Dye (chromoendoscopy)
Optical
Electronic
Lugol’s solution
Magnification endoscopy
Spectrometry
Methylene blue
Narrow band imaging
Optical coherence tomography
Crystal violet
Autofluorescence imaging
Fujinon intelligent color enhancement
Indigo carmine
Endocytoscopy
iScan
Congo red
Confocal endomicroscopy
Enhanced endoscopic visualization adds valuable information during all stages of ESD. It is important during screening of patients, as it improves detection of lesions [1, 2]. During the treatment phase, it allows better delineation of the margins of the lesion, allowing accurate demarcation of the area to be resected. Some methods can also reveal changes in the mucosal surface and vascular pattern that could help establish the degree of invasion [3]. Therefore, these technologies have become particularly important for helping the endoscopist select the optimal treatment [4]. Finally, during surveillance, these methods can help distinguish between scar tissue and recurrence of or residual lesion [5, 6].
An endoscopist performing ESD needs to become familiarized with these tools. As these technologies continue to improve our visualization of the mucosa and ongoing research standardizes and validates lesion pattern recognition, it is only a matter of time before they will become standard practice.
Chromoendoscopy
Chromoendoscopy is based on the application of various dye solutions to the mucosa of the gastrointestinal tract, thus enhancing subtle mucosal changes not perceivable by purely optical methods. Several different dye stains are used in the upper GI tract, most of which are absorptive in function (e.g. Lugol’s solution, methylene blue, crystal violet, toluidine blue) and require additional time (2–20 min) for staining before interpretation can be carried out. Other agents used work as contrast (e.g. indigo carmine) or reactive agents (e.g. Congo red) [7].
The most widely used classification system was developed by Kudo, and it classifies colonic lesions into seven subtypes [8]. Lesions with advanced, superficially invasive neoplasia are the best candidates for ESD and are termed type Vi (irregular), with highly irregular yet intact pit patterns (Fig. 5.1) [8]. Deeply invasive lesions, which cannot be fully removed by ESD, typically have non-structural, or complete loss of pits. Unfortunately, these patterns typically require magnification and crystal violet staining, which is not widely used in the West.
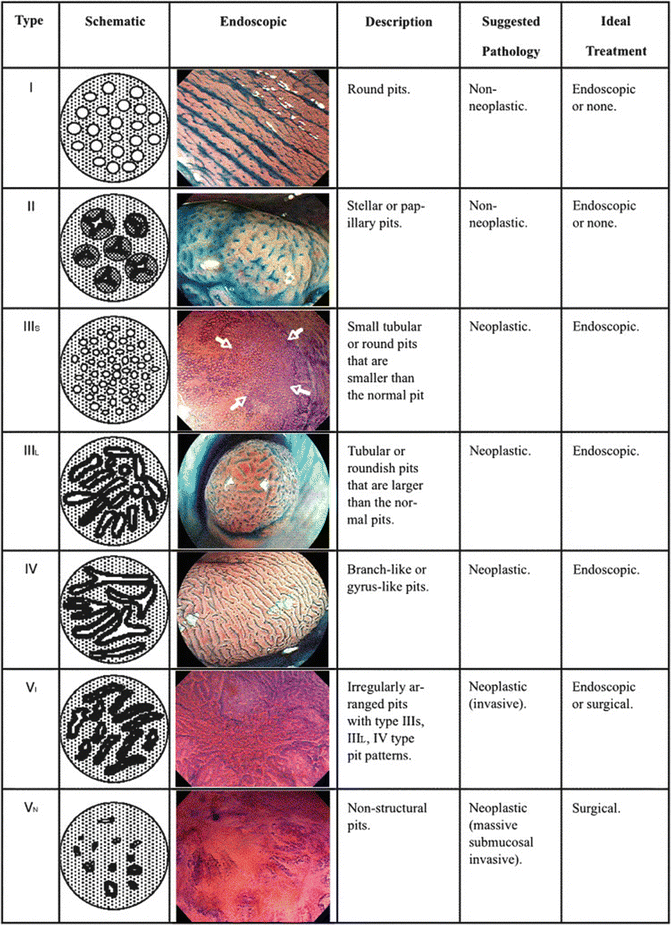
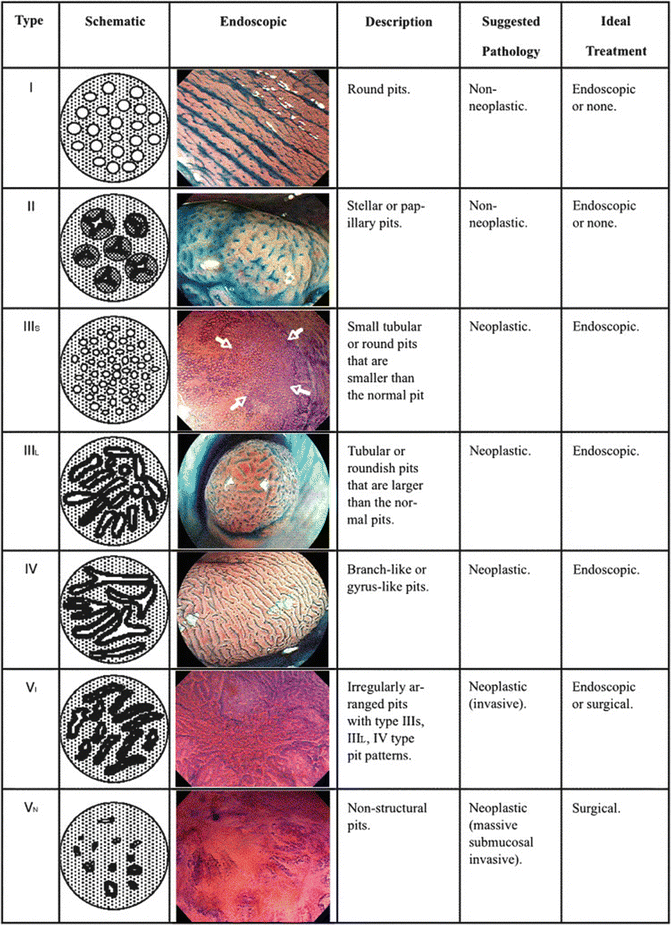
Fig. 5.1
Colonic pit pattern classification. Reproduced with permission, Tanaka GIE 2006;64:604–13
The use of chromoendoscopy has remained useful in regions with high incidences of squamous cell carcinoma (SCC) of the esophagus. Lugol’s solution stains glycogen, which is found within non-keratinized squamous cells, into a dark brown color, allowing delineation of normal epithelium from glycogen-depleted cells (e.g. dysplastic epithelium, SCC, columnar epithelium, or inflammation) [7] (Fig. 5.2).
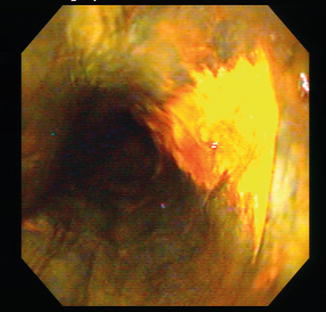
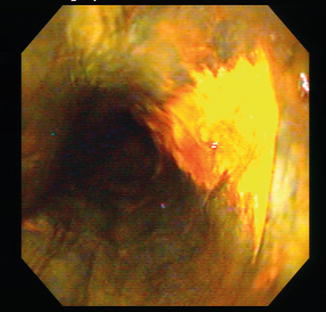
Fig. 5.2
Chromoendoscopy using Lugol’s solution to enhance resection margins of an esophageal squamous cell carcinoma (bright yellow)
The dyes most commonly used for chromoendoscopy in Barrett’s esophagus (BE) are acetic acid and methylene blue. Acetic acid produces reversible changes on the surface proteins of columnar epithelium, causing transient whitening of the epithelium that usually lasts 2–3 min. This whitening effect is lost faster in dysplastic epithelium compared to the surrounding mucosa, therefore enhancing its identification. Methylene blue is absorbed by BE including dysplastic epithelium, but not by squamous epithelium or gastric-type metaplasia in the lower esophagus. However, the absorption is heterogenous and of a lighter intensity in high-grade dysplasia or adenocarcinoma of the esophagus, allowing differentiation from non-dysplastic BE [9].
In contrast, in areas with high incidence of BE, the use of chromoendoscopy has been declining in favor of virtual chromoendoscopy techniques (e.g. NBI or FICE), which have the advantage of providing mucosal enhancement within seconds by simply pushing a bottom within the endoscope and therefore obviating the need to spray and rinse dye. In fact, a recent meta-analysis evaluating the increased diagnostic yield for the detection of dysplasia or cancer on patients with BE found that both chromoendoscopy and virtual chromoendoscopy equally increased the diagnostic yield for dysplasia [1].
In the stomach, chromoendoscopy has long been used to detect intestinal metaplasia, dysplasia, and early gastric cancer. A combination of methylene blue with Congo red or acetic acid has been found useful for detecting early gastric cancer. In one study, the detection of synchronous lesions increased from 28 % under standard white light endoscopy up to 89 % with a combination of methylene blue and Congo red, as cancerous areas do not stain with either dye [7]. Similarly, others have also found increased early gastric cancer detection with a combination of indigo carmine and acetic acid [10, 11]. In one study, the diagnostic yield almost doubled with a combination of indigo carmine and acetic acid (94 %) versus either one alone (42 % for acetic acid and 52 % for indigo carmine; p < 0.005) [10]. In another study, this same combination was able to identify 6 of 39 lesions that were previously missed with standard white light endoscopy and using only indigo carmine [11].
Optical Imaging Technologies
Narrow Band Imaging
Narrow band imaging (NBI) is an optical technique that uses reflected light to visualize the superficial structure and enhances vasculature within the mucosal layer. It selects light within the hemoglobin absorption band, such that blood vessel-rich structures, such as neoplastic lesions, are demonstrated with high contrast relative to their non-neoplastic background.
While conventional white light endoscopy uses the full visible wavelength range (400–700 nm) to produce a red-green-blue image, NBI uses manipulations of the light source (optical) to enhance visualization of the surface. NBI imaging in combination with magnification endoscopy (ME) illuminates the tissue surface using special filters that narrow the red-green-blue bands and simultaneously increase the relative intensity of the blue band [12].
In patients undergoing surveillance for BE, NBI, when compared to standard resolution endoscopy, detected significantly more patients with dysplasia (57 % vs. 43 %) and higher grades of dysplasia (18 % vs. 0 %; p < 0.001), but with fewer biopsy samples (mean 4.7 vs. 8.5; p < 0.001) [13]. Similarly, when compared to high definition white light endoscopy (HDWLE), NBI detected a higher proportion of areas with dysplasia (30 % vs. 21 %, p = 0.01) with fewer biopsies needed per patient (3.6 vs. 7.6, p < 0.0001) [14]. Results from a recent meta analysis support the routine use of NBI for detection of dysplasia and neoplasia during BE surveillance [1] (Fig. 5.3).
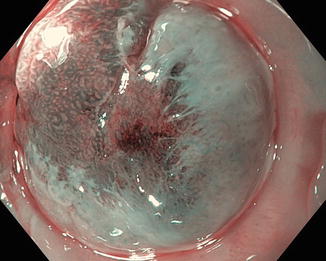
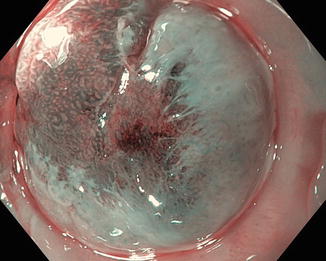
Fig. 5.3
NBI of esophageal nodule in a patient undergoing surveillance for Barrett’s esophagus. Note the loss of all pit pattern (Kudo V). Resection specimen revealed T1a adenocarcinoma
NBI enhancement of microvascular and microsurface pattern changes in the esophageal and gastric mucosa allows differentiation between early cancer and benign lesions [3, 15]. With the use of ME, NBI has allowed identification of specific vascular patterns in the intrapapillary capillary loop of the esophagus which helps recognize early SCC [3].
In addition, ME-NBI has been shown as superior to chromoendoscopy (97.4 % vs. 77.8 %; p = 0.009) in delineating the margins of early gastric cancer [16] (Fig. 5.4). ME-NBI was able to define the entirety of margins in 72.6 % of the lesions that had unclear margins by chromoendoscopy [17].
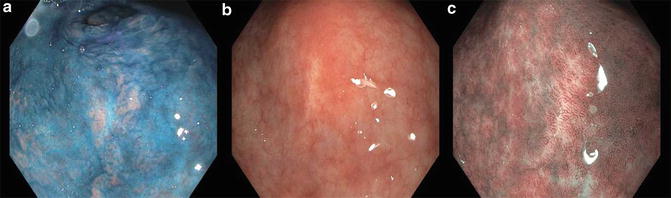
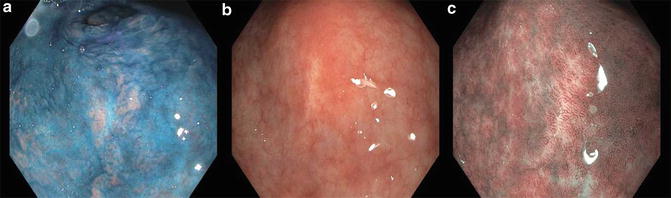
Fig. 5.4
Chromoendoscopy (a), HDWLE (b), and ME-NBI (c) of the same suspicious area found on the lesser curvature of the stomach on a patient with known gastric intestinal metaplasia undergoing surveillance endoscopy
Recently, an NBI international consensus for endoscopic (NICE) classification has been developed with regard to distinguishing hyperplastic, neoplastic, and deeply invasive neoplastic lesions to facilitate selection of lesions for ESD with acceptable accuracy and reliability [18] (Fig. 5.5). For predicting deep submucosal invasion, which is a contraindication to ESD, the sensitivity was 91.8 %, specificity 88.3 %, and negative predictive value 91.9 % for lesions predicted with high confidence. However, only half of the lesions could be predicted with high confidence. Most importantly, this classification has only been validated for colonic lesions [18]. In the upper GI tract there are many proposed classifications [15], but there is a lack of standardization between the described patterns and further research is necessary to reach a consensus.
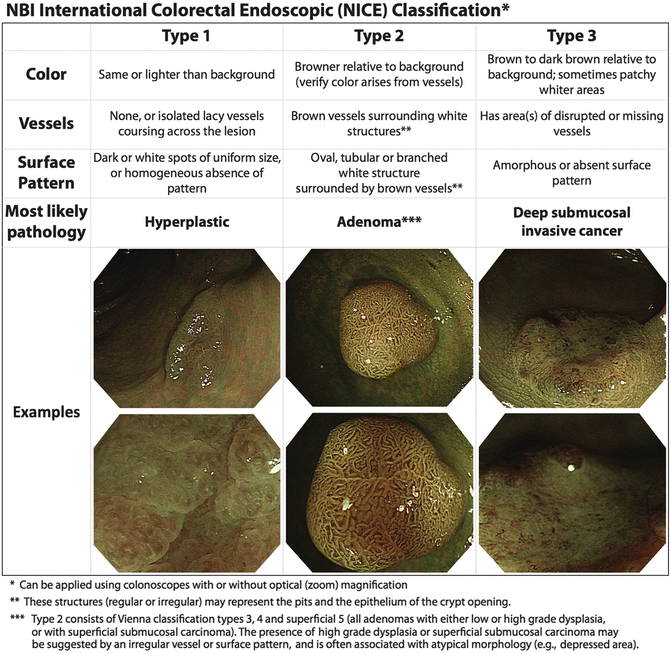
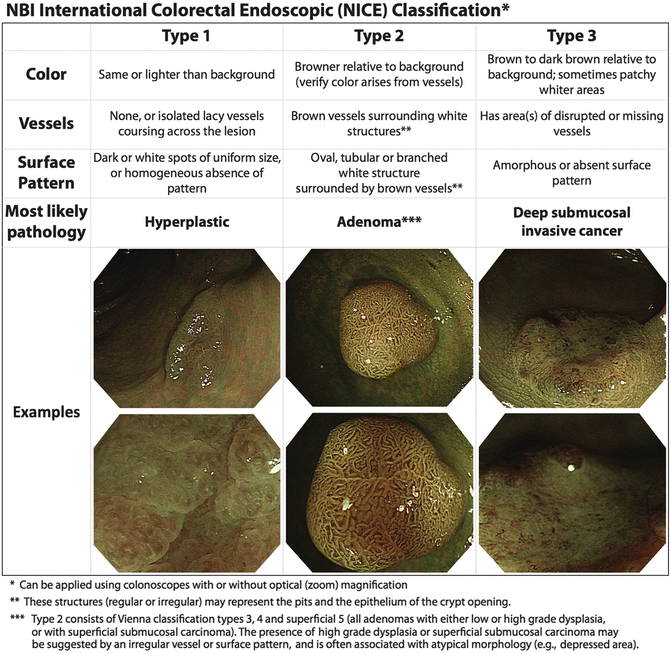
Fig. 5.5
The NBI international colorectal endoscopic (NICE) classification
Autofluorescence Imaging
Autofluorescence imaging (AFI) is a technique based on the principle that excitation of tissue with light of a shorter wavelength leads to emission of a longer wavelength of light. In the gastrointestinal tract, autofluorescence detects subtle changes in the concentration of specific chemicals in the tissue that have the ability to fluoresce when activated by specific wavelengths of light. Malignant transformation of tissue is associated with emission of relatively longer wavelengths of light (shift from green toward the red end of the spectrum) [12].
However, a recent analysis of data from five prospective clinical trials, encompassing close to 400 patients with BE, found little influence of AFI on the diagnosis of early stage neoplasia or on therapeutic decision making. The authors therefore concluded that AFI has a limited role in routine surveillance or management of patients with BE [19].
AFI has also been assessed for detecting early gastric neoplasia in the stomach [20, 21]. The results of a recent trial suggest that AFI can help detect those patients who are most at risk for developing metachronous lesions after ESD of early gastric cancer (hazard ratio 4.88, CI 95 %: 1.32–18.2, p = 0.018) by stratifying the patients by the extent of their intestinal metaplasia. However, there are no trials comparing the added benefit of this technology over more readily available advanced imaging modalities (e.g. NBI, HDWLE, CLE).
Endocytoscopy
Endocytoscopy is a novel technology that allows microscopic imaging of the gastrointestinal surface epithelium. Similar to a contact light microscope, it uses a high-power objective lens to project highly magnified (from 400 to 1,400-fold) images onto the charge-coupled device of the scope processor. It allows real-time magnification of the uppermost epithelial layer, allowing cytological and architectural assessment [22]. Methylene blue is commonly used as a contrast agent. Similar to confocal laser endomicroscopy (CLE), there are two systems developed: endoscope and probe-based. However, neither of these systems is currently available for clinical practice. There are limited data on the use of endocytoscopy in the upper GI tract, and most of the reported studies are pilot studies performed for lesion characterization in the esophagus [22, 23].
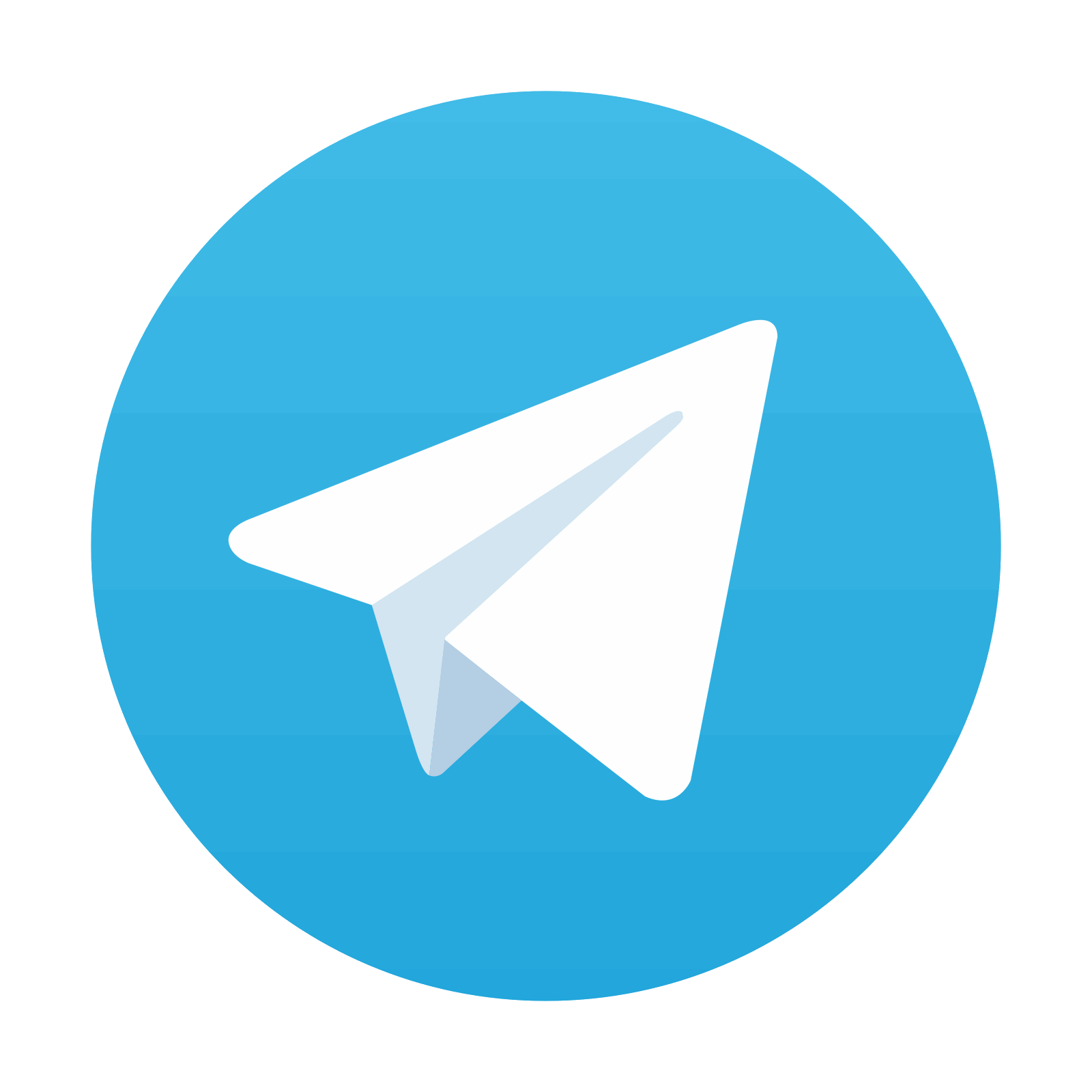
Stay updated, free articles. Join our Telegram channel

Full access? Get Clinical Tree
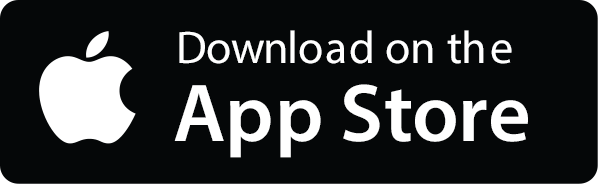
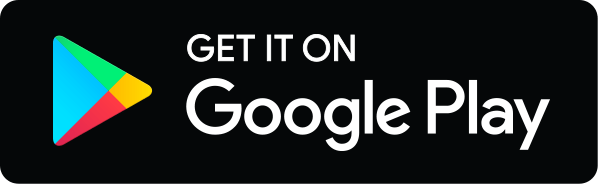