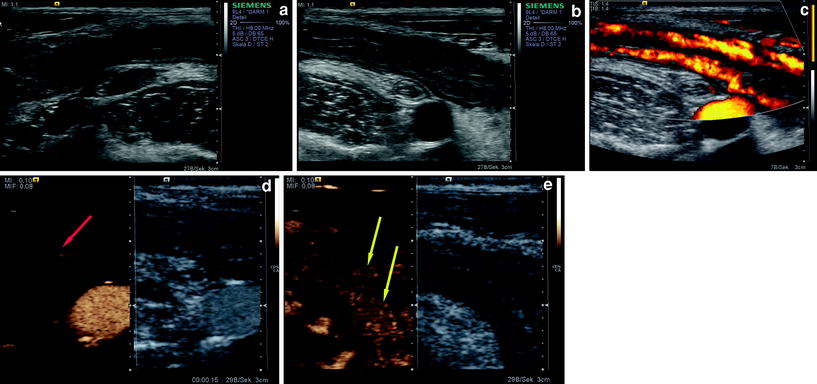
Fig. 2
24-year-old female patient with Crohn’s disease. a B-mode sonography shows an increased bowel-wall thickness with surrounding lymph nodes in the right lower quadrant of the abdomen. b B-mode sonography about the same region showing a prominent appendix. c Power Doppler sonography shows increased vascular signals. d Contrast-enhanced sonography (15 s after injection of 2.4 ml SonoVue®) shows the arterial arrival of the microbubbles and the feeding vessel (red arrow) of the bowel wall. e Slightly later, the signals in the bowel wall are seen (yellow arrows)
Contrast harmonic imaging at a low MI is technically feasible for the demonstration of increased intestinal perfusion in inflammatory bowel disease by using high frequency ultrasound transducers (Plikat et al. 2004; Migaleddu et al. 2009; Kumar et al. 2009). One of the drawn conclusions of a study of Rapaccini et al. (2004) was that intestinal loops with thickened walls should be subjected to color power Doppler sonography, and if intramural flow signals are absent the examination should be repeated with echo enhancement since the presence of color flow signals shows good concordance with clinical and laboratory indices of active disease. In patients with active disease, power Doppler signals emerged in the intestinal wall. In other words, the utilization of echo-enhancer media improves the diagnostic sensitivity of intestinal wall power Doppler scan (Table 2).
Table 2
Characteristics of patient subgroups with active and quiescent crohn’s disease (From Rapaccini et al. 2004)
Quiescent disease | Active disease | P | |
---|---|---|---|
No. of patients | 26 | 22 | – |
Intestinal wall thicknessa | 6.8 ± 1.3 mm | 7.5 ± 1.3 mm | 0.11 |
SMA RIb | 0.83 ± 0.02 | 0.81 ± 0.01
![]() Stay updated, free articles. Join our Telegram channel![]() Full access? Get Clinical Tree![]() ![]() ![]() |