Early in the last century little distinction was made in the care of children from adults. William E. Ladd a general surgeon observed this first hand as he cared for severely burned children in the Halifax disaster of 1917. “Dr Ladd was distressed by the quality of surgical care offered to these small patients and was determined to improve it.”1 He and Dr Robert E. Gross in the mid-20th century pioneered a new field of surgery specific to children, illuminating features of children that merited special attention. They and others recognized that although the care of children shared some similarities to that of the adult, there were distinctly different anatomic, physiologic characteristics, and surgical conditions in children that made them unique. In 1962 the first pediatric trauma unit was opened at the Kings County Hospital Center in Brooklyn. Yet, the lack of dedicated care and clinical protocols persisted in the United States until the early 1970s. The first designated pediatric shock trauma unit in the United States was opened at the Johns Hopkins Children’s Center and became incorporated into the statewide University of Maryland Institute of Emergency Medical Systems.2 In the years that followed, the American College of Surgeons Committee on Trauma established the treatment of pediatric injury as an important component of all regional trauma centers, integrated pediatric trauma into the Advanced Trauma Life Support course, and established minimum requirements for pediatric trauma center designation. By 2010 the number of pediatric trauma centers in the United States grew to 43 but still far less than the 474 adult level I and II centers.
C. Everett Koop, a former pediatric surgeon and US Surgeon General declared, “If a disease were killing our children at the rate unintentional injuries are, the public would be outraged and demand that this killer be stopped.” Worldwide, every year nearly a million children are killed. Nonfatal injuries affect the lives of between 10 million and 30 million more. Each year in the United States, more than 12,000 children die from unintentional injuries and nearly a million children are treated in emergency departments for nonfatal injuries.3 The cost of unintentional injury can be measured in dollars, permanent disability, and potential lost years in productivity or life. Danseco reported that the estimated medical costs of unintentional childhood injuries in the United States were an astounding $347 billion per year. This estimate included $17 billion in medical costs, $72 billion in future work lost, and $257 billion in lost quality of life.4
Injuries are the leading cause of death in the United States for persons aged 1–19 years and the fifth leading cause of death for newborns and infants aged less than 1 year (Fig. 43-1). The death rate for males is almost two times the rate for females. Pediatric trauma patients demonstrate a lower mortality than adults. Similarly, injured children appear at lower risk of post-injury organ failure.5 Children demonstrate differing injury patterns by age and differ from those observed in adults. By adolescence, however, patterns of injury mirror those seen in adults. The mechanisms of injury affecting each age group are largely influenced by developmental stage. Two peaks in deaths are observed; the toddler years (age 1–4 years) and adolescence (15–18 years). Infants are at highest risk of death from inflicted trauma. Strikingly, intentional injury from abusive trauma accounts for more deaths in infants than unintentional injury. Toddlers often suffer injury from falls due to their immature motor and cognitive skills coupled with their higher center of gravity. From ages 5 to 9 years pedestrian injuries are the leading cause of death, reflecting greater mobility and thus, risk. Motor vehicle occupant injuries predominate in the 10- to 14-year age group. Adolescent death is most often due to a motor vehicle crash as the driver or occupant. This age group is particularly at risk of serious injury or death due to greater autonomy, poor judgment, and alcohol or drug use. Injury death rates for teenagers are significantly higher than all other age groups. While penetrating injury is relatively uncommon in young children it becomes more prevalent in adolescence. Homicide is the second leading cause of death in age more than 15 years, but in African-American youths is the leading cause of death. The gun-related homicide rates for African-Americans 15–24 years is double that of Caucasians.6
Thirty percent of children in the United States lack prompt access to pediatric trauma care. Ninety percent of injured children receive care in facilities other than pediatric trauma centers.7 Dictated largely by geography and pediatric specialist limitations most injured children are initially cared for adult specialists with variable pediatric specific training or resources. Therefore, it is incumbent on all providers of trauma care to have knowledge in the unique needs of children, recognizing that children are not just little adults.
The initial evaluation of children does not differ from that in adults. The assessment should proceed in a systematic approach based on the principles of the Advanced Trauma Life Support program published by the American College of Surgeons Committee on Trauma. There are unique characteristics of children that differ from adults that must be considered during the assessment.
The most common cause of preventable prehospital deaths is due to airway obstruction.8,9 Early death (<6 hours) from trauma most often results from lethal CNS injury or hemorrhage.8,10 Prehospital cardiopulmonary resuscitation portends a poor prognosis in the absence of easily reversible airway obstruction, tension pneumothorax, or hemorrhage. In a review of 25 children suffering blunt trauma and requiring prehospital CPR no child survived. Children died predominantly of lethal CNS injury with death from exsanguinating hemorrhage much less common.11 Fallat has suggested that if a child has arrested, resuscitation time exceeds 30 minutes, and the nearest facility is more than 30 minutes away that transport may be futile. Despite current evidence that suggests either death or a poor outcome is inevitable many children undergoing prehospital CPR will still be transported to the nearest hospital.12
Additional factors at presentation alert the physician to a child with life-threatening injury. Low Glasgow Coma Scale (GCS), prehospital intubation, and shock have traditionally been used to identify the high-risk patient. Falcone performed an analysis of 29 commonly used trauma team activation criteria including the six minimum criteria of the American College of Surgeons’ Committee on Trauma (ACS-COT) for highest trauma team activation. The most predictive of requiring a high-level resource were gunshot wound to the abdomen, blood given before arrival, traumatic arrest, tachycardia/poor perfusion, pretrauma center resuscitation with greater than 40 mL/kg, age-appropriate hypotension, airway compromise, and GCS less than 8. Nearly one-half of the injured children who met these criteria needed at least one high-level resource during their ED resuscitation; nearly a fourth required two to three resources. The criteria used for trauma team activation not only identify children at risk of life threatening injury but also those who will likely require one or more emergent interventions.13 It is in this high-risk group that adequate preparation, skills, and age appropriate equipment are essential. Team failure is most likely to occur in high-acuity pediatric trauma activations, those without prior notification, or penetrating injury.14
In young children (<40 kg) the Broselow emergency tape is a useful tool that should at least be available to be used by providers treating injured children, particularly those who treat them infrequently. Not only does the tool guide provide appropriate fluid administration, drug dosing, and normal ranges of vital signs by age, it also guides appropriate size of necessary equipment. The tool has limitations. The length-estimated weight tape may underestimate weight and consequently lead to under dosing of medications. Sinha reported that the Broselow tape underestimated weight on average 2.6 kg with the effect most pronounced in the highest weight category.15 So while it can provide estimates, adjusted dosing or redosing of medications may become necessary.
Ensuring an adequate airway is the first priority in trauma resuscitation. In analysis of preventable deaths in the state of Montana, Esposito found that most of the preventable deaths occurred in the subgroup of children less than or equal to 14 years of age. Inappropriate trauma care was highest in the ED phase of care and primarily resulted from errors in management of the airway.16 Similar findings were reported in a more recent study by Sanddal of preventable pediatric deaths in Utah. Among those patients surviving to a hospital, the preventable death rate was 11%. Issues with care were again mainly in management of the airway, fluid resuscitation, chest injury diagnosis and management.17
Infants and young children have a prominent occiput that tilts the head forward when supine; in combination with a short neck, floppy epiglottis, and increased lymphoid tissue upper airway obstruction is common, particularly in the unconscious child. A simple maneuver, the jaw thrust or chin lift can relieve obstruction caused by the tongue, facilitate bag mask ventilation, and allow time to prepare for endotracheal intubation (ETI). Children have an increased metabolic rate and oxygen consumption. They have diminished functional residual capacity. Together this results in a propensity for rapid oxygen desaturation. With little intrapulmonary oxygen reserve they become hypoxic more precipitously than adults. Supplemental oxygen or oxygen with bag mask ventilation helps to minimize this characteristic of young children.
After simple support of the airway and administration of oxygen the need for intubation should be assessed. In spontaneously breathing children basic airway maneuvers can establish airway patency and restore adequate respirations. Unconsciousness, combativeness, declining GCS, or shock is the most common indication for a definitive airway in an injured child and identifies a seriously injured subset of children with an increased risk of death.18 Nasotracheal intubation is rarely performed in children. The child is preoxygenated, RSI (rapid sequence intubation) drugs administered. ETI generally proceeds orally with direct visualization of the glottis and in line stabilization of the neck. A neck injury should always be assumed to be present. Standard direct laryngoscopy is usually performed.
There has been recent interest in the use of video laryngoscopy (VL, Glidescope) to facilitate successful intubation in the difficult airway. In a meta-analysis of 14 studies by Sun, although VLs improved glottis visualization in pediatric patients but an increase in time to intubation and number of failures was observed.19 The use of VL in trauma remains unproven. In a simulation of pediatric trauma patients with cervical spine immobilization, 23 children age less than 10 years underwent direct laryngoscopy (DL) and VL. The use of the VL resulted in a less optimal view of the glottis and required 21 seconds compared to 7 seconds by DL.20 These data suggest that DL is not inferior to VL when performed by those experienced in endotracheal intubation and caution is warranted before application of VL in the injured child.
The child’s airway is conical rather than cylindrical as in the adult. The narrowest point of the airway is the cricoid cartilage not the vocal cords. Therefore, selection of the correct size endotracheal tube (ETT) is essential to successful intubation. Inappropriate size, multiple attempts can lead to laryngospasm and inability to maintain the airway. Oxygen desaturation and laryngospasm are two of the most common complications observed in attempted endotracheal intubation in children. The third most common complication is right main stem intubation due to the relative short length of the airway. The physician should have some idea of the appropriate depth of the endotracheal tube. A general rule of thumb is three times the diameter of the ETT should be the measurement at the lip indicated by the markings on the ETT.
Rapid sequence intubation is a commonly used adjunct in trauma emergency airway management.21 RSI maximizes the chances for success while minimizing the adverse physiologic effects of intubation.22 The most feared complication of the technique is the inability to intubate and subsequently ventilate the patient. RSI requires familiarity with airway-management techniques, sedation agents, neuromuscular blocking agents, supplemental agents, and postintubation management techniques.23 Children exhibit an exaggerated vagal response to hypoxia, succinylcholine, laryngoscopy, and intubation.24 To attenuate the physiologic effects of ETI especially in children with traumatic brain injury (TBI)25 premedication medications (eg, lidocaine, fentanyl, and atropine) may be administered. A sedative induction agent (eg, thiopental, ketamine, propofol, fentanyl, or etomidate) is given, followed by a rapidly acting neuromuscular blocking agent (eg, succinylcholine), resulting in unconsciousness and motor paralysis.
Cricoid pressure is applied and intubation follows. Over compression can easily occur due to the compressibility of the pediatric airway. Once the ETT is placed a colorimetric device that measures exhaled CO2 is connected. A color change from purple (EtCO2 <0.5%) to yellow (EtCO2 >4%), symmetric chest rise, and auscultation of bilateral breath sounds suggest successful intubation. In the child suffering circulatory arrest a color change may not be observed until the circulation is restored.
If ETI is unsuccessful there are several rescue maneuvers available. The first and perhaps easiest is the placement of a laryngeal mask airway (LMA) designed for use during anesthesia. The LMA is easy to insert, requires less training than ETI but does not protect against aspiration. Successful use after failed emergency ETI has been reported.26 When basic interventions or ETI fail, particularly in the paralyzed patient an emergent surgical airway becomes necessary. Cricothyroidotomy, needle cricothyroidotomy, or translaryngeal jet ventilation offer the last options in securing the airway. While there is ample literature concerning these techniques in adults, their use in children has been limited emphasizing the importance of expertise in emergency pediatric airway management.27
The first step in assessment of breathing is the rate and quality of respirations. The infant may breathe normally up to 60 times per minute, young children up to 40 times per minute, and older children 20–30 times per minute. Young children primarily use the diaphragm to breathe; they have undeveloped musculature rendering them prone to muscle fatigue and less likely able to maintain a sustained high respiratory rate. Ineffective movement of air may result from poor effort, parenchymal injury, pneumothorax, or airway obstruction. Signs of respiratory distress include stridor, chest retractions, paradoxical motion of the diaphragm, and grunting. When these signs are present, support of respiration becomes necessary.
Pulse oximetry is a noninvasive measure of hemoglobin saturation that correlates well with arterial Pao2 and is a measure of blood oxygen saturation. At readings of less than 80% correlation with Pao2 is lost and severe hypoxemia may be present. Oximetry can provide indirect evidence of ventilation but objective evaluation requires measurement of arterial Pco2 or EtCO2. If it is determined that ventilation is inadequate it becomes necessary to give supplement breaths or assume all ventilation. Breaths should be delivered every 2–3 seconds with adequate chest rise. Full ventilation will require adequate sedation and often paralysis. For mechanical ventilation tidal volume of 6–8 mL/kg is used to reduce the risk of barotrauma and the Fio2 titrated to below 60% to minimize the harmful effects of hyperoxemia. Measurement of end tidal capnography is useful to guide the rate of assisted breaths. Significant hypocarbia is deleterious in the brain-injured patient due to the effects on cerebral circulation. Current guidelines suggest that an EtCO2 of 35–40 is optimal.28 Excessive ventilation with low EtCO2 can be minimized with capnography.29 Inadequate ventilation or high peak inspiratory pressure (>30 mm Hg) with ventilation may be due to unrecognized pneumothorax. In this setting evidence of pneumothorax requires rapid decompression with chest tube placement. The Broselow tape or palpation of the intercostal space can guide selection of the appropriate size chest tube. In the absence of pneumothorax/hemothorax significant pulmonary contusion or aspiration may account for the impaired ventilation but the treatment remains largely supportive.
The child’s chest is highly compliant, poorly muscularized, and the ribs less ossified leaving the intrathoracic contents susceptible to injury. External signs of trauma may be absent or minimal. The mediastinum is mobile and pneumothorax can rapidly deteriorate to tension pneumothorax especially if positive pressure ventilation is required. Rib fractures are uncommon in blunt injured children for the aforementioned reasons. Rib fracture(s) suggest a significant blunt force injury. Kessel found that children with rib fracture were more likely than adults with rib fracture to suffer brain injury, hemothorax/pneumothorax, and spleen, and liver injury.30
Ventilation may also be compromised by inadequate gastric decompression. The distended stomach interferes with diaphragmatic excursion, especially in the very young (Fig. 43-2). Therefore, an orogastric tube should be placed in any injured child requiring prolonged bag mask ventilation or endotracheal intubation. A nasogastric tube should not be placed in any child with signs of basilar skull fracture or facial trauma, so a policy of orogastric tube placement simplifies decision-making and reduces the risk of malplacement.
Infants and young children have a limited ability to meet increased cardiac output demand. Stoke volume is generally fixed. Thus, an increase in cardiac output requires an increase in heart rate. Compensation for the loss of intravascular volume results in an increase in both heart rate and systemic vascular resistance with shunting of blood to vital organs. In a classic experiment of hemorrhagic shock, puppies were subjected to continuous hemorrhage calculated at 1% blood volume/minute. The response to hemorrhage was gradual and compensated until nearly a third of the blood volume was lost. With continued blood loss the fall in mean arterial pressure and cardiac output accelerated followed by death.31 Children have robust compensatory mechanisms for mild to moderate shock. But once a critical threshold is passed decompensation occurs rapidly emphasizing the need for early recognition.
Assessment of the circulation begins with the examination of the level of consciousness, skin color, heart rate, blood pressure, and peripheral pulses. An adequate blood pressure may be falsely misleading. The assessment of capillary refill allows an estimate of the degree of vasoconstriction and compensation present. Delayed (>2 seconds) capillary refill in the absence of hypothermia suggests that blood pressure is maintained by an increase in vascular resistance and that the child is significantly hypovolemic. A rapid assessment should be made for any external signs of bleeding that can be controlled by direct pressure or a tourniquet. The efficacy of tourniquets to control blood loss in the military is well described, however, less is known of their efficacy in children.32 Based on the military experience in both adults and children, if there is major hemorrhage from an extremity or amputation application of a tourniquet should be considered. The pelvis should be examined for stability. If a pelvic fracture is suspected in conjunction with signs of shock the pelvis should be wrapped in a sheet to provide compression that can reduce ongoing bleeding.
Signs of decompensated shock in children include altered mental status, mottled or cyanotic skin, cool pale extremities, weak peripheral pulses, and delayed capillary refill. Review of age based normative values for heart rate and blood pressure demonstrates changes that occur from infancy to adolescence (Table 43-1). In general, respiratory rate and heart rate are normally higher, while blood pressure is lower in the young but with aging they approach the normative values of the adult by age 12 years. Mortality is increased in children suffering traumatic injury and hypotension. Gunst reported 2% mortality in hypotensive injured children.33 Hypotension, even brief episodes, substantially increases mortality in children suffering traumatic brain injury.34,35
Age group (years) | Weight range (kg) | Heart rate (beats/min) | Blood pressure (mm Hg) | Respiratory rate (breaths/min) | Urinary output (mL/(kg h)) |
---|---|---|---|---|---|
Infant (0–1) | 0–10 | <160 | >60 | <60 | 2.0 |
Toddler (1–3) | 10–14 | <150 | >70 | <40 | 1.5 |
Preschool (3–5) | 14–18 | <140 | >75 | <35 | 1.0 |
School age (6–12) | 18–36 | <120 | >80 | <30 | 1.0 |
Adolescent (>12) | 36–70 | <100 | >90 | <30 | 0.5 |
Injured children generally suffer one of three types of shock: hemorrhagic, cardiogenic, or distributive. Hemorrhagic and distributive shock is the most common; cardiogenic shock is rarely the cause of primary traumatic shock in children and if present suggests that death is imminent. While hemorrhage should always be assumed as the source of traumatic shock, it must be remembered that severe CNS injury in children can produce hypotension in the absence of hemorrhage.36 A simple calculation, the Shock index (HR/SBP) can quantify the degree of shock; stratify the risk of death, and direct triage of injured patients to a trauma center. Cannon demonstrated in adults that the risk of death is 16% when the SI greater than 0.9 compared to a 6% risk of death when the SI less than 0.9. An increase in SI from the field to the ED nearly doubles mortality.37 The applicability of an uncorrected adult SI to children is limited given the relatively high values of normal heart rate and low values of normal of systolic blood pressure in children relative to adults leading to a falsely lowered SI (<0.9). In an effort to correct for age based normative values, Acker examined an age adjusted shock index (SIPA). They found that similar to observations in adults once SI is adjusted for age, SIPA identifies injured children with high injury severity, blood transfusion within the first 24 hours, high-grade solid organ injury requiring blood transfusion, and increased in-hospital mortality.38
Among the most difficult challenges in the care of children is vascular access. Smaller veins coupled with increased subcutaneous fat in children make both palpation and visualization of veins more difficult. Peripheral vein collapse due to hypovolemia, vasoconstriction from hypothermia, failed attempts with resultant hematoma or bruising, and extremity fractures all further complicate attempts at successful peripheral IV access. Ideally access is obtained with placement of two peripheral IV catheters in the upper extremities. The Broselow emergency tape guides selection of an appropriate IV catheter size. What may appear to be an inadequate catheter size in an adult may actually be suitable for rapid infusion in a child. The saphenous vein at the ankle is an excellent option for peripheral IV access if attempts in the upper extremity fail.
Pediatric Advanced Life Support (PALS) and Advanced Trauma Life Support (ATLS) now recommend placement of an intraosseous (IO) line if IV access cannot be established within three attempts or 90 seconds, whichever is sooner.39 In children IO placement is generally first attempted in the proximal tibia. Other potential sites include the distal tibia, the proximal humerus, and the distal femur. IO avoids many of the problems associated with central venous access. IO placement with a battery-operated device (eg, EZ IO) is rapid, requires little training, and provides reliable access in which most drugs, fluids, and blood achieve the central circulation in seconds (Fig. 43-3). Complications are low but IO placement should be avoided in a fractured extremity due to the risk of fluid extravasation and resultant compartment syndrome.
FIGURE 43-3
(A, B) Placement of intraosseous catheter in the proximal tibia, (C) EZ IO.39 (Reproduced with permission from Ramaiah R, Sharar S. Anesthetic management of the pediatric trauma patient. In: Varon AJ, Smith CE, eds. Essentials of Trauma Anesthesia. Copyright © Cambridge University Press 2012.)



Historically, if peripheral venous access failed then central venous access using the Seldinger technique based on anatomic landmarks was attempted. This practice has largely been abandoned but remains an option when all other measures fail. Usually attempts are made to access the femoral vein first followed by the internal jugular vein or subclavian vein. Ultrasound is readily available in most emergency departments (ED), is easy to learn, and permits visualization of the femoral vein or internal jugular vein facilitating central venous catheter placement. In a randomized trial of children undergoing planned central venous access ultrasound reduced the number of cannulation attempts with a greater success rate relative to the landmark approach.40 Regardless, central venous access carries the risk of serious complication, delay, and because of the suboptimal conditions in the ED requires early catheter removal to avoid central line associated blood stream infection. Therefore, central venous access should only be considered if peripheral IV and IO have failed.
Once IV access is obtained, a 20 mL/kg bolus of 0.9% NS or LR is administered. Due to the risk of nonanion gap metabolic acidosis, LR is favored over 0.9% NS. In the brain injured early administration of hypertonic saline 3% or 7.5% rapidly restores blood volume and may be beneficial in the treatment of traumatic brain injury (TBI).41 However, two recent randomized trials in patients greater than 15 years old failed to demonstrate any benefit of prehospital HTS over 0.9% NS in either patients with hypovolemic shock (SBP <70 or SBP 71–90 and HR >108)42 or TBI (GCS <8).43 Colloid solutions for the resuscitation of traumatic shock fail to demonstrate consistent benefit. A meta-analysis by the Cochrane Review found no evidence from randomized controlled trials that resuscitation with colloids reduces risk of death compared to resuscitation with crystalloids in patients with trauma. Moreover, the use of the colloid, hydroxyethyl starch may increase mortality.44 For now it appears that 0.9% NS or LR should remain the initial resuscitative fluid unless blood transfusion becomes necessary. If the patient does not respond or only transiently responds a second 20 mL/kg bolus of isotonic crystalloid should be given. Blood samples for arterial blood gas, lactate, hemoglobin, coagulation (TEG or PT/PTT), and type and cross should be obtained.
Under or over fluid resuscitation is likely deleterious in trauma patients. The optimal amount of fluid needed in the first 24 hours to adequately resuscitate injured patients remains unclear. However, most patients dying of shock or TBI die within 24 hours from either hemorrhage or lethal brain injury. Most deaths occur despite ongoing resuscitation.45 In adults, Glue grant investigators found that ventilator days, ICU and hospital LOS, risk of acute respiratory distress syndrome, multiple organ failure, bloodstream and surgical site infections, and abdominal/extremity compartment syndromes were all increased in a dose-dependent fashion with aggressive crystalloid administration.46 In contrast, Acker reported that high volume early resuscitation in children, defined as greater than 60 mL/kg/24 hours, did not demonstrate an increased risk of ARDS, MOF, abdominal compartment syndrome, or blood stream infections but like the Glue grant investigators observed an increase in ventilator days, ICU LOS, anemia, and thrombocytopenia.47 Edwards reported similar findings to both studies in a cohort of pediatric combat causalities.48 They observed that the adverse effects of crystalloid became most pronounced when greater than 150 mL/kg was administered in the first 24 hours but 50–150 mL/kg was not associated with increased mortality. Not surprisingly, under resuscitation (<50 mL/kg/24 hours) led to increased mortality but this may simply reflect survival bias; that is children who died prior to hemorrhage control and ongoing fluid resuscitation. Injured children should be judiciously resuscitated but adequate fluid should be given to ensure adequate tissue oxygenation and cerebral perfusion regardless of the amount of crystalloid needed in the early resuscitative phase. While adult studies have suggested benefit to permissive hypotension, Hughes warns that there is no evidence to support permissive hypotension strategies in pediatrics.49
In the absence of a sustained response to 40 mL/kg isotonic crystalloid fluid bolus then ATLS advises the administration of blood. Blood component administration is the resuscitative fluid of choice in the bleeding patient until hemorrhage control is achieved. The decision to begin blood transfusion must be balanced against its potential delayed adverse effects50,51 including a potential negative effect on outcome in children suffering TBI.52 Injured children requiring early blood administration represent a seriously injured subset. Early blood transfusion (<6 hours) is associated with up to 50% mortality in stark contrast to the low mortality in those who do not receive blood or undergo delayed blood transfusion.51 Interestingly, the majority of children who received early blood in this series died of lethal CNS injury rather than hemorrhage. It may be that the brain-injured child in shock and without hemorrhage might benefit from an alternate resuscitative strategy utilizing vasopressors as suggested by Di Gennaro.53
An ongoing requirement for blood suggests incompressible intracavitary hemorrhage and massive transfusion becomes more likely. FAST examination demonstrating free fluid can assist in directing the child to emergent laparotomy to achieve hemorrhage control.54 Like traumatic shock, the need for massive transfusion is associated with an increased risk of death in children. Neff found in combat injured children that once a threshold of 40 mL/kg/24 hours of blood products is given critically injured children are greater than 2.5 times more likely to suffer both early and in-hospital death.55 Similar findings are reported in civilian pediatric victims.51 Current evidence in adults suggests that in this setting blood components be transfused in 1:1:1 or 1:2:1 (pRBC, FFP, platelets) until hemorrhage is controlled and there is no evidence of ongoing bleeding.56 In contrast, a study of pediatric casualties in Iraq and Afghanistan, Edwards found that balanced component resuscitation did not improve outcomes and was associated with higher mortality when all transfused patients were considered.48 Further studies are needed to reconcile this apparent difference in children from adults.49,57
The decision to transfuse blood is based on vital signs, injury patterns, anticipated major bleeding, or risk of continued bleeding after surgical control. The Assessment of Blood Consumption (ABC) score consists of four variables (HR >120, SBP <90, + FAST, penetrating torso injury) each assigned one point. Validated in adults, a score of greater than 2 triggers MTP and correctly classified most bleeding adult patients in need of MTP.58 Sisak found in a prospective study of injured adults that 9% required early blood transfusion and nearly half had activation of a massive transfusion protocol. Injury pattern, vital signs, lactate, and anticipated major bleeding were the best predictors of MTP in this cohort that demonstrated a high rate of emergency surgery (86%) and mortality (14%).59 Less is known in children but the ABC score is not applicable in children due to differing normal values of HR/BP with age. In children most protocols recommend that MTP be activated in children with signs of bleeding. Criteria may include greater than 20 mL/kg pRBC in the first hour, anticipated blood loss greater than one-half blood volume in 12 hours, or greater than one blood volume in 24 hours. While it is evident that MTP results in earlier delivery of blood components, it remains unclear if they improve outcome in children.57,60,61
In the child who presents with signs of life and loses vital signs while in the ED resuscitative thoracotomy may be considered. First, reversible causes of pulseless electrically activity like tension pneumothorax or hypovolemia should be excluded. Unfortunately, the outcome in blunt injured children suffering cardiac arrest and EDT is dismal.62 The results are more favorable in patients suffering penetrating injury and witnessed cardiac arrest, particularly those suffering stab wound to the heart63 EDT may be warranted in children suffering penetrating torso injury who lose vital signs in the ED or have short transport time with prehospital CPR less than 15 minutes.
The child’s level of consciousness, pupillary examination, and neurologic exam defines disability. The aim of the neurological assessment is to identify severe head injury or spinal cord injury that may require urgent neurosurgical intervention. A rapid assessment can be determined using the Alert/Verbal/Pain/Unconscious (AVPU) scale. AVPU appears to correlate well with the Glasgow Coma Scale: Alert—GCS 15 (IQR 15), verbal—GCS 13 (IQR 12–14), pain—GCS 8 (IQR 7–9), and unconscious—GCS 3 (IQR 3).64 Glasgow Coma Scale is more typically used and shows good performance in preverbal children compared to older children with blunt head injury. Receiver operator characteristic curves demonstrate good correlation of GCS with brain injury confirmed by CT or requiring acute intervention.65 Concerns remain that calculation of the GCS can be difficult in children, is compromised by the need for ETI, and suffers significant inter-rater variability. Ultimately, the goal is to ascertain quickly what children require urgent CT scan and perhaps neurosurgical intervention. This has led others to examine the use of abbreviated GCS or one of the three components of the GCS to rapidly identify at risk children.66,67 Acker found that the motor component of the GCS alone identifies children with serious TBI and the elimination of the eye; verbal components did not adversely affect the performance of the tool.67
If after assessment by one of the above tools the child is felt to have a serious brain injury, the eye examination should be repeated to determine if there is evidence of impending transtentorial brain herniation suggested by unilateral dilation of a pupil. This or rapid progressive neurologic deterioration implies critical intracranial hypertension. If present, temporizing measures such as brief hyperventilation (EtCO2 25–30 mm Hg), 3% HTS IV bolus (1–6 mL/kg), or IV mannitol (0.25–1 mg/kg) should be considered until definitive neurosurgical care is available.68 Mannitol should not be administered to a child with hypotension or shock. Unlike mannitol HTS, a volume expander, can be used in the setting of hypotension. The child is examined for evidence of spinal cord injury. Respiratory failure and/or shock can be the first signs of spinal cord injury but are only attributed to spinal cord injury once all other potential causes are excluded. Symmetric flaccid paralysis of the extremities indicates spinal cord injury and spinal immobilization should continue.
Children are susceptible to hypothermia, more so than adults. Hypothermia can lead to arrhythmias, abnormal coagulation69 and metabolic acidosis; the latter two drivers in the lethal triad of trauma death. Review of the National Trauma Bank database (NTDB) demonstrated that of those trauma patients in whom temperature was measured that 2% had a core temperature as low as 32°C. Comparison of core temperature versus mortality revealed that as temperature decreased, the mortality rate increased, reaching approximately 39% at 36°C.70
The trauma room temperature should be maintained greater than 80°F. Wet clothes should be removed, the child covered with warm blankets, and exposures for procedures minimized. All IV fluids and blood products should be warmed using fluid warmers. Active external rewarming with a convective air blanket (eg, Bair Hugger) should be initiated when the core temperature falls below 36°C. Despite the importance of exposure and environmental control during pediatric trauma resuscitation, compliance is poor. In children with TBI or requiring ETI where the duration of exposure is prolonged Kelleher found hypothermia common.71 Sundberg reminds us that a hypothermic patient is 9.2 times more likely to die than a normothermic patient when adjusting for seasonal variation.72 Therefore, children who are prone to hypothermia or arrive hypothermic must be rewarmed and kept warm. There is no role for hypothermia in the acute setting of trauma despite reports of hypothermia used in cardiac arrest or management of traumatic brain injury.
The secondary assessment begins only after all elements of the primary assessment are satisfied. Periodic review of vital signs, pulse oximetry, EtCO2 capnography, fluid administration, and GCS alerts the surgeon to significant change and the need to interrupt the secondary assessment. Chest and pelvis radiographs are obtained if indicated. The examination generally proceeds systematically from head to toe as in adults. At this time a brief medical history, current medications, and immunization status are obtained. For penetrating wounds it may be necessary to administer tetanus toxoid and/or immunoglobulin depending on the immunization status. At this point, if the child is hemodynamically stable with evidence of external injury or in pain an analgesic should be given that will address the pain and facilitate the remainder of the examination. In children, pain is considered the fifth vital sign but is often overlooked.73
Bleeding from scalp lacerations should be controlled with pressure, surgical staples, or injection of local anesthetic containing epinephrine. Due to the frequency of head injury in children, the child should be examined for bleeding from the ear, bruising posterior to the ear, or orbital ecchymosis; findings that suggest basilar skull fracture. The chest is examined for crepitus, rib fractures, bruising or penetrating wounds, and the chest auscultated. One or more of the following in children increases the odds of significant chest injury following blunt trauma: low systolic blood pressure, elevated respiratory rate, abnormal thoracic examination, abnormal chest auscultation, femur fracture, and a GCS score of less than 15.74 If there is evidence of pneumothorax tube thoracostomy is performed.
Increased risk of abdominal injury in children is present when examination reveals: low systolic blood pressure, abdominal tenderness, or femur fracture. Abdominal tenderness confers a sixfold increased risk of significant risk intra-abdominal injury75 but the reliability of absent abdominal tenderness to exclude intra-abdominal injury decreases with declining GCS.76 Another important finding in children is bruising of the abdomen from either a seatbelt77 or handle bar.78 When present, they should raise concern for a significant intra-abdominal injury. Lutz reported among restrained children involved in a motor vehicle crash that those with a bruise were 232 times more likely to have a significant intra-abdominal injury when compared with to children without bruising of the abdominal wall.79 Pelvic fracture is uncommon in children80 nevertheless the pelvis should be palpated for tenderness and instability. If either is present an x-ray should be obtained. However, the routine use of pelvic x-ray in the evaluation of blunt injured children is discouraged.81,82 As in adults, if a pelvic fracture is present in males the meatus and in females the vaginal introitus should be examined for blood. In postmenarche females a urine pregnancy test should be obtained to identify an unknown pregnancy and the potential for fetal compromise. Routine rectal examination, like routine urinary catheterization is not indicated in children.83
When examining the extremities it is important to remember that children may have fractures with minimal displacement, known as greenstick fractures. Tenderness with or without hematoma, bruising, displacement should prompt imaging. For displaced fractures, the pulses should be assessed distally, and the fractured extremity splinted to reduce pain and bleeding. It may be necessary to attempt reduction to restore flow if a distal pulse is absent.
FAST examination, although a diagnostic test, is considered a component of the secondary assessment. In special circumstances it may be performed earlier, particularly in the child in cardiac arrest or profound shock. In this situation, FAST permits examination of the heart and abdomen that can direct emergent interventions or discontinuation of resuscitative efforts if cardiac activity is absent. In stable children, the FAST cannot be solely relied upon to rule-out intra-abdominal injury due to its low sensitivity.84 Thirty to 50% of children with negative FAST will have a solid organ injury.54,84,85 In general, the presence of free fluid on FAST is an indication for CT imaging in the stable child. Some believe that the utility of FAST to direct CT imaging can be improved if integrated with additional supportive findings, like elevated liver transaminases86 a scoring system87 or perhaps a step-up approach where imaging is only indicated if additional clinical factors are present.88 Until these novel approaches that combine FAST with adjunctive findings, tests, or as an element of a scoring scale are validated, FAST should only be relied upon to direct intervention in a child with shock.
The utility of routine laboratory studies in the evaluation of trauma patients to aid in diagnosis of injury is limited and has been largely abandoned. The tests rarely result in a change in management and add unnecessary cost to care.89 Capraro showed that in children with confirmed abdominal injury by CT that no routine laboratory test demonstrated good sensitivity, specificity, PPV, or NPV; leading the authors to conclude that routine “trauma panels” are not indicated as a screening tool in children with blunt trauma.90 Selective testing for suspected injury to the liver (AST), pancreas (lipase), or kidney (urinalysis) is not unreasonable but may lead to unnecessary CT imaging that only reveals injuries not requiring surgical intervention.91 Laboratory studies are most helpful in assessing the metabolic state of the patient92 degree of acute blood loss anemia, and degree of trauma induced coagulopathy. Keller found that over half the children with severe TBI (GCS 3–8) had abnormal coagulation studies but few needed an intervention.93 Arterial blood sampling provides valuable information regarding the metabolic condition of the critically injured patient. The degree of blood loss or the need for blood transfusion can be aided by hematocrit measurement94 although acute values may be falsely elevated.59 Serum lactate, a byproduct of anaerobic metabolism, is useful to quantify the degree of metabolic derangement, is prognostic, and can guide resuscitation.95
The Environmental Protection Agency reports that nearly half of all radiation in the United States is attributable to medical imaging (Fig. 43-4). The overall average radiation of the US population has doubled in the past 25 years and is largely due to increased utilization of diagnostic CT scanning.96 The radiosensitive pediatric population is most at risk. Brenner predicted that while pediatric patients only represented 4% of all CT imaging, will account for 20% of all CT related cancer deaths.97 In 2013, Miglioretti quantified the risk of developing a future malignancy based on a child’s age at the time of the CT scan. He estimated that one in 570–6130 scans will lead to the development of a new cancer depending on the child’s age, sex, and the type of scan.98 In 2007, CT imaging was predicted to cause 29,000 new cases of cancer of which 15% would result from imaging children. The theoretic prediction of cancer from CT imaging has been validated. A linear relationship between the dose of radiation delivered during a CT scan and the relative risk of developing leukemia and brain cancer was reported in a large cohort of European children.99 Similar findings were reported in a study of Australian children in 2013.100 While the estimated future risk of cancer is low, these studies represent only a relatively short period of observation following exposure. Hunter Moore speculated that if real, late deaths due the effects of ionizing radiation could emerge as the fourth peak in trauma related mortality, albeit many years later (Fig. 43-5).101
Given our increasing knowledge of the risks associated with radiation, a CT scan should only be ordered when the results are likely to impact the care of the patient. To raise awareness and reduce radiation dosing during pediatric medical examination the Alliance for Radiation Safety in Pediatric Imaging launched the Image Gently campaign in 2008. These and other efforts are intended to decrease the amount of radiation from imaging through specific steps102 or by eliminating unnecessary imaging. Among the “five things that physicians and patients should question” the American College of Surgeons advises to avoid the routine use of “whole-body” diagnostic computed tomography (CT) scanning in patients with minor or single system trauma. Clarifying which patients can safely forgo CT scans to minimize radiation exposure without missing clinically important injuries is critical. Children who suffer blunt trauma and have a normal chest radiograph do not benefit from chest CT.103 Great vessel injury is rare in children and typically an abnormal silhouette on chest radiograph is reported. CT will detect more injuries than a conventional chest radiograph but management rarely changes. For example, children like adults with an occult pneumothorax detected by CT but not seen on plain films do not require treatment but are simply observed.104 Fenton has demonstrated that although children are frequently imaged with CT few will require a surgical intervention.105 In a cohort of injured children undergoing evaluation for blunt trauma no child imaged on the basis of mechanism of injury alone had a significant or life threatening injury. These children averaged 1.7 CT studies with a radiation exposure nearly three times the accepted annual limit for environmental sources. In contrast, in children with abnormal GCS (<15), abnormal vital signs or physical examination nearly one fourth of studies were positive for significant or life threatening injury.101 Children should not be imaged on the basis of mechanism of injury but rather imaging should be directed by findings of the primary and secondary survey.
In an effort to reduce CT utilization clinical decision rules to identify children at low risk for clinically important injuries requiring intervention have been developed. The use of these decision instruments can effectively reduce unnecessary imaging of minor head injury, the cervical spine, and the abdomen. In the absence of GCS less than 14, altered mental status, LOC greater than 5 seconds, vomiting, severe mechanism of injury, or skull fracture children can be observed safely rather than imaged by CT. When studied the prediction rule demonstrated a negative predictive value of 100% in children over the age of 2 years.106 In the National Emergency X-Radiography Utilization Study decision instrument was examined in a prospective, multicenter study. The investigators found that the decision rule correctly identified all pediatric patients with cervical spine injury and correctly designated 20% of patients as low risk for whom imaging could have been eliminated.107 The Pediatric Emergency Care Applied Research Network examined a clinical decision rule comprised of seven history and physical examination factors (abdominal wall bruising, GCS <14, abdominal tenderness, thoracic trauma, complaints of abdominal pain, decreased breath sound, or vomiting) that successfully identified children at low risk of clinically important abdominal injuries; 5.4% of children with a seatbelt sign or GCS less than 14 underwent an abdominal intervention (Fig. 43-6). In contrast, if none of the criteria were present the risk of an abdominal intervention was 0.1%.108 While the decision instrument can reduce imaging, the specificity of 42.5% demonstrates that over half of the studies still remain negative of clinically significant injury. These data support the opinion that pan-scanning or nonselective imaging of children is unwarranted.
FIGURE 43-6
Example of a validated decision instrument to reduce unnecessary imaging in injured children by identifying at risk children who likely benefit from imaging.108 (Reproduced with permission from Holmes JF, Lillis K, Monroe D, et al. Identifying children at very low risk of clinically important blunt abdominal injuries. Ann Emerg Med. 2013;62(2):107-116. Copyright © Elsevier.)

The primary and secondary assessments in children demonstrate subtle differences from those in the adult but the principles remain largely the same. Seriously injured children typically suffer multisystem injury and if shock is present the risk of death is increased. Conversely, the majority of children suffer single system injury, with a low frequency of needing a craniotomy, thoracotomy, or laparotomy. Careful examination, selective laboratory studies, and the use of decision instruments can lead to directed imaging with few missed injuries.
Head injury is common in children. Traumatic brain injury (TBI) is a leading type of childhood injury according to the Center for Disease Control. In the United States alone children aged 0–14 years make almost half a million emergency department visits for TBI annually.109 TBI resulting from unintentional trauma constitutes the primary cause of death among children and young adults. Fortunately, most brain injuries are mild110 but even this level of injury is associated with ongoing problems.111 TBI is a significant financial burden on the US economy. Charges for TBI related hospital visits are in excess of 1 billion dollars per year in the United States.112 Neuropsychological sequelae associated with pediatric TBI can influence key developmental processes, such as learning, emotional awareness, and social functioning.113 Injury costs for children are often greater than those for adults, because they include expenditures for acute treatment, long-term rehabilitation, and loss of productivity for the parent or guardian.
Children less than the age of 1 year are at increased risk of abusive head injury. In toddlers (age 1–4 years) head injury most often occurs due to fall. By adolescence head injury as a result of motor vehicle crash is most frequent. Children suffering unintentional serious head injury often have concomitant multisystem injury due to significant blunt force mechanism. As a result nearly 80% of all trauma deaths in children have associated traumatic brain injury. Consequently, TBI is the most common cause of disability and death in childhood. In children with severe TBI mortality exceeds 30%.114 As the severity of injury increases the risk of death, vegetative state, or decreased functional status rises. Interestingly the initial severity of injury does not correlate with the risk of late disability with some type of disability observed with the same frequency in mild (47%), moderate (45%), or severe injury (48%).111
At birth the head is one-fourth the total body length, whereas in the adult it is one-seventh (Fig. 43-7). The head is proportionally large and the legs proportionally short during childhood. As a result the center of gravity in an infant and toddler is more cephalad.115 The skull of the infant is thin and soft, and the closure of sutures is not completed until age 3 years. This heavier head mass coupled with a higher center of gravity and weak neck, may in part account for the propensity for children to strike the head in a fall and the higher frequency of head injury. The volume of cerebrospinal fluid is smaller than that of the adult, brain water content is increased, and brain myelination is incomplete until age 2 years. As a result the brain is soft and prone to injury. Due to the anatomy and biomechanical factors observed in young children, acceleration and deceleration injury yields a greater amount of force applied to the brain and greater frequency of shear injury. Children appear to experience age-dependent pathology following pediatric TBI. In infants and young children, subdural hematomas and diffuse injury are more common than focal injuries, particularly in those suffering abusive head trauma.116
TBI is a constellation of the primary brain injury that occurs at the time of the inciting event and the subsequent secondary brain injury that arises from derangements in cerebral blood flow, ischemia, and the inflammatory response to injury. Primary brain injury results from the immediate force of trauma. These forces can produce focal injury to the brain. Shearing of blood vessels can produce epidural hematoma, subdural hematoma, and/or subarachnoid hemorrhage. Parenchymal injury is worsened by compression from the mass effect of blood. The end result is parenchymal injury exacerbated by increased swelling of the injured brain that is trapped in a confined space. The intracranial vault is incompressible and holds a fixed volume of brain, cerebrospinal fluid, and blood. Any increase in volume of one of the cranial constituents must be compensated by a decrease in volume of another. This concept, the Monro-Kellie doctrine, serves as the basis for the management of brain injury.117
Initial compensation for the increase in intracranial volume is displacement of CSF to the spinal canal and displacement of venous blood to the jugular veins to reduce intracranial pressure (ICP). Once these compensatory mechanisms are exhausted, even small increases in cerebral edema and intracranial volume lead to significant increases in ICP further compromising cerebral perfusion. Cerebral ischemia and edema follow and if uninterrupted result in brain herniation and death. Care of the primary brain injury may require neurosurgical intervention but reduction in secondary brain injury can be initiated as early as the prehospital phase of care by nonneurosurgeons. It must be remembered that secondary brain injury develops in the initial minutes following primary brain injury. A myriad of physiologic and metabolic alterations ensue. Hypotension and hypoxia have consistently been demonstrated to exacerbate secondary brain injury.34 Both can be addressed by the non-neurosurgeon by the restoration of respiration and circulation. The acute management of traumatic brain injury is directed to prevent or ameliorate events that promote secondary brain injury. To date prevention of delayed secondary brain injury as a result of neuronal cell degeneration, axonal injury (TAI) with neuronal cell death remains investigational.118 Contemporary therapies focus on cerebrovascular dysregulation, swelling, metabolism, and ischemia.
The Glasgow Coma Scale (GCS) is a scoring tool that permits assessment and stratification of the severity of brain injury. GCS correlates with the risk of disability or death.114 Calculation of the GCS and physical examination permits stratification of injured children with mild, moderate, or severe TBI. Validated decision instruments direct the need to obtain brain imaging.106 In general, any child with an abnormal GCS should undergo brain imaging. Additional history (LOC >5 seconds, amnesia), symptoms (vomiting, persistent headache), or physical findings (raccoon eyes, battle sign) may prompt brain imaging. The purpose of brain imaging is to exclude or confirm a brain injury, identify extra-axial blood collections, and the degree of brain compression. Prompt neurosurgical intervention may be needed for evacuation of extra-axial blood with significant mass effect, decompressive craniectomy for impending herniation or control of ICP, and placement of an intraventricular drain or ICP monitor. Delays in craniotomy or placement of ICP monitoring are associated with worse outcome.119 Goals of ICP monitoring are to adjust therapies to maintain the ICP less than 20 and maintain a minimum CPP greater than 40 mm Hg.28
Not all children with minor TBI require imaging. For children with minor TBI a period of observation with scheduled neurologic assessment is recommended. Children with intracranial injuries may initially present with only headache and vomiting due to increased intracranial pressure and thus, the recommendation to consider imaging or a period of observation. It must be remembered that even in the setting of minor TBI there is significant risk of ongoing cognitive and behavioral issues. Decision instruments for selective imaging of children can be used to reserve imaging only for those with abnormal GCS, symptoms of increased intracranial pressure (vomiting, headache), or other signs of head injury (skull fracture, battle sign, and raccoon eyes).106 Plain x-rays may demonstrate a skull fracture but provide no information regarding the underlying brain injury and cannot substitute for CT. The CT is reviewed for mass lesions, early signs of brain swelling, or evidence of diffuse brain injury. Conversely, a normal CT scan and brief period of observation may permit discharge with continued observation at home obviating the need for hospitalization.120
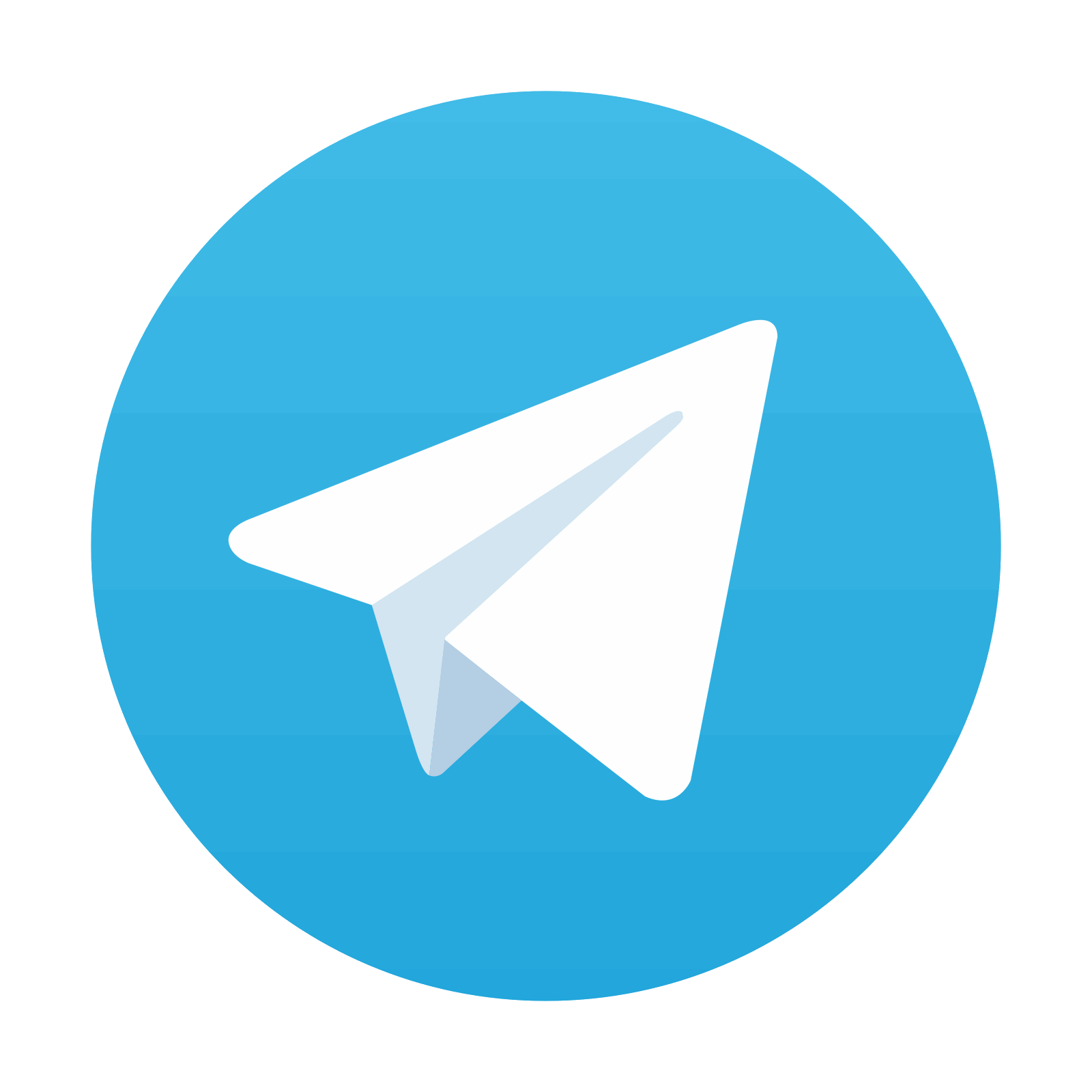
Stay updated, free articles. Join our Telegram channel

Full access? Get Clinical Tree
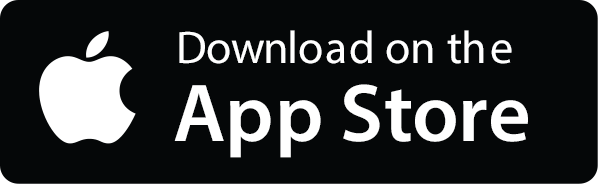
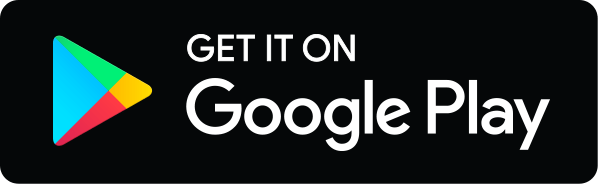