Fig. 17.1
Chromoendoscopy of colorectal lesions. (a) A polypoid lesion can be identified in the ascending colon of a 64-year-old patient with 34 years of ulcerative colitis. (b) Chromoendoscopy with methylene blue (0.1 %) clarifies the mucosal pattern (pit-pattern IIIL, arrow), which predicts a tubular adenoma. Endoscopic resection was performed and final histology confirmed adenoma with low-grade intraepithelial neoplasia. (c) A sessile lesion can also be identified. Here wide cryptapertures can be seen (pit-pattern II) using magnification and (d) chromoendoscopy. Hyperplastic changes (non-neoplastic) could be confirmed histologically
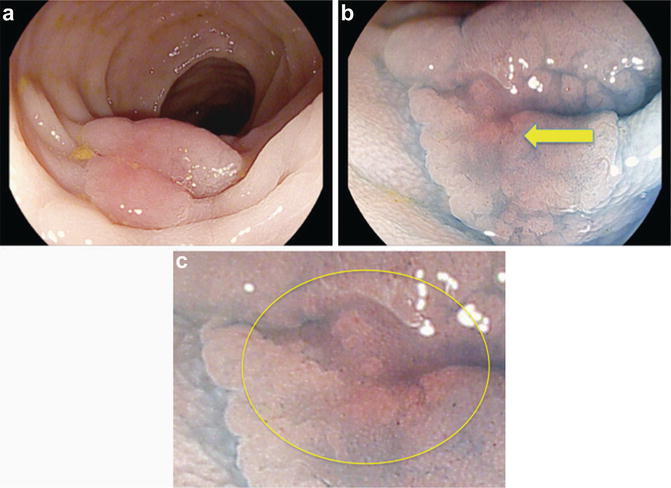
Fig. 17.2
Colitis-associated dysplasia. (a) A flat lesion is visible using white light HD colonoscopy. (b) Chromoendoscopy with methylene blue (0.1 %) is used to clarify the borders and surface architecture. Irregular pattern with shallow depression (Type IIc, pit-pattern V) can be identified (arrow) and (c) magnified view. Endoscopic resection revealed colitis-associated early cancer (shallow infiltration of the submucosal layer)
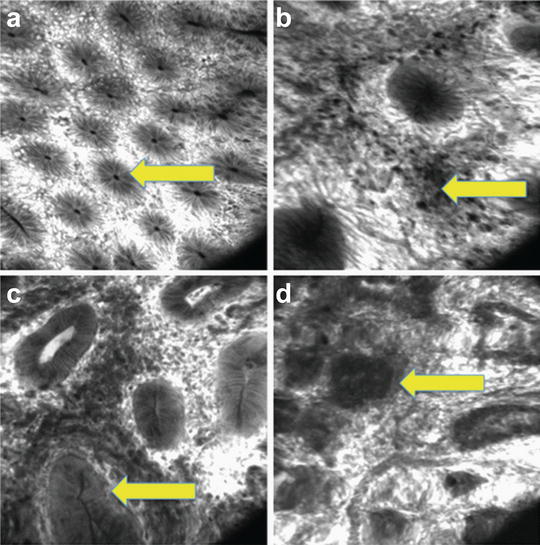
Fig. 17.3
Endomicroscopy in IBD. (a) Normal colonic mucosa with regular crypt architecture (arrow) can be seen. (b) Inflammatory cells can be identified within the lamina propria as a sign of chronic inflammatory changes. (c) Inflammatory changes and dysplastic crypts can be seen (arrow). The basement membrane is intact. Targeted biopsies confirmed the presence of low-grade intraepithelial neoplasia (colitis associated dysplasia). (d) Colitis associated cancer is present. Distorted glands with infiltration of malignant cells into the lamina propria (arrow) can be identified
Endomicroscopy has also shown to be of benefit for dysplasia detection and differentiation of lesions to optimize their management (differentiation between colitis-associated neoplasia, sporadic neoplasia and non-neoplastic lesions) and to reduce the number of unnecessary biopsies [4]. Confocal laser endomicroscopy (CLE) has for the first time revealed in vivo tissue microscopy to gastroenterologists [4]. Using this technology, changes in vessel, connective tissue and cellular-subcellular structures can be defined during ongoing colonoscopy at subcellular resolution [5, 6].
Confocal endomicroscopy has further decreased the need for random biopsies. Endomicroscopy is often combined with chromoendoscopy. Vital staining is used to unmask lesions and targeted endomicroscopy is performed to clarify the need for standard biopsies. Endomicroscopy has a very high negative predicting value. Thus, endomicroscopically normal-looking mucosa does not usually require additional standard biopsies. Neoplastic changes and regenerative tissue can readily be identified using this method. However, significant knowledge about the micro-architecture of the mucosa is necessary to achieve high diagnostic yields [6, 7].
Technical Principles of Confocal Laser Endomicroscopy
The CLE-technique introduced in 2004 has been developed for cellular and subcellular imaging of the mucosal layer [5].
In confocal microscopy a low power laser is focused to a single point in a microscopic field of view and the same lens is used as both condenser and objective folding optical path, so the point of illumination coincides with the point of detection within the specimen [6]. Light emanating from that point is focused through a pinhole to a detector and light emanating from outside the illuminated spot is rejected from detection.
As illumination and detection system are at the same focal plane, they are termed “confocal” [6]. All detected signals from the illuminated spot are captured and the created image is an optical section representing one focal plane within the examined specimen. The image of a scanned region can be constructed and digitized by measuring of light returning to the detector from successive points and every point is typically scanned in a raster pattern [6].
Currently, two CLE-based systems are used in clinical practice and research [6, 7] (see Table 17.1):
Table 17.1
Technical aspects of endomicroscopic systems
Endoscope based | Probe based | |
---|---|---|
Outer diameter (mm) | 12.8 | 1.0; 2.7; 2.6a |
Length (cm) | 120; 180 | 400; 300a |
Field of view (μ[mu]m) | 475 × 475 | 320; 240; 600 μ(mu)m2a |
Resolution | 0.7 | 3.5; 1.0a |
Magnification | ×1000 | ×1000 |
Imaging plane depth (μ[mu]m) | 0–250 (dynamic) | 40–70; 55–65; 70–130 (fixed)a |
1.
In confocal laser endomicroscopy (CLE), a miniaturized confocal scanner has been integrated into the distal tip of a flexible endoscope (Pentax Endomicroscopy System, Japan). A blue laser light source delivers an excitation wavelength of 488 nm, and light emission is detected at > 505 nm [8]. Successive points within the tissue are scanned in a raster pattern to construct serial en face optical sections of 475 × 475 μ(mu)m at user-controlled variable imaging depth. Lateral resolution is 0.7 μ(mu)m and optical slice thickness 7 μ(mu)m (axial resolution). Images on the screen approximate a 1,000-fold magnification of the tissue in vivo [8].
2.
In contrast, the probe-based system (pCLE—Cellvizio Endomicroscopy System, Mauna Kea Technologies, Paris, France) consists of a 1.5-mm flexible mini-probe with lateral resolution depending on the mini-probe between 3.5 μ(mu)m–1 μ(mu)m and axial resolution 5.0 μ(mu)m and is compatible with the working channel of any standard endoscope [7, 8]. These probes can be fitted through the working channel of most endoscopes for clinical use. Image acquisition is faster with this probe (12 frames/s) at the expense of resolution being limited by the number of the fibers (30,000 single fibers = pixels).
Compared with probe-based CLE, the endoscopic CLE has slightly higher lateral resolution (approximately 0.7 versus 1.0 μ[mu]m), a larger field of view (approximately 475 versus 240 μ[mu]m) and variable imaging plane depth (approximately 0–250 versus 0–65 μ[mu]m). However, the mini-probe is currently the only commercially available system and can be used in conjunction with any standard endoscope. It is passed through the working channel and endomicroscopic images at video-frame rates are obtained, which allows a dynamic examination of the vessels and micro-architecture (12 versus 0.8–1.6 frames per second).
Endomicroscopy requires contrast agents. The most commonly used dyes are fluoresceine (intravenous application), acriflavine (local application) and cresyl violet (local application) [8–11].
The great potential of endomicroscopy is not only in vivo histology. Endomicroscopy also enables us to display and observe physiologic and pathophysiologic changes during ongoing endoscopy. Furthermore molecular imaging becomes possible [12].
In inflammatory bowel diseases, CLE has been able to demonstrate intramucosal bacteria within the lamina propria [13]. These intramucosal bacteria are much more common in IBD patients compared to normal controls. These new visible details might refine our understanding of IBD, because increased cell shedding is linked with increased intramucosal bacteria as well as with a higher risk to develop a flare within 12 months [14].
Most recently, endomicroscopy has been used for molecular imaging. As such labelled antibodies (adalimumab) have been applied topically onto the affected (inflamed) mucosa in patients with Crohn’s disease. The amount of membranous TNF-alpha receptors within the mucosa could be quantified and the response to biological therapy could be predicted with high accuracy based on the fluorescence pattern of the receptors [15].
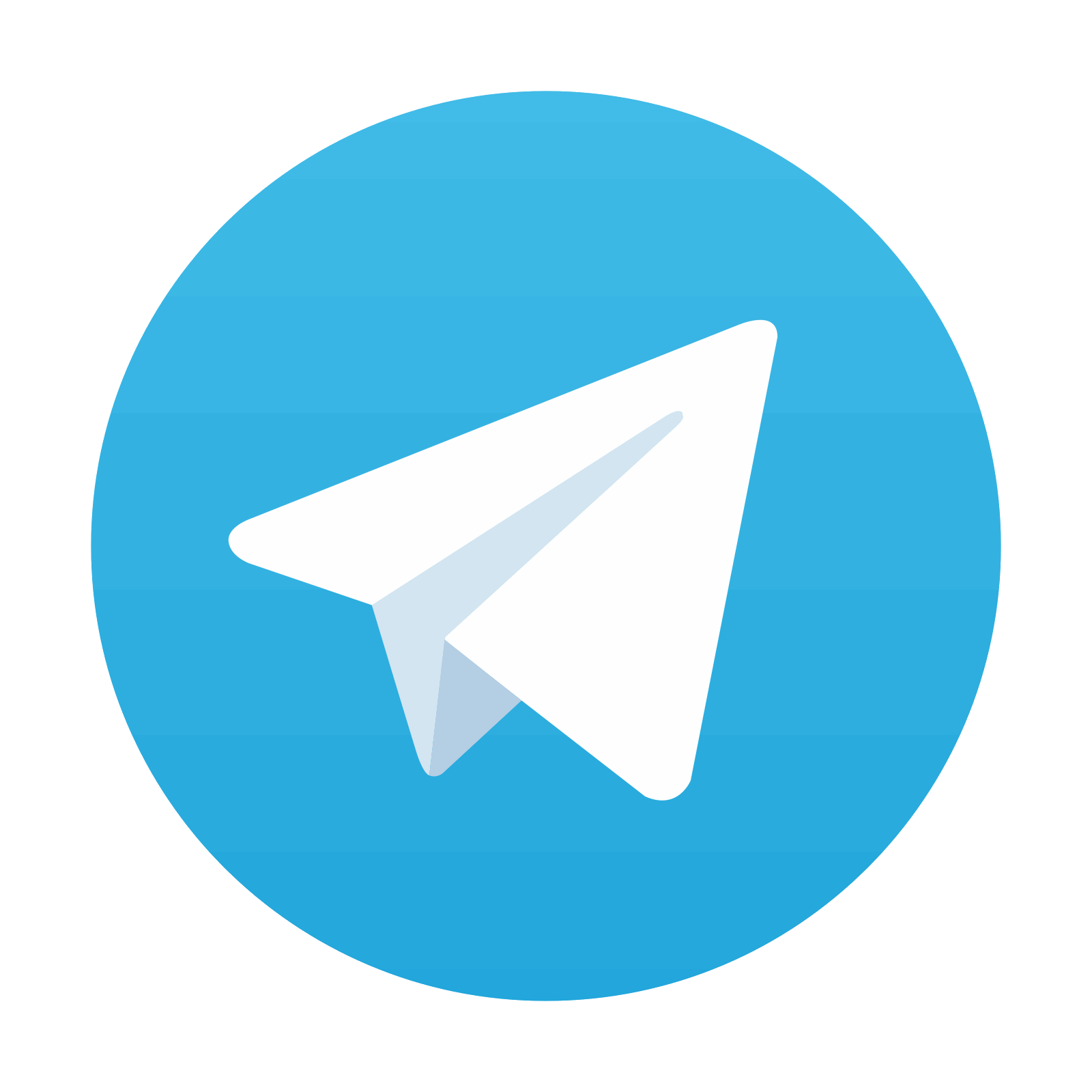
Stay updated, free articles. Join our Telegram channel

Full access? Get Clinical Tree
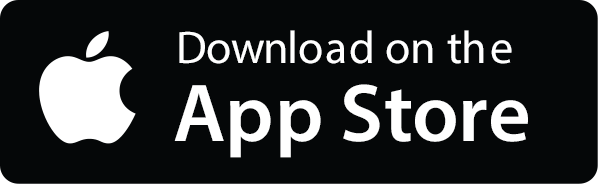
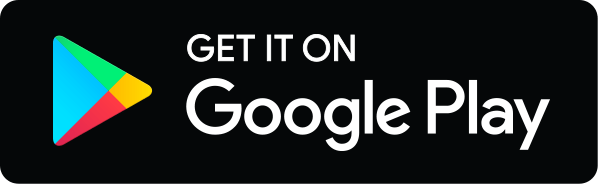