Abstract
This chapter addresses the physiology and regulation of fetal and neonatal acid-base balance at the cellular and organ system levels, with attention to the clinical relevance of the complex interactions between the mother and the fetus ensuring the maintenance of fetal acid-base homeostasis and the physiology of acid-base homeostasis in the neonate following delivery. The physiologic processes regulating the elimination of the acid load by the placenta, the lungs, and the kidneys are reviewed in more detail along with the impact of acid-base disturbance on fetal and postnatal growth. Finally, the effects of selected obstetrical management approaches on fetal and neonatal acid-base balance are addressed.
Keywords
acid-base homeostasis, mother, fetus, neonate, respiratory acidosis, metabolic acidosis, maternal anesthesia
- •
In Addition to the Buffering Systems, Fetal Acid-Base Homeostasis Is Regulated by Maternal Respiratory and Renal Function and the Immature Fetal Kidneys
- •
Fetal Acid-Base Homeostasis Affect Fetal Growth and May Have Long-Term Consequences
- •
Certain Aspects of Obstetrical Management of Labor and Delivery (e.g., Prenatal Steroid Administration or the Practice of Delayed Cord Clamping) and Anesthesia During Labor and Delivery Also Affect, Albeit Transiently, Fetal and Postnatal Acid-Base Homeostasis
Introduction
This chapter addresses the regulation of fetal and neonatal acid-base balance with a focus on the elimination of the acid load by the placenta, lungs, and kidneys, briefly discusses the impact of acid-base disturbance on fetal and postnatal growth, and describes the effect of selected obstetrical management approaches on fetal and neonatal acid-base balance.
Hydrogen ion concentration is tightly regulated by the intracellular and extracellular buffer systems and respiratory and renal compensatory mechanisms. The normal range of hydrogen ion concentration in the extracellular fluid is between 35 and 45 nEq/L (3.5–4.5 × 10 −8 M), which translates to a pH range of 7.35 to 7.45 (pH = −log 10 [H + ]). Under physiologic circumstances, volatile and fixed acids generated by normal metabolism are excreted and the pH remains stable. Carbonic acid is the most common volatile acid produced and is readily excreted by the lungs in the form of carbon dioxide. Fixed acids, such as lactic acid, ketoacids, phosphoric acid, and sulfuric acid, are buffered principally by bicarbonate in the extracellular compartment. The bicarbonate used in this process is then regenerated by the kidneys in a series of transmembrane transport processes linked to the excretion of hydrogen ions in the form of titratable acids (phosphate and sulfate salts) and ammonium. Several aspects of the regulation of acid-base homeostasis are developmentally regulated in the fetus and neonate and thus differ from those in children and adults. These developmentally regulated differences of acid-base homeostasis and their impact on fetal and postnatal growth are reviewed in this chapter.
Regulation of Acid-Base Homeostasis
Respiratory Acidosis
Unlike in fetal respiratory acidosis, in postnatal respiratory acidosis, immediate activation of the pulmonary compensatory mechanism leads to enhanced elimination of carbon dioxide, and the resulting fall in carbon dioxide concentration increases the pH toward normal (pH = [HCO 3 − ] ÷ pCO 2 ; where [HCO 3 − ] = bicarbonate and pCO 2 = carbon dioxide tension). The rapid activation of the respiratory compensatory mechanism is a result of the free movement of carbon dioxide across the blood-brain barrier, leading to instantaneous changes in cerebrospinal fluid (CSF) and cerebral interstitial fluid hydrogen ion concentrations. The degree of maturity of the central respiratory control system and pulmonary function determines the overall effectiveness of the respiratory compensatory mechanism.
Correction of Fetal Respiratory Acidosis
Fetal respiratory acidosis develops when prolonged maternal hypoventilation occurs with maternal asthma, airway obstruction, narcotic overdosing, maternal anesthesia, severe hypokalemia, and magnesium sulfate toxicity. Fetal breathing movements increase, and the fetal kidneys exert a maturation-dependent limited response by reclaiming more bicarbonate in an attempt to restore the physiologic 20 : 1 ratio of bicarbonate to carbonic acid, resulting in a return of the pH toward normal. In the fetus, only the renal compensation has some limited physiologic significance when respiratory acidosis develops due to prolonged maternal hypoventilation. Rather, the placenta, coupled with maternal compensatory mechanisms, performs most of the effective compensatory functions.
Correction of Postnatal Respiratory Acidosis
In the clinical setting, acute neonatal respiratory acidosis develops most frequently in preterm infants with respiratory distress syndrome. Although stimulation of the respiratory center in the brain by the decrease in pH due to the elevated interstitial carbon dioxide concentration immediately increases respiratory rate and depth, carbon dioxide elimination by the lungs is usually limited because of immaturity and parenchymal disease. Importantly, in premature infants, increase in minute ventilation is achieved primarily by the increase in respiratory rate accompanied by only minor changes in tidal volume. On the other hand, term infants respond with increased tidal volume first. Respiratory compensation can be further affected by the decreased carbon dioxide sensitivity of the premature infant and the altered interaction between arterial oxygen tension (pO 2 ) and carbon dioxide sensitivity, particularly at the level of central receptors, during the neonatal period as compared with adults.
As in the fetus, the kidneys reclaim more bicarbonate in response to respiratory acidosis. However, renal compensation is limited by the developmentally regulated immaturity of renal tubular functions, especially during the first few weeks of postnatal life.
Regulation of Acid-Base Homeostasis
Metabolic Acidosis
As in respiratory acidosis, the pulmonary gas exchange serves as the immediate regulator of acid-base homeostasis when metabolic acidosis develops. However, because plasma bicarbonate does not readily cross the blood-brain barrier, CSF bicarbonate levels change only with a delay. Therefore full activation of the respiratory acid-base regulatory system occurs only hours after the development of metabolic acidosis. This is different from the previously described truly immediate activation of the respiratory acid-base regulatory system by respiratory acidosis. In the CSF, generation of bicarbonate via hydroxylation of the dissolved carbon dioxide during CSF formation is the primary source of bicarbonate. On the other hand and as mentioned earlier, changes in arterial carbon dioxide tension rapidly affect carbon dioxide tension in the CSF, even in the setting of primary metabolic acidosis. Changes in CSF carbon dioxide levels also readily affect CSF bicarbonate levels. In addition, the respiratory response to changes in CSF bicarbonate levels in metabolic acidosis appears to be not as robust as that triggered by changes in carbon dioxide tension. Finally, the respiratory compensatory mechanism is complemented by removal of the acid load by the kidneys.
Fetoplacental Elimination of Metabolic Acid Load
Fetal respiratory and renal compensation in response to changes in fetal pH is limited by the level of maturity and the surrounding maternal environment. However, although the placentomaternal unit performs most compensatory functions, the fetal kidneys have some, although limited, ability to contribute to the maintenance of fetal acid-base balance.
The most frequent cause of fetal metabolic acidosis is fetal hypoxemia owing to abnormalities of uteroplacental function or blood flow, or both. Primary maternal hypoxemia or maternal metabolic acidosis secondary to maternal diabetes mellitus, sepsis, or renal tubular abnormalities is an unusual cause of fetal metabolic acidosis.
The pregnant woman, at least in late gestation, maintains a somewhat more alkaline plasma environment compared with that of nonpregnant controls. This pattern of acid-base regulation in pregnant women is present during both resting and after maximal exertion and may serve as a protective mechanism from sudden decreases in fetal pH. Maintenance of the less acidic environment during pregnancy appears to be achieved through reduced plasma carbon dioxide and weak acid concentrations.
The placenta plays an essential role in the maintenance of fetal acid-base balance when metabolic acidosis develops. As mentioned earlier, fetal metabolic acidosis most frequently occurs when abnormal uteroplacental function or blood flow results in fetal hypoxemia. Fetal hypoxemia then causes a shift to anaerobic metabolism and large quantities of lactic acid accumulate. As hydrogen ions are buffered by the extracellular and intracellular buffering systems of the fetus, pH drops as plasma bicarbonate decreases. Because of the unhindered diffusion of carbon dioxide through the placenta, restoration of normal fetal pH initially occurs through elimination of the volatile element of the carbonic acid-bicarbonate system via the maternal lungs. However, as lactate and other fixed acids cross the placenta more slowly, the onset of maternal renal compensation of fetal metabolic acidosis is delayed. In addition, if fetal oxygenation improves, the products of anaerobic metabolism are also metabolized by the fetus.
Because there is no physiologic significance to respiratory compensation of metabolic acidosis in utero, the finding that the respiratory control system in the fetus is much less sensitive to changes in pH than in the neonate has little practical importance. Yet, a decrease in the fetal pH stimulates breathing movements in the fetus.
Finally, as for the role of the fetal kidneys in the maintenance of acid-base balance, available evidence indicates that the fetal kidneys excrete both inorganic and organic acids and are also able to reabsorb bicarbonate. Studies in fetal sheep have found age-dependent increases in glomerular filtration rate (GFR) and urinary titratable acid, ammonium, and net acid excretion. A positive relationship also exists between changes in GFR and bicarbonate, sodium, and chloride excretions. Yet, the adaptive capacity of the fetal kidney to changes in fetal acid-base balance is limited. In fetal sheep the hydrochloric acid infusion-induced metabolic acidosis results in increases in titratable acid, ammonium, and net acid excretion without significant changes in GFR or renal tubular bicarbonate absorption. However, as mentioned earlier, under certain conditions, such as volume depletion or recovery from mild hypocapnic hypoxia, the fetal kidney has the ability to increase bicarbonate reabsorption. It is also important to note that the vast majority of these data have been obtained in animal models and that there is only very limited information available concerning renal acidification by the human fetus. In addition, the physiologic importance of the adaptive fetal renal responses is limited compared with that in the postnatal period because the acid load excreted in the fetal urine remains within the immediate fetal environment and needs to be eliminated by the placenta or metabolized by the fetus.
Indeed, amniotic fluid acid-base status and electrolyte composition have been shown to affect the fetus. When the effects of amnion infusion of physiologic saline with those of lactated Ringer were compared in the fetal sheep, significant increases in fetal plasma sodium and chloride concentrations were noted only in the physiologic saline infusion group. In addition, fetal arterial pH decreased in the physiologic saline group and the change in the fetal pH was directly related to the changes in plasma chloride concentrations. However, despite the significant changes in plasma sodium and chloride concentrations and pH, fetal plasma electrolyte composition and acid-base balance remained in the physiologic range, leaving these findings with little clinical significance.
Postnatal Elimination of Metabolic Acid Load
The most frequent causes of increased anion gap (lactic acid) metabolic acidosis in the neonate are hypoxemia or ischemia secondary to perinatal asphyxia; vasoregulatory disturbances and/or myocardial dysfunction caused by immaturity, sepsis, or asphyxia; severe lung disease with or without pulmonary hypertension; certain types of structural heart disease; and volume depletion. Severe metabolic acidosis caused by a neonatal metabolic disorder is rare but should always be considered. Preterm neonates frequently present with a mild to moderate normal anion gap acidosis, which almost always is the consequence of the low renal bicarbonate threshold of the premature kidney. However, the use of carbonic anhydrase inhibitors and parenteral alimentation, as well as the maturation-related decreased sensitivity to aldosterone, have also been suggested to contribute to the development of normal anion gap acidosis in the neonate.
As mentioned earlier, in metabolic acidosis caused by the accumulation of lactic acid, hydrogen ions are buffered by the intracellular and extracellular buffering systems and plasma bicarbonate concentration decreases and pH drops. Restoration of pH toward normal initially occurs through elimination of the volatile element of the carbonic acid–bicarbonate system via the lungs. This process may be severely compromised in the sick preterm and term neonate with parenchymal lung disease.
The principle mechanism of the renal compensation is the regulation of renal tubular bicarbonate and acid secretion in response to changes in extracellular pH. Although full activation of this system requires at least 2 to 3 days, changes in renal acidification may be seen as early as a few hours following the development of the acid-base disturbance. Although renal compensation is the ultimate mechanism that adjusts the hydrogen ion content of the body, this compensatory function is also affected by the immaturity of the neonatal kidneys. Both renal hemodynamic and tubular epithelial factors play a role in the limited renal compensatory capacity of the newborn.
Renal blood flow (RBF) significantly increases after the immediate postnatal period, and some of the renal vasodilatory mechanisms are functionally mature as early as the 24th week of gestation. Similar to RBF, GFR is also low in the immediate postnatal period and increases as a function of both gestational and postnatal age. Indeed, the low GFR is considered the primary hemodynamic factor limiting the ability of the neonate to adequately handle an acid load.
In addition, net renal acid excretion is regulated by several tubular epithelial functions. In the proximal tubule the following major transport mechanisms regulate active acid extrusion and transepithelial bicarbonate reabsorption: the H + -ATPase, the Na + ,K + -ATPase–driven secondary active Na + /H + antiporter in the apical membrane and the electrogenic Na + /3HCO 3 − cotransporter in the basolateral membrane, and the Na + ,K + -ATPase–driven tertiary active Na + -coupled organic ion transporter. Because approximately 80% of the filtered bicarbonate is reabsorbed in the proximal tubule, the function of these proximal tubular transporters determines the renal threshold for bicarbonate reabsorption. The bicarbonate threshold is approximately 18 mEq/L in the premature and approximately 21 mEq/L in the term infant, and it reaches adult levels (24–26 mEq/L) only after the first postnatal year. However, in the extremely low gestational age neonate the renal bicarbonate threshold may be as low as 14 mEq/L. Because renal carbonic anhydrase is present and active during fetal life and because its activity is similar in the 26-week-old extremely immature neonate to that of the adult, a developmentally regulated immaturity of the function of the previously described proximal tubular transporters is most likely responsible for the low bicarbonate threshold during early development. Indeed, both the activity and the hormonal responsiveness of the proximal tubular Na + ,K + -ATPase are decreased in younger compared with older animals. Moreover, similar to the decrease in bicarbonate reabsorption in the proximal tubule, the activity of Na + /H + antiporter is also decreased to approximately one-third of the adult level.
In addition to immaturity, medications used in critically ill neonates may also affect proximal tubular bicarbonate reabsorption. For example, via inhibition of the proximal tubular Na + /H + antiporter, dopamine may potentially decrease the low bicarbonate threshold of the neonate. Carbonic anhydrase inhibitors also decrease proximal tubular bicarbonate reabsorption by limiting bicarbonate formation and hydrogen ion availability for the Na + /H + antiporter. By acting on several transport proteins along the nephron, furosemide directly increases urinary excretion of titratable acids (phosphate and sulfate salts) and ammonium. On the other hand, by inhibition of the activation of aldosterone receptors, spironolactone indirectly decreases hydrogen ion excretion in the distal tubule.
Under physiologic circumstances, the thick ascending loop of Henle and the distal tubule reabsorb the remaining filtered bicarbonate via transport mechanisms similar to those of the proximal tubule. In addition, by transporting bicarbonate across the membrane, the distal nephron-specific HCO 3 − /Cl − antiporter located in the basolateral membrane contributes to the reabsorption of bicarbonate.
Hydrogen ions are excreted in the urine in the form of titratable acids (phosphate and sulfate salts) and as ammonium salts, which are formed by the combination of hydrogen with ammonia. Because the major constituent of titratable acid in the urine is H 2 PO 4 − , drugs that decrease proximal tubular phosphate reabsorption and thus increase the delivery of phosphate to the distal nephron may increase the renal acidification capacity of the neonate. Indeed, by inhibiting proximal tubular phosphate reabsorption, dopamine has been shown to increase the excretion of titratable acids in preterm infants. Furthermore, net hydrogen ion secretion in the distal nephron continues after the reabsorption of virtually all bicarbonate by active extrusion of hydrogen and via the ability of the distal tubular epithelium to maintain large transepithelial concentration gradients for hydrogen and bicarbonate.
Urinary excretion of titratable acid and ammonium increases as a function of gestational and postnatal age. However, because effective urinary acidification is usually acquired by the age of 1 month, even in premature infants, postnatal distal tubular hydrogen ion secretion is inducible independent of the gestational age at birth.
Aldosterone is one of the most important hormones influencing distal tubular acidification. By affecting the function of several different transport mechanisms, aldosterone stimulates net hydrogen ion excretion in the distal nephron. Of note is that the premature neonate has a developmentally regulated relative insensitivity to aldosterone.
In summary, the renal response to metabolic acidosis in the immediate postnatal period consists of attenuated increases in GFR, proximal tubular bicarbonate reabsorption, and distal tubular net acid secretion. However, a significant improvement in the overall renal response occurs after the first postnatal month even in the premature infant.
Regulation of Acid-Base Homeostasis
Respiratory Alkalosis
Correction of Fetal Respiratory Alkalosis
Rather than causing fetal respiratory alkalosis, acute maternal hyperventilation may lead to the development fetal hypoxia and metabolic acidosis. The fetal acidosis under these circumstances is thought to be the consequence of the acute decrease in placental blood flow caused by the maternal hypocapnia-induced significant uterine vasoconstriction. In these cases, restoration of maternal carbon dioxide levels rapidly corrects both the abnormal uterine blood flow and the acid-base abnormality in the fetus.
The physiologic hyperventilation of the pregnant woman causes a compensatory decrease in her serum bicarbonate concentration to approximately 22 mM without any apparent effect on the fetus (see earlier).
Correction of Postnatal Respiratory Alkalosis
Neonatal respiratory alkalosis occurs most often in the febrile nonventilated neonate or in cases with iatrogenic hyperventilation of the intubated preterm or term infant. Rarely, respiratory alkalosis may be the presenting sign of a urea cycle disorder during the first days of postnatal life because the rising ammonia level may initially stimulate the respiratory center in the brain. As for the renal compensation of respiratory alkalosis, both urinary bicarbonate reabsorption and distal tubular net acid excretion decrease and thus extracellular pH tends to return toward normal. This renal compensation plays an important although somewhat limited role in neonatal respiratory alkalosis.
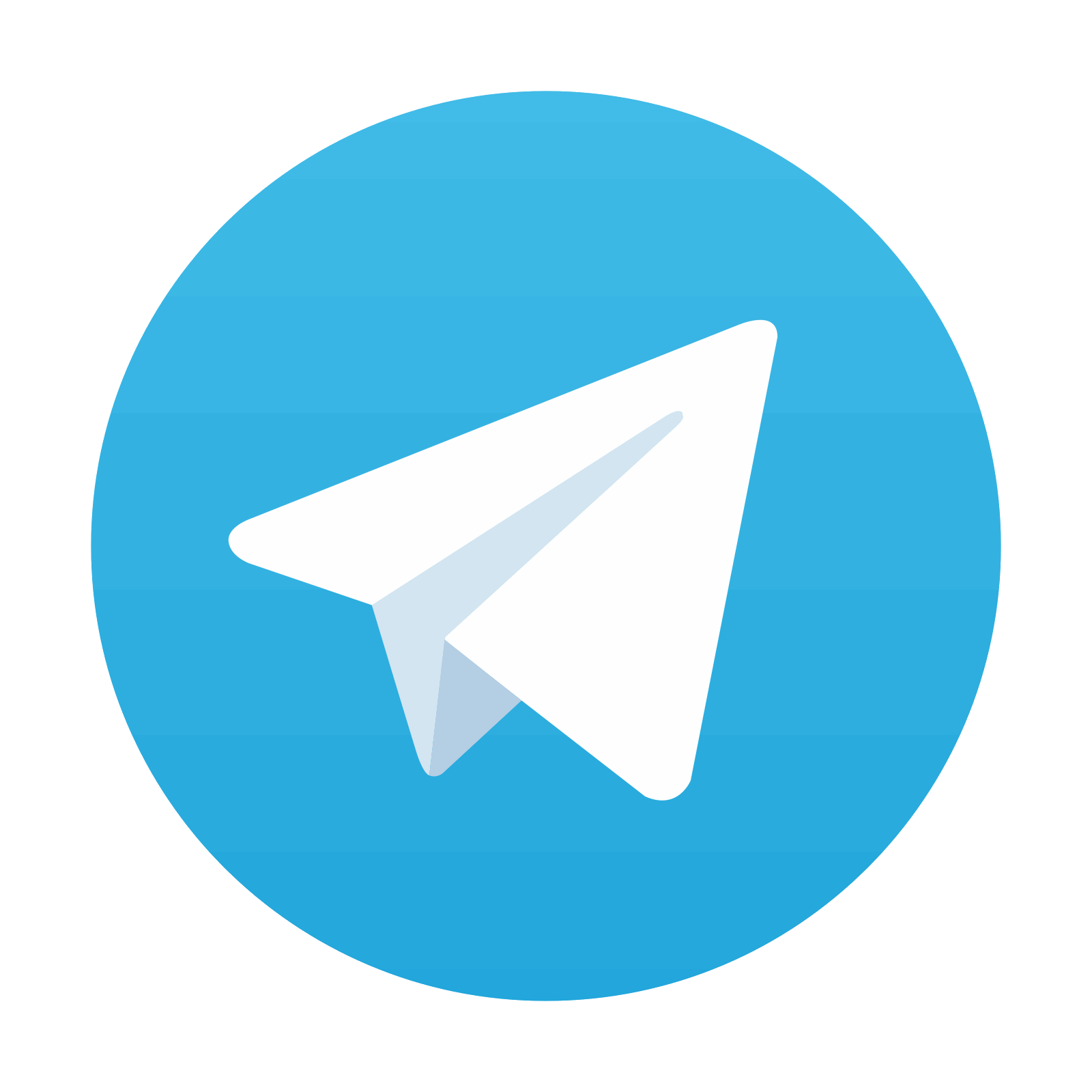
Stay updated, free articles. Join our Telegram channel

Full access? Get Clinical Tree
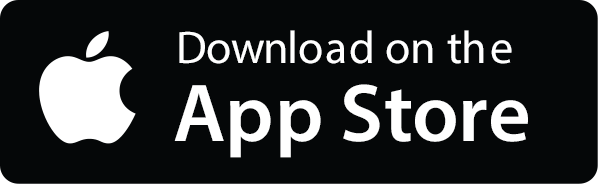
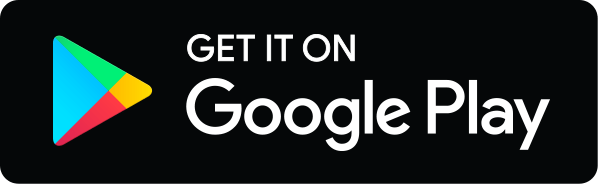