Fig. 3.1
Amniotic stem cells

Fig. 3.2
Differentiation potency of amniotic fluid stem cells into muscle, neuron, endothelium, bone, fat, and chondrocyte
The stem cells are usually extracted from the amniotic sac by amniocentesis which occurs without harming the embryos. The use of amniotic fluid stem cells is therefore generally considered to lack the ethical problems associated with the use of cells from embryos. The majority of stem cells present in the amniotic fluid share many characteristics, which suggests they may have a common origin.
This cell was confirmed that the amniotic fluid contains a heterogeneous mixture of multipotent cells after it was demonstrated that they were able to differentiate into cells from all three germ layers but they could not form teratomas following implantation into immunodeficient mice. This characteristic differentiates them from embryonic stem cells but indicates similarities with adult stem cells. However, fetal stem cells attained from the amniotic fluid are more stable and more plastic than their adult counterparts making it easier for them to be reprogrammed to a pluripotent state.
A variety of techniques has been developed for the isolation and culturing of amniotic stem cells. One of the more common isolation methods involves the removal of amniotic fluid by amniocentesis. The cells are then extracted from the fluid based on the presence of c-Kit. Several variations of this method exist. There is some debate whether c-Kit is a suitable marker to distinguish amniotic stem cells from other cell types because cells lacking c-Kit also display differentiation potential. Culture conditions may also be adjusted to promote the growth of a particular cell type.
Amniotic fluid highly contained MSCs and several techniques have been described for MSCs isolation. They usually involve the removal of amniotic fluid by amniocentesis, and their distinction from other cells may be based on their morphology or other characteristics. Human leukocyte antigen testing has been utilized to confirm that the MSCs are from the fetus and not from the mother. Comparison of amniotic fluid-derived MSCs to bone marrow-derived ones showed that the former has a higher expansion potential in culture. However, the cultured amniotic fluid-derived MSCs have a similar phenotype to both adult bone marrow-derived MSCs and MSCs originating from second-trimester fetal tissue(Fig. 3.3).


Fig. 3.3
Mesenchymal cell markers expression analysis of amniotic stem cells by FACS
As like mesenchymal stem cells, embryonic-like stem cells also are abundant in the amniotic fluid. Embryonic-like stem cells were originally identified using markers common to embryonic stem cells such as nuclear Oct4, CD34, vimentin, alkaline phosphatase, stem cell factor, and c-Kit. However, these markers were not necessarily concomitantly expressed. In addition, all of these markers can occur on their own or in some combination in other types of cells. The pluripotency of these embryonic-like stem cells remains to be fully established. Although those cells which expressed the markers were able to differentiate into muscle, adipogenic, osteogenic, nephrogenic, neural, and endothelial cells, this did not necessarily occur from a homogenous population of undifferentiated cells. Evidence in favor of their embryonic stem cell nature is the cells’ ability to produce clones (Figs. 3.4 and 3.5).



Fig. 3.4
Pluripotency marker expression of amniotic fluid stem cell to the passages

Fig. 3.5
Comparison of pluripotency marker expression among amniotic fluid, bone marrow, and umbilical cord-derived stem cell
3.2 Applications
3.2.1 Stem Cell Culture
3.2.1.1 Human Embryonic Stem Cell Culture Protocol
Isolation of Primary Mouse Embryo Fibroblasts
- 1.
Dissect out the uterus of mouse (pregnant day 13.5, ICR) with sterile forceps and scissors, and place it into a Petri dish with PBS.
- 2.
Isolate the embryos and transfer into a second Petri dish with PBS.
- 3.
Remove the heads, livers, intestines, heart, and all viscera.
- 4.
Transfer the carcasses to a fresh Petri dish, and mince with a blade.
- 5.
Add 5.0 mL of 0.25% trypsin/EDTA per ten fetuses, and triturate through a 10 mL pipette.
- 6.
Transfer the solution to a 10 mL syringe with 18 G needle, slowly push the solution through the needle (two times), and collect in a 50 mL conical tube.
- 7.
Incubate for 15 min at 37°C, triturating three or four times using a 10 mL pipette.
- 8.
Add an equal volume of complete MEF medium.
- 9.
Plate to T175 flask, and add complete MEF medium to make up a final volume of 30 mL.
- 10.
The dissociated MEFs will attach to the flask and begin to divide overnight.
- 11.
Replace the medium the next day with an equal volume of fresh complete MEF medium.
- 12.
3–4 days after, rinse the cells with Ca2+/Mg2+ free PBS.
- 13.
Add 3 mL of 0.05% trypsin/EDTA solution per flask. Inactivate the trypsin solution by adding 5 mL complete MEF medium.
- 14.
Count the number of viable cells using trypan blue exclusion and a hemocytometer. Viability should be between 90 and 95%.
- 15.
Centrifuge the cell suspension at room temperature for 4 min at 200 g.
- 16.
Aspirate medium and resuspend the cells in complete MEF medium (freezing concentration, 2.4 × 107/mL). Add an equal volume of fetal bovine serum with 20% DMSO to get 1x final stocks. Dispense 1 mL per cryovial and freeze.
Preparation of MEF-Conditioned Medium, MEF-CM
- 1.
Plate 4 × 106 mitomycin C-treated MEFs in a T75 flask coated with 0.5% gelatin, in complete MEF medium.
- 2.
The next day, replace the MEF medium with 37.5 ml 20% KSR hESC medium (containing 4 ng/ml bFGF), and incubate for 24 h at 37°C, 5% CO2.
- 3.
Collect MEF-CM from the flasks after 24 h and 0.22 μM filter sterilize. MEF-CM can
Microdissection Passaging of hESCs
- 1.
Warm MEF-CM to 37°C in water bath, and add freshly thawed 1 M ß-mercaptoethanol to a final concentration of 0.1 mM.
- 2.
Assess the undifferentiated colonies from differentiated colonies. Use a fine pointed tool (fire drawn Pasteur pipette needles), and score four to five times across a colony and perpendicular to that another four to five times. This creates a grid that cuts the colony into pieces.
- 3.
Use a P1000 pipette tip to lift the colony pieces, and put them into MEF-CM.
3.2.1.2 Human-Induced Pluripotent Stem Cell Generation Protocol
Preparation of Fibroblasts
- 1.
Disinfect skin surface three times with povidone iodine, and administer lidocaine.
- 2.
Push a disposable biopsy punch against the skin, deep enough to reach the fatty layer of subcutaneous tissue.
- 3.
Put the tissue into a 100 mm dish containing FP medium (DMEM containing 10% FBS and 50 units and 50 mg/ml penicillin and streptomycin), and cut it into 1 mm pieces.
- 4.
Transfer them to a 35 mm dish, put the coverslip onto the tissues, and then add 2 ml of FP medium.
- 5.
Incubate at 37°C, 5% CO2. Leave the dish untouched for a week, and later change the medium twice a week.
- 6.
When cells cover 30–50% of the dish, it’s time to transfer the cells to a larger dish.
Lentivirus Production
- 1.
Passaging 293FT cells: Adjust the concentration to 4 × 105 cells per milliliter with 293FT medium without antibiotics. Seed cells at 4 × 106 cells (10 ml) per 100 mm dish, and incubate overnight at 37°C, 5% CO2.
- 2.
Transfection to 293FT cells: Dilute 9 μg of Virapower packaging mix (pLP1, pLP2, and pLP/VSVG mixture) and 3 μg of pLenti6/UbC encoding the mouse Slc7a1 gene in 1.5 ml of OPTI-MEM I, and mix gently. In a separate tube, dilute 36 μl of Lipofectamine 2000 in 1.5 ml of OPTI-MEM I. Mix gently. After 5 min incubation, combine the diluted DNA with the diluted Lipofectamine 2000. During 20 min incubation, remove the medium from 293FT dishes, and add 9 ml of fresh medium to each dish. Then add 3 ml of the DNA-Lipofectamine 2000 complexes in each dish. Twenty-four hours after transfection, aspirate the medium containing the transfection cocktail, and add 10 ml of fresh FP medium.
- 3.
Collection of virus-containing supernatant: 48 h after transfection, collect the supernatant of the 293FT culture with a 10 ml disposable syringe, and then filtrate it with a 0.45 μm pore size cellulose acetate filter.
Lentiviral Infection
- 1.
Seeding fibroblasts: Adjust the concentration to 8 × 104 cells/ml by adding appropriate volume of FP medium. Seed the cells at 8 × 105 cells (10 ml of cell suspension) per 100 mm dish. Incubate overnight at 37°C, 5% CO2.
- 2.
Transduction of fibroblasts: Replace medium with 10 ml/dish of the virus-containing supernatant, supplemented with 4 μg/ml Polybrene.
- 3.
Twenty-four hours after transduction, aspirate off the virus-containing medium, wash cells, and add 10 ml of fresh FP medium.
Preparation of SNL Feeder Cells
- 1.
Thawing SNL cells: Suspend the cells with 10 ml of SNL medium, and transfer to a gelatin-coated 100 mm dish. Incubate the cells in a 37°C, 5% CO2 incubator, until the cells become 80–90% confluent.
- 2.
Mitomycin C-inactivation of SNL cells: Add 0.3 ml of 0.4 mg/ml mitomycin C solution directly to the culture medium of SNL dish, and incubate 2.25 h at 37°C, 5% CO2. Then wash the cells twice with 10 ml of PBS. Add 0.5 ml of 0.25% trypsin/1 mM EDTA, and let sit for 1 min at room temperature. Neutralize the trypsin by adding 5 ml of SNL medium, and seed the cells at 1.5 × 106 cells per 100 mm dish.
Preparation of Plat-E Cells
- 1.
Thawing Plat-E cells: Suspend the cells with 10 ml of FP medium, and transfer to a 100 mm dish. Incubate the cells in a 37°C, 5% CO2 incubator. The next day, replace the medium with 1 μg/ml of puromycin and 10 μg/ml of blasticidin S and incubate until 80–90% confluent.
- 2.
Passage of Plat-E cells: Seed cells on new 100 mm dishes at 1:4–1:6 dilution. Cells should become confluent within 2–3 days.
Generation of iPS Cells
- 1.
Day 1 Plat-E preparation: Count cell number and adjust the concentration to 3.6 × 105 cells/ml with FP medium.
- 2.
Day 2 Retrovirus production (transfection into Plat-E cells): Transfer 0.3 ml of OPTI-MEM I into a 1.5 ml tube. Deliver 27 μl of transfection reagent into the prepared tube, and incubate for 5 min. Add 9 μg of pMXs plasmid DNA (respectively encoding Oct3/4, Sox2, Klf4, and c-Myc) drop by drop, and incubate for 15 min. Add the DNA and incubate overnight.
- 3.
Day 3 Retrovirus production: Aspirate the transfection reagent-containing medium and add 10 ml of fresh FP medium.
- 4.
Preparation of fibroblasts: When human fibroblasts expressing mouse Slc7a1 gene reach to 80–90% confluence, aspirate medium and wash once with 10 ml of PBS. Add 1 ml per dish of 0.05% trypsin/0.53 mM EDTA, add 9 ml of the FP medium per plate, and suspend the cells to a single cell. Count cell numbers to 8 × 104 cells/ml with FP medium.
- 5.
Day 4 Retroviral infection: 48 h post-transfection, collect the medium from each Plat-E dish, filter it through a 0.45 μm pore size cellulose acetate filter, and transfer into a 15 ml conical tube. Add 5 μl of 8 mg/ml Polybrene solution into the filtrated virus-containing medium. Make a mixture of equal parts of the medium containing OCT3/4, SOX2, KLF4, and c-MYC retroviruses. Aspirate the medium from fibroblast dishes, and add 10 ml per dish of the Polybrene/virus-containing medium. Incubate the cells for 4 h.
- 6.
Day 5–10: After 4 h–overnight culture, aspirate the medium from the transduced fibroblasts, and add 10 ml per dish of fresh FP medium.
- 7.
Day 11 Replating transduced fibroblasts onto mitomycin C-treated SNL feeder: Aspirate the culture medium and wash with 10 ml per dish of PBS. Add 1 ml per dish of 0.05% trypsin/0.53 mM EDTA, add 9 ml per dish of FP medium, and suspend the cells. Count cell numbers, and adjust the concentration to 5 × 103 or 5 × 104 cells/ml.
- 8.
Day 12~: Colonies should become visible 2–3 weeks after the retroviral infection. They can be picked up at around day 30.
- 9.
Picking up the iPS colonies: Pick colonies using a P2 or P10 Pipetman L, transfer it into the 96-well plate with 20 μl ES medium, and add 180 μl. Pipet up and down to break up the colony to small clumps, transfer cell suspension into 24-well plates with mitomycin C-treated SNL feeder cells, add 300 μl per well of human ES medium, and incubate until the cells reach 80–90% confluence.
- 10.
Passaging of iPS cells: After washing with PBS, add 0.1 ml per well of CTK solution and incubate at 37°C for 2–5 min. Washing with PBS, add 0.5 ml of human ES medium and suspend the cells to small clumps of 20–30 cells by pipetting. Transfer to a 6-well plates with mitomycin C-treated SNL feeder cells, add 1.5 ml of human ES medium, and incubate until cells become 80–90% confluent in 6-well plates.
- 11.
Expansion of iPS cells: After washing with PBS, add 0.5 ml per well of CTK solution and incubate at 37°C for 2–5 min. Washing with PBS, add 2 ml of human ES medium. Detach iPS cells by using cell scraper and break up the colonies to small clumps by pipetting. Transfer the cell suspension to a 60 mm dish with SNL feeder. Add 2 ml human ES medium, and incubate until cells reach 80–90% confluence.
3.2.1.3 Mesenchymal Stem Cell Culture Protocol
Mesenchymal Stem Cell Isolation from Human Amniotic Membrane
- 1.
Prepare phosphate-buffered saline solution (PBS), Dulbecco’s Modified Eagle’s Medium, and high glucose (HG-DMEM), and add 10% fetal bovine serum (FBS), 1% antibiotic-antimycotic solution, and a collagenase II solution at 100 U/ml in HG-DMEM without FBS or antibiotic-antimycotic solution.
- 2.
Sterilize the surgical instruments by autoclaving (standard scissors, simple dissecting forceps, fine point and toothed forceps, and a scalpel handle).
- 3.
Hold the umbilical cord, detach the amniotic membrane (AM) to obtain a fragment of approximately 5 cm2, and remove residual blood with three washes of 5 ml of PBS. Make a small cut in the AM with the scalpel to generate fragments of approximately 0.5 cm2.
- 4.
Incubate the AM with 5 ml of 0.125% trypsin/0.5 mM EDTA solution at 37°C for 30 min in a 50 ml conical tube at 37°C in a 5% CO2. Centrifuge at 250 g and 25°C for 5 min and then remove the trypsin solution.
- 5.
Add 15 ml of collagenase type II solution and incubate for 2 h at 37°C. Immediately after digestion, add 15 ml of PBS and centrifuge at 250 g and 25°C for 10 min. Discard the supernatant and wash the cellular pellet with 15 ml of PBS solution and centrifuge at 250 g and 25°C for 15 min. Discard the supernatant. Resuspend the cellular pellet in 10 ml of HG-DMEM by shaking gently.
- 6.
Seed 1 × 104 cells/cm2 in culture flask, add HG-DMEM, and incubate the cells at 37°C in a 5% CO2. Eliminate the nonadherent cell by changing the culture medium after 5–7 days. Change the culture medium every 3 days for an additional 7–10 days to obtain a cellular confluence of 90%.
- 7.
Recover the cells and incubate them for 5 min at 37°C in a 5% CO2 with 0.125% trypsin/EDTA solution. Then, collect cells and transfer to a 15 ml conical tube and centrifuge at 250 g for 5 min. Discard the supernatant and resuspend the cellular pellet in 10 ml of HG-DMEM. Culture cell suspension in two 75 cm2 culture flasks (5 ml in each flask), and maintain the culture until 90% confluence. Repeat steps until the 9th passage or subculture.
Mesenchymal Stem Cell Isolation from Adipose Tissue
- 1.
Wash ~250 mL of fat 3–5 times with PBS for 5 min in each wash, add collagenase, and incubate for 1–4 h at 37°C on a shaker.
- 2.
Add 10% FBS to neutralize collagenase, and centrifuge at 800 g for 10 min.
- 3.
Aspirate floating adipocytes, lipids, and liquid, leaving stromal vascular fraction (SVF) pellet, resuspend in 160 mM NH4Cl, and incubate for 10 min at room temperature. Centrifuge at 400 g for 10 min at room temperatures.
- 4.
Layer cells on Percoll gradient, and centrifuge at 1000 g for 30 min at room temperature. Wash cells twice with PBS and centrifuge at 400 g for 10 min each wash. Resuspend cell pellet in PBS and filter cells through 100 μM nylon mesh. Pass cells through 400 μM mesh. Centrifuge at 400 g for 10 min.
- 5.
Resuspend cell pellet in 40% FBS/DMEM and plate, and incubate at 37°C, 5% CO2 incubator overnight.
Mesenchymal Stem Cell Isolation from Cord Blood
- 1.
Dilute cord blood with RPMI Medium 1640 with a 3:1 ratio (three-part cord blood to one part RPMI).
- 2.
Using Ficoll-Paque, isolate mononuclear cells (MNCs) by density gradient centrifugation at 400 g for 30 min at room temperature.
- 3.
Transfer MNCs to new centrifuge tube and add PBS with a 1:3 ratio (1 part MNCs to 3 parts PBS). Centrifuge at 400 g for 10 min at room temperature.
- 4.
Remove supernatant and resuspend cells by adding culture medium and plate. Incubate at 37°C, 5% CO2 incubator overnight.
Mesenchymal Stem Cell Isolation from Amniotic Fluid
- 1.
Collect amniotic fluid from 8 s-trimester pregnancies.
- 2.
Four the AF samples were centrifuge 4 mL of amniotic fluid for 10 min at 300 g.
- 3.
Pellets were resuspended and cultured in DMEM with low glucose supplemented with 15% fetal bovine serum and antibiotics.
- 4.
Culture flasks were incubated at 37°C, 5% CO2.
- 5.
Medium was changed every 3–4 days until day 14.
- 6.
Treatment with trypsin/EDTA was applied to detach the cells from the bottom of the flasks (passage 0). Cell number and vitality were determined and analyzed by flow cytometry using specific CD73+/CD44+/CD45 cell surface markers.
Mesenchymal Stem Cell Isolation from Mouse Bone Marrow
- 1.
Prepare six 2 weeks-old BALB/c mice; MSCs were collected from femoral and tibial bone marrow of three mice by inserting a 26-gauge syringe at bone cavity, washing it with 10 ml of Knockout DMEM.
- 2.
After centrifuging at 1200 rpm for 10 min, bone marrow cells were resuspended in 1 ml at the corresponding culture media for cell counting and cell feasibility verification
- 3.
Cultures were maintained at 37°C with 5% CO2.
- 4.
After 72 h of culture, the medium was refreshed in an interval of 3–4 days.
Mesenchymal Stem Cells Isolated from Peripheral Blood
- 1.
The peripheral blood-derived MSCs (PB-MSCs) were obtained from mononuclear cells by density gradient centrifugation, plated at a density of 4 × 105/cm2 in 25 cm2 flasks in growth medium (GM) containing 10% fetal bovine serum (FBS) and 100 units/ml penicillin/streptomycin (pen/strep) in high-glucose Dulbecco’s Modified Eagle’s Medium and cultured in a humidified atmosphere at 37°C with 5% CO2.
- 2.
The medium was replaced twice a week and nonadherent cells were discarded.
- 3.
After 2 weeks one colony of adherent fibroblast-like cells was noticed in one of the seeded specimens. When the colony reached the approximate size of 5 cm2, cells were detached and seeded in a new flask in GM.
- 4.
Cells reached confluence after 10 days. Following first confluence, cells were passaged regularly.
3.2.2 Stem Cell Characteristic Analysis Methods
The characterization of stem cell has two purposes: monitoring the genomic integrity of the cells and tracking the expression of proteins associated with pluripotency. Genomic analysis is necessary to ensure stem cells maintained in culture have not undergone chromosomal changes through chromosomal loss or duplication or changes in their epigenetic profiles. Proteomic analysis ensures that the cells are expressing the factors necessary to maintain pluripotency.
Confirmation of the differentiated state by analyzing key genetic and protein markers ensures identification and propagation of the correct cell type. The general analysis methods for stem cells identification are karyotyping, single-nucleotide polymorphism (SNP) analysis, epigenetic profiling, flow cytometry, immunocytochemistry, RT-qPCR, western blotting, biomarker analysis, and teratoma formation in vivo.
3.2.2.1 Culture-Related Factors
Culture-Related Factors are media composition, cell density, feeder cell type/density, growth factors/additives, feeder-free culture, passage method, number of passages, freezing and thawing protocols, and microbial contamination
Possible Changes
The types of possible changes are chromosomal, phenotype/morphology, differentiation, pluripotency loss, epigenetic changes, tumorigenesis, and loss of self-renewal ability.
A number of techniques are used for genetic characterization of stem cells: karyotyping, fluorescence in situ hybridization (FISH), comparative genomic hybridization, single-nucleotide polymorphism (SNP) analysis, and epigenetic profiling. Some types of stem cells, particularly ESCs and iPSCs, are more prone to being genetically unstable and should be observed frequently for chromosomal changes.
Stem cells express both unique and specific combinations of transcription factors, cell surface proteins, and cytoplasmic proteins. Techniques used for stem cell analysis and characterization include flow cytometry, array-based analysis of the transcriptome, immunocytochemistry, western blots, and biomarker analysis.
Different classes and types of stem cells are characterized by different combinations of markers. In addition to confirming that stem cell lines are stable, markers can be used for screening during the reprogramming of somatic cells into iPSCs or to follow the progression of stem cell differentiation.
Colony-Forming Unit-Fibroblastic (CFU-F)
CFU-F assays were performed by plating MSCs (P3 to P6) in 6-well plates at 10, 50, and 100 cells/well in GM, in two replicas. After 14 days under standard culture conditions, the cells were fixed with ice-cold methanol and stained with 0.3% crystal violet. The number of visible colonies (more than 50 cells) was counted.
Karyotyping
Karyotyping is the examination of cellular chromosome number and morphology. Differences in appearance include size, position of centromeres, and changes in banding patterns. Stem cells in culture can accumulate changes including chromosomal abnormalities. Alterations in the genome can lead to changes in gene expression and cellular functions of stem cells [49] and increase the risk of the stem cells being tumorigenic. Thus, it is crucial to monitor a culture for the any chromosomal abnormalities, particularly in stem cells intended for therapeutic use.
Traditional karyotyping uses dye to stain the chromosomes of a metaphase cell in distinct banding patterns. The most common method is Giemsa staining, known as G-band karyotyping or G-banding; other methods include R-banding (reverse Giemsa staining), C-banding (constitutive heterochromatin staining), Q-banding (quinacrine staining), and T-banding (telomeric staining). Changes in banding patterns are used to identify abnormalities.
Fluorescence In Situ Hybridization (FISH)
In FISH, fluorescently labeled overlapping DNA fragments are hybridized to interphase or metaphase chromosomes. The chromosomes are formalin fixed onto a surface and chemically denatured. Fluorescence microscopy is used to detect and analyze the chromosomes. In fast FISH, chromosomes are denatured with high temperatures instead of chemically.
Spectral karyotyping (SKY) and multiplex fluorescence in situ hybridization (M-FISH) are commonly used forms of FISH for detection of changes. Both are hybridization-based combinatorial techniques using fluorescently labeled DNA probes. Each chromosome is labeled with a different combination of fluorophores specific for that chromosome, giving a unique spectral signature for each chromosome. Chromosomes are identified by their unique signatures, and then the output is pseudo-colored to aid in visualization. The difference between SKY and M-FISH is how the fluorescent signals are collected and processed. These methods can both detect translocations within and between chromosomes more accurately than traditional karyotyping; [50] however, they cannot detect inversions or duplications and deletions of less than approximately 5 Mb.
The main disadvantage of FISH for general screening of stem cells is that probes are required for the length of all the chromosomes. FISH is most commonly used for confirmation of the findings of other techniques using probes for a specific region of a chromosome. Deletions and insertions greater than ~20 kb are detected. Fast Fish is usually used for detecting specific aneuploidies that are commonly found in iPSCs and ESCs.
Comparative Genomic Hybridization
Comparative genomic hybridization can both determine chromosomal ploidy and detect copy number variations (CNVs), which are variable numbers of copies of segments of DNA. In this technique, DNA samples from the test stem cell population and from a reference population are each labeled with different probes. When this technique was first developed, reference and test DNA were denatured then hybridized to metaphase chromosomes. Using microscopy and fluorescence measurements, the chromosomes were scanned for any differences in fluorescent signals between the test and reference samples [51]. This technique detects and locates CNVs without requiring any knowledge of the particular sequence.
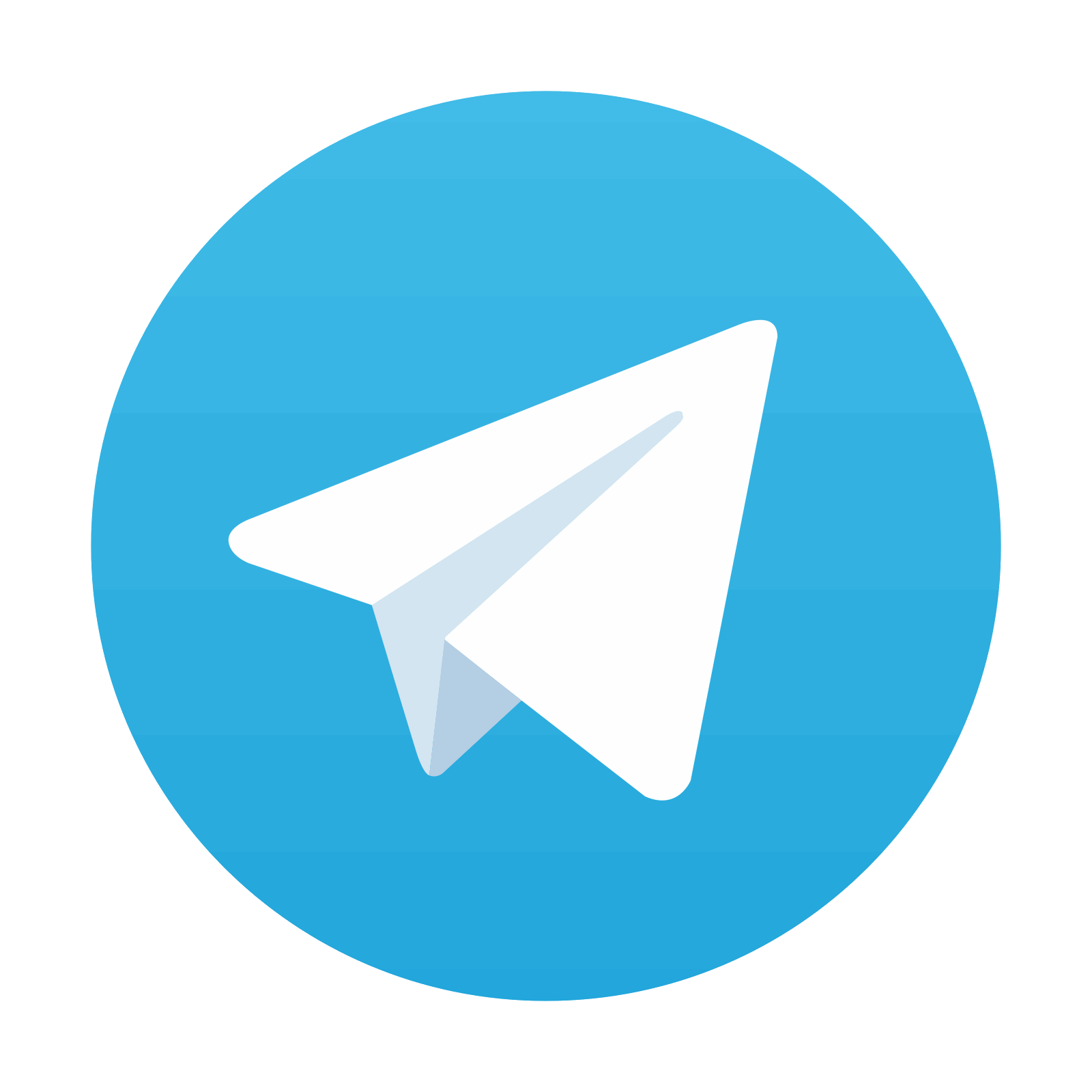
Stay updated, free articles. Join our Telegram channel

Full access? Get Clinical Tree
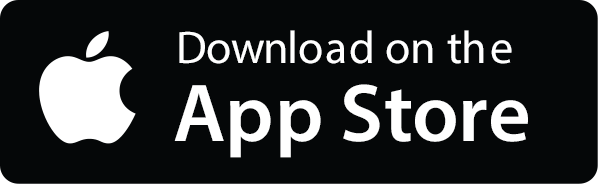
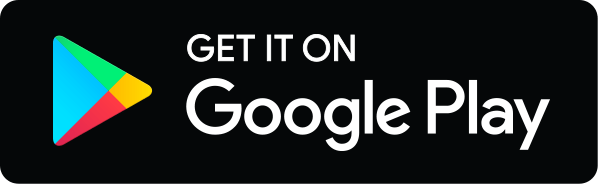
