Choice of Mesh
Arthur Rawlings
Brent D. Matthews
If we could artificially produce tissue of the density and toughness of fascia and tendon, the secret of the radical cure of hernia repair would be discovered.
Theodore Bilroth (1829–1894)
Introduction
Edoardo Bassini ushered in the modern era of hernia repair in 1887 with his “radical cure” for an inguinal hernia on the basis of an anatomical repair. Despite improved understanding of abdominal wall anatomy, the advent of aseptic technique, the development of antibiotic therapy for prophylaxis, and refined surgical skills over the decades, recurrence from a tissue repair of an abdominal wall hernia occurs at an alarming rate. This is not the “radical cure” that Bassini envisioned for an inguinal hernia nor for any abdominal wall hernia. For example, one study showed that a primary repair of a large ventral hernia is reported to have a 63% recurrence rate at 10 years. This is reduced to 32% if a mesh is used to augment the primary closure. If the hernia is small, less than 10 cm2, then the recurrence rate for a primary repair is 67%, whereas it drops to 17% if a mesh is used to augment the repair. Though there is much to learn about hernia anatomy and its usefulness in repair, studies have demonstrated that a mesh should be a primary tool for an abdominal wall hernia repair. A mesh should be used unless there is a compelling reason not to use one. With so many options available the question becomes, “Which one?”
What is the Ideal Mesh?
Before discussing what is available, it would be a good exercise to consider what would be an ideal mesh. What is being asked from a piece of mesh in an abdominal wall hernia repair? There are several desired characteristics, some absolute while others only highly desirable. The ideal mesh would be (in no significant order):
Noncarcinogenic
Strong enough to prevent a recurrence
Easy to handle
Easy to manufacture
Economical
Biocompatible: Having a minimally adverse or no inflammatory host response, or being completely remodeled into the host tissue
Treatable if it becomes infected
Undetected by the patient or by physical examination
Compatible with future abdominal access
Nonallergenic or causing no hypersensitivity reaction
On looking over the list, it is easy to say that the ideal mesh has yet to be produced. This does give a good benchmark for the evaluation of what is on the market and a goal for future developments.
What is Available?
Phelps used the first man-made prosthetic material for hernia repair in 1894. He placed silver wire coils in the floor of the inguinal canal and closed the layers of the abdominal wall over them. He relied on the host response to this foreign body to increase the fibrosis in the inguinal floor to reinforce the hernia repair. This was further developed by German surgeons who used hand-made silver filigrees, fine silver wire woven into a net, as the first “mesh” to be routinely used for hernia repairs. Though this has fallen out of favor, metal mesh for hernia repair was used longer than any other prosthetic material for hernia repair, including even the most popular materials used today.
Francis Usher initiated the current revolution in prosthetic materials for hernia repair when he published his use of polypropylene mesh for hernia repair in 1958. Since then many materials have come and gone; a few have stayed. Through all the experiments and trials, three nonbiologic mesh materials have stood the test of time: Polypropylene, polyester, and polytetrafluoroethylene (PTFE).
Polypropylene
Polypropylene, the mesh used by Usher, is a polymer of a carbon backbone with hydrogen and methyl groups attached (Fig. 23.1). It looks as if it would be inert in the human host, but this structure initially undergoes oxidation at the tertiary carbons, which then can progress to oxidation of the carbon backbone. The impact of this clinically is that explanted meshes have shown oxidative damage with surface cracking, a decrease in
mass, and reduced compliance. This polymer can be manufactured into weaves or knits of different patterns and densities. Absorbable strands can also be woven together with the polypropylene to give the mesh a stiffer feel and easier handling characteristics for implantation, which will then become more pliable in the patient as the body degrades the absorbable strands.
mass, and reduced compliance. This polymer can be manufactured into weaves or knits of different patterns and densities. Absorbable strands can also be woven together with the polypropylene to give the mesh a stiffer feel and easier handling characteristics for implantation, which will then become more pliable in the patient as the body degrades the absorbable strands.
Polyester
Polyester is a polymer of a carbon and oxygen backbone with hydrogen and oxygen attached (Fig. 23.2). This polymer comes in many different forms, polyethylene terephthalate (PET or Dacron) being one of the most common. Its versatility and strength to weight ratio make it a popular fabric for clothing. This material also looks as if it would be inert in the human host, but that is not the case. Polyester is hydrophilic and undergoes hydrolysis whereas polypropylene is hydrophobic and undergoes oxidation. The hydrolysis of polyester can break the backbone of the polymer in a slow process that eventually can turn the polymer into a monomer. For example, one study looked at 65 explanted polyester vascular grafts and showed by a linear regression model that the bursting strength is reduced by 31.4% at 10 years and 100% by 25 to 39 years. The clinical significance for this in abdominal hernia repair is not fully known, but it does highlight that these seemingly inert materials do undergo change in the human host. In general terms, polyester tends to have less scar contraction, less tissue adherence, and feels softer than polypropylene.
Polytetrafluoroethylene (PTFE)
Polytetrafluoroethylene (PTFE) is a polymer of fluorine atoms attached to a carbon backbone (Figs. 23.3 and 23.4). Its most commonly known commercial applications are
Teflon and Gore-Tex. The carbon–fluorine bond is one of the strongest organic bonds known. This means that PTFE is more resistant to oxidation in the biochemical milieu of the human host than polyester or polypropylene. Tissue enzymes and microorganisms appear to not degrade this mesh. These properties led pediatric surgeons, who wanted a prosthetic that could be easily removed from the patient’s body at a later date, to be the first to use PTFE as a prosthetic material. Since then, PTFE meshes have been engineered with different pore sizes on each side to take advantage of the host tissue’s different interactions with pore size. These expanded PTFE (ePTFE) meshes have been designed with very small pores on one side, which significantly reduces adhesions, and large pores on the other side, where tissue can grow into the material. This extremely small pore size means that ePTFE performs poorly in the presence of infection. Unlike polypropylene and polyester, which performs reasonably well in a contaminated environment or when exposed to the outside by allowing granulating tissue to grow between the mesh strands, ePTFE usually has to be removed if there is an infection or if it becomes exposed. It is more prone to seroma formation and encapsulation than polypropylene and polyester. But, with its small pore composition, it does not develop adhesions like bare polypropylene or polyester.
Teflon and Gore-Tex. The carbon–fluorine bond is one of the strongest organic bonds known. This means that PTFE is more resistant to oxidation in the biochemical milieu of the human host than polyester or polypropylene. Tissue enzymes and microorganisms appear to not degrade this mesh. These properties led pediatric surgeons, who wanted a prosthetic that could be easily removed from the patient’s body at a later date, to be the first to use PTFE as a prosthetic material. Since then, PTFE meshes have been engineered with different pore sizes on each side to take advantage of the host tissue’s different interactions with pore size. These expanded PTFE (ePTFE) meshes have been designed with very small pores on one side, which significantly reduces adhesions, and large pores on the other side, where tissue can grow into the material. This extremely small pore size means that ePTFE performs poorly in the presence of infection. Unlike polypropylene and polyester, which performs reasonably well in a contaminated environment or when exposed to the outside by allowing granulating tissue to grow between the mesh strands, ePTFE usually has to be removed if there is an infection or if it becomes exposed. It is more prone to seroma formation and encapsulation than polypropylene and polyester. But, with its small pore composition, it does not develop adhesions like bare polypropylene or polyester.
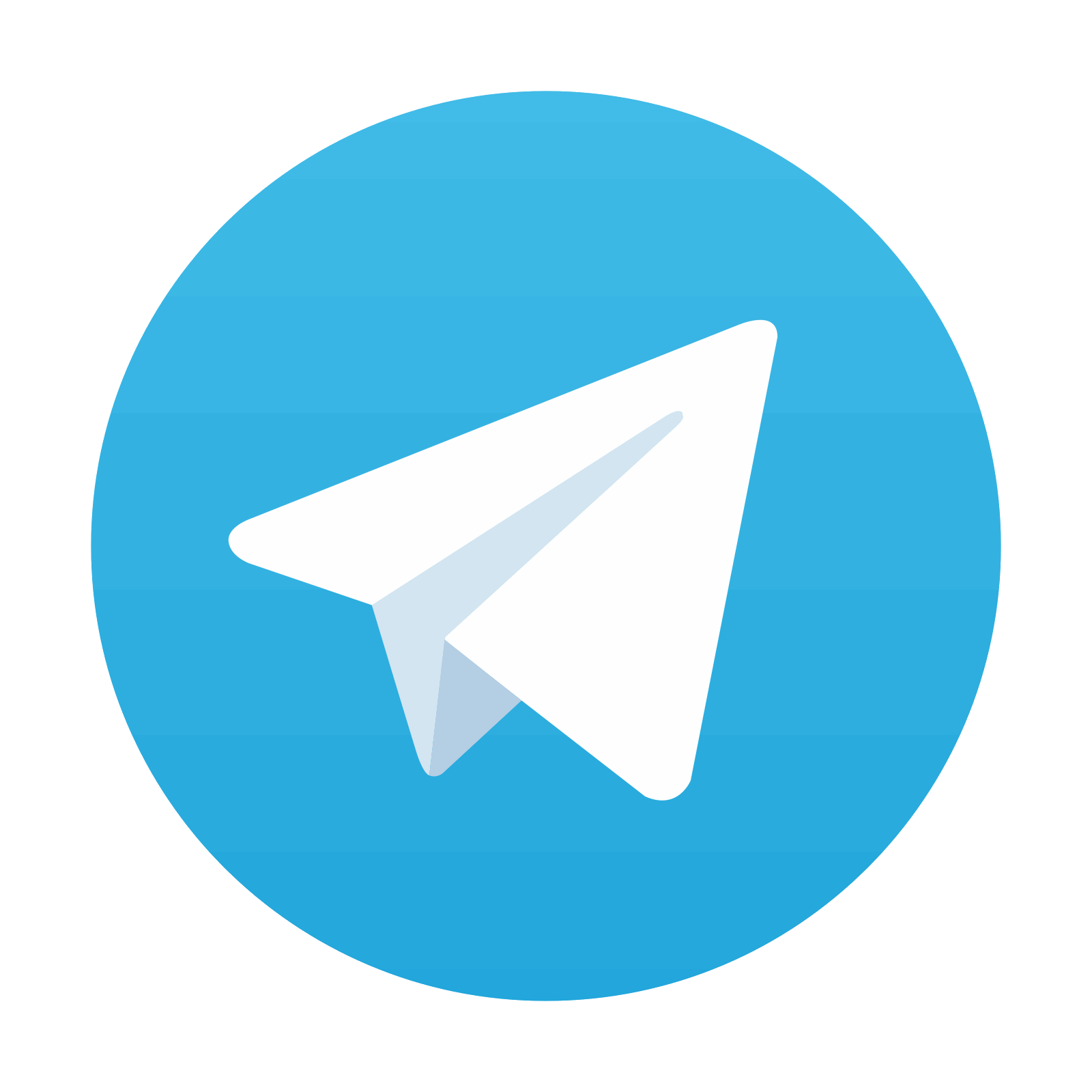
Stay updated, free articles. Join our Telegram channel

Full access? Get Clinical Tree
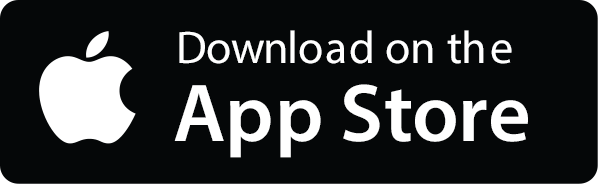
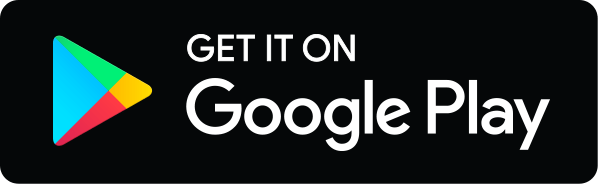