Basic Science and Renal Transplant
Basic physiology of bladder and urethra
Bladder
The bladder has an endothelial lining (urothelium) on a connective tissue base (lamina propria), surrounded by smooth muscle (detrusor), with outer connective tissue (adventitia). Urothelium consists of multilayered transitional epithelium, with numerous tight junctions that render it impermeable to water and solutes. Detrusor muscle is a homogeneous mass of smooth muscle bundles. C-kit antigen-positive ‘interstitial cells’ exist around detrusor bundles and in the suburothelium and play a role in modulating contractile behaviour of adjacent smooth muscle. The bladder base is known as the trigone—a triangular area with the two ureteric orifices and the internal urinary meatus forming the corners. Intravesical pressure during filling is low. The main excitatory motor input to the bladder is from the autonomic nervous system and is predominantly parasympathetic innervation (S2-4). Preganglionic nerve fibres are conveyed to the bladder in the pelvic nerves and then synapse with cholinergic postganglionic nerve cells in the pelvic plexus and on the bladder, which, when activated, cause muscle contraction. Sympathetic innervation (T10-L2) plays a role in urine storage (see
p. 592).

Urethra
The bladder neck (and posterior urethra) is normally closed during filling. It is composed of circular smooth muscle (with sympathetic innervation) and is also referred to as the internal sphincter. High pressure is generated at the midpoint of the urethra in women and at the level of the membranous urethra in men where the urethral wall is composed of a longitudinal and circular smooth muscle coat, surrounded by striated muscle (external urethral sphincter).
The striated part of the sphincter receives motor innervation from the somatic pudendal nerve derived from (S2-4) in a region in the sacral spinal cord called ‘Onuf’s nucleus’. It has voluntary control and acetylcholine (ACh) mediates contraction. The smooth muscle component of the sphincter has myogenic tone and receives excitatory and inhibitory innervation from the autonomic nervous system. Contraction is enhanced by sympathetic input (noradrenaline) and Ach. Inhibitory innervation is nitrergic (nitric oxide; see
pp. 593-4).

Micturition
As the bladder fills, sensory afferent nerves respond to stretch in the bladder wall and send information about bladder filling to the central nervous system (CNS). During urine storage, the pontine storage centre mediates enhanced external urethral sphincter activity (so causing constriction of the sphincter). There is also somatic outflow via the pudendal nerve to the external striated sphincter muscle to cause contraction and sympathetic outflow to constrict the internal smooth muscle sphincter (bladder neck) and also inhibit ganglia in the bladder wall. At a socially acceptable time, the voiding reflex is activated (Fig. 18.1). Neurones in
the periaqueductal grey (PAG) matter in the pons trigger a switch to the pontine micturition centre (PMC) in the brainstem to activate the voiding reflex. Stimulation of detrusor smooth muscle by parasympathetic cholinergic nerves causes the bladder to contract. Simultaneous activation of nitrergic nerves reduces the intraurethral pressure. Inhibition of somatic input relaxes the external striated sphincter muscle and sympathetic inhibition causes coordinated bladder neck smooth muscle (internal sphincter) relaxation, resulting in bladder emptying (also see
pp. 596-7).
the periaqueductal grey (PAG) matter in the pons trigger a switch to the pontine micturition centre (PMC) in the brainstem to activate the voiding reflex. Stimulation of detrusor smooth muscle by parasympathetic cholinergic nerves causes the bladder to contract. Simultaneous activation of nitrergic nerves reduces the intraurethral pressure. Inhibition of somatic input relaxes the external striated sphincter muscle and sympathetic inhibition causes coordinated bladder neck smooth muscle (internal sphincter) relaxation, resulting in bladder emptying (also see

Basic renal anatomy
The kidneys and ureters lie within the retroperitoneum (behind the peritoneal cavity). The hila of the kidneys lie on the transpyloric plane (vertebral level L1). Each kidney is composed of a cortex surrounding the medulla which forms projections (papillae) that drain into cup-shaped epithelial-lined pouches called calyces. The calyx draining each papilla is known as a minor calyx and several minor calyces coalesce to form a major calyx, several of which drain into the central renal pelvis (Fig. 18.2). The renal artery, which arises from the aorta at vertebral level L1/2, branches to form interlobar arteries which in turn form arcuate arteries, and then cortical radial arteries from which the afferent arterioles are derived. Venous drainage occurs into the renal vein. There are two capillary networks in each kidney—a glomerular capillary network (lying within Bowman’s capsule) which drains into a peritubular capillary network surrounding the tubules (proximal tubule, Loop of Henle, distal tubule, and collecting ducts).
Anatomical relations of the kidney
Anterior relations of the right kidney are, from top to bottom, the adrenal (suprarenal) gland, liver, and hepatic flexure of the colon. Medially and anterior to the right renal pelvis is the second part of the duodenum. The anterior relations of the left kidney are, from top to bottom, the adrenal gland, stomach, spleen, and splenic flexure of the colon. Medially lies the tail of the pancreas.
Posterior relations of both kidneys are, superiorly, the diaphragm and lower ribs, and inferiorly (from lateral to medial), transversus abdominis, quadratus lumborum, and psoas major muscles.
The nephron
Each kidney has 1 million functional units or nephrons (Fig. 18.3). These consist of a glomerular capillary network, surrounded by podocytes (epithelial cells) that project into Bowman’s capsule which then drains into a tubular system. This includes a proximal convoluted tubule (PCT), Loop of Henle (LoH), distal convoluted tubule (DCT), collecting tubule (CT), and collecting duct (CD). Blood is delivered to the glomerular capillaries by an afferent arteriole and drained by an efferent arteriole. An ultrafiltrate of plasma is formed within the lumen of Bowman’s capsule, driven by Starling forces across the glomerular capillaries. Reabsorption of salt and water occurs in the PCT, LoH, DCT, and CD, although the majority of glomerular filtrate is absorbed in the PCT (Table 18.1). LoH generates hypertonicity; its descending limb is only permeable to water whereas its ascending limb is only permeable to solutes. The role of the DCT is fine adjustment of the composition of urine by selective reabsorption or secretion of solutes.
Table 18.1 Solute and water reabsorption by different parts of the nephron | |||||||||||||||||||||||||||||||||||||||||||||||||||||||
---|---|---|---|---|---|---|---|---|---|---|---|---|---|---|---|---|---|---|---|---|---|---|---|---|---|---|---|---|---|---|---|---|---|---|---|---|---|---|---|---|---|---|---|---|---|---|---|---|---|---|---|---|---|---|---|
|
Renal physiology: glomerular filtration and regulation of renal blood flow
Renal plasma clearance
Clearance is the volume of plasma that is completely cleared of solute by the kidney per minute. The clearance ratio for a substance indicates the amount of active reabsorption or excretion (i.e. ratio <1 = actively reabsorbed; >1 = actively excreted). Clearance of a substance from the plasma can be expressed mathematically as:
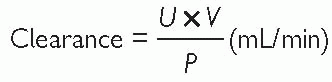
Clearance ratio = clearance/GFR
where U is the concentration of a given substance in urine, P is its concentration in plasma, and V is the urine flow rate.
Glomerular filtration rate (GFR) (see also
p. 38) Glomerular filtration is driven by Starling forces: a hydrostatic pressure gradient between capillary and Bowman’s capsule, which favours filtration, and colloid oncotic pressure which opposes filtration. GFR is the clearance for any substance which is freely filtered and is neither reabsorbed, secreted, nor metabolized by the kidney. For such a substance, clearance is equivalent to GFR. Where a substance is both filtered at the glomerulus and secreted by the renal tubules, its clearance will be greater than GFR. Where a substance is filtered at the glomerulus, but reabsorbed by the renal tubules, its clearance will be less than GFR.

Clinically, GFR is estimated using creatinine and is ˜125mL/min. Of note, serum creatinine is an insensitive marker of early renal impairment, as GFR needs to fall below 60-80mL/min before a rise in creatinine is seen.
GFR is directly related to renal plasma flow (RPF). Experimentally, GFR can be accurately calculated by measuring the clearance of inulin (a substance, which is freely filtered by the glomerulus and is neither secreted nor reabsorbed by the kidneys), using the equation:
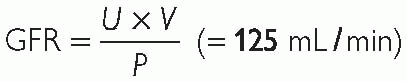
Thus, the volume of plasma from which in 1min the kidneys remove all inulin is equivalent to GFR. Factors affecting the GFR are:
Rate of blood flow through the glomerulus.
Permeability of glomerular capillary wall (K).
Surface area of glomerular capillary bed (S).
Differences in hydrostatic pressure between glomerular capillary lumen (Pgc) and Bowman’s space (Pt).
Differences in oncotic pressure between glomerular capillary (πgc) and Bowman’s space (πt) (although autoregulation of blood flow tends
to keep GFR constant despite a varying range of incoming perfusion pressures).
This can be represented by the equation:

Renal blood flow (RBF)
The kidneys represent <0.5% of body weight, but they receive 25% of cardiac output (˜1300mL/min through both kidneys; 650mL/min per kidney). Combined blood flow in the two renal veins is about 1299mL/min and the difference in flow rates represents the urine production rate (i.e. ˜1mL/min).
Autoregulation of RBF
RBF is defined as the pressure difference between the renal artery and renal vein divided by the renal vascular resistance. The glomerular arterioles are the major determinants of vascular resistance. RBF remains essentially constant over a range of perfusion pressures (˜80-180mmHg, i.e. RBF is autoregulated). Autoregulation requires no innervation and probably occurs via:
A myogenic mechanism: increased pressure in the afferent arterioles causes them to contract, thereby preventing a change in RBF.
Other factors that influence RBF
Neural mechanisms
Sympathetic nerves innervate the glomerular arterioles. A reduction in circulating volume (such as blood loss) can stimulate sympathetic nerves, causing the release of noradrenaline (NA) (which acts on α1-adrenoceptors on the afferent arteriole) to cause vasoconstriction. This results in reduced RBF and GFR.
Endocrine and paracrine mechanisms
Angiotensin II constricts efferent and afferent arterioles and reduces RBF.
Stay updated, free articles. Join our Telegram channel
Full access? Get Clinical Tree
