In this article, the authors describe the evolution of urologic robotic systems and the current state-of-the-art features and existing limitations of the da Vinci S HD System (Intuitive Surgical, Inc.). They then review promising innovations in scaling down the footprint of robotic platforms, the early experience with mobile miniaturized in vivo robots, advances in endoscopic navigation systems using augmented reality technologies and tracking devices, the emergence of technologies for robotic natural orifice transluminal endoscopic surgery and single-port surgery, advances in flexible robotics and haptics, the development of new virtual reality simulator training platforms compatible with the existing da Vinci system, and recent experiences with remote robotic surgery and telestration.
This article is not certified for AMA PRA Category 1 Credit ™ because product brand names are included in the educational content. The Accreditation Council for Continuing Medical Education requires the use of generic names and or drug/product classes as the required nomenclature for therapeutic options in continuing medical education.
For more information, please go to www.accme.org and review the Standards of Commercial Support.
Despite its relative infancy, laparoscopic surgery has radically transformed the landscape of operative urology. The advent of surgical robotics at the turn of the new millennium heralded a quantum leap forward for minimally invasive urologists, who first used it successfully for performing robotic-assisted radical prostatectomy. Since then, there has been an unprecedented explosion in the use of robotics in other aspects of oncologic and reconstructive urologic procedures, with more than 55,000 radical prostatectomies performed with da Vinci ® (Intuitive Surgical, Inc., Sunnyvale, California) robotic assistance in the United States in 2007 and more than 70,000 performed worldwide in 2008 (unpublished data, Intuitive Surgical, Inc., 2008). The increasing popularity of robotic-assisted laparoscopic surgery seems to be mirrored in Europe and other parts of the world, and in the arenas of cardiothoracic, gynecologic, and general surgery.
Propelling this zeitgeist for surgical robotics have been exciting advances in robotic technologies and their potential applications in urologic surgery. This article therefore serves as a timely review of these promising innovations to date and discusses likely future directions for our craft as the practice of surgery becomes increasingly robotic-assisted and computer-aided.
Evolution of urologic robotic systems and current state of the art
Urologic robotic systems used in recent years have essentially comprised a computer with real-time imaging capability linked to various effector units for execution of specific tasks. Off-line (ie, fixed path) robots are automated systems that execute precise movements within specified confines based on preprogrammed imaging studies obtained before surgery, operating independently without requiring active input from the surgeon. These include (1) robots for prostate access, such as the ProBot (prototype by a team from Imperial College, London–not commercially available and not manufactured), a robotic resection device with 7 df , and various robotic prostate biopsy systems, and (2) renal access systems, such as the PAKY-RCM and Acubot robots (prototypes developed by Stoianovici et al at URobotics Laboratory, Johns Hopkins Medical Institute, Baltimore, Maryland), for precise percutaneous access to the kidney.
Conversely, on-line robotic systems are designed to replicate the surgeon’s movements in real time in the operative field with improved tremor-free precision and scale adjustment when applicable. These surgeon-directed robots may be broadly divided into endoscope manipulators and master-slave systems. Endoscopic manipulators, such as the Automated Endoscopic System for Optimal Positioning (AESOP; Intuitive Surgical Inc.) and Naviot (Hitachi Hybrid Network Co., Ltd., Yokohama, Japan) systems, have the benefits of being less expensive, smaller, and easier to set up with stable adjustable positioning, but their usefulness remains limited in complex operations. Master-slave systems, such as the now obsolete Zeus (originally manufactured by Computer Motion, Inc., Goleta, California) and the da Vinci ® system, comprise a computerized surgical console connected to an endoscopic manipulator with two or three robotic arms for instrument manipulation. Precise digital control of surgical instruments through the console eliminates movement tremors and allows motion scaling, wherein the surgeon’s movements may be amplified or dampened.
The most commercially successful robotic system to date has been the da Vinci ® system, with more than 1000 systems currently installed in hospitals worldwide. From its inception, the benefits of the da Vinci ® system over conventional laparoscopy were readily apparent: superior ergonomics; optical magnification of the operative field within direct control of the console surgeon; and enhanced dexterity, precision, and control of operative movements. Comprising a patient-side cart with three or four robotic manipulator arms connected to a master console, the da Vinci’s binocular images obtained by means of the laparoscopic camera lens (0° or 30°) are integrated by the computer to provide a composite three-dimensional (3D) image when viewed by means of the immersive stereo viewer at the console. The patented robotic instruments also have additional articulating joints (EndoWrist; Intuitive Surgical, Inc.) that permit 7 df of movement, empowering the minimally invasive surgeon to perform intracorporeal suturing and dissection intuitively and effortlessly.
Its current state-of-the-art version, the da Vinci ® S HD Surgical System (Intuitive Surgical, Inc.), integrates 3D high-definition vision capability with the existing robotic platform, providing twice the effective viewing resolution with improved clarity and detail of tissue planes ( Fig. 1 ). Its digital zoom function reduces interference between the endoscope and instruments, and the integrated touch-screen monitor permits telestration for improved proctoring and team communication. In addition, the TilePro ® (Intuitive Surgical, Inc.) multi-image stereo viewer enables simultaneous display of multiple video inputs in the surgeon console, integrating display of the patient’s ultrasound, CT, and MRI images. Extended-reach instruments are also now available for multiquadrant access, with a 50% increase in pitch and yaw range of motion and four times the working volume. Fewer cable connections between components has also helped to shorten setup time, and a high-speed fiberoptic connection in the surgical platform offers the potential for remote telementoring.
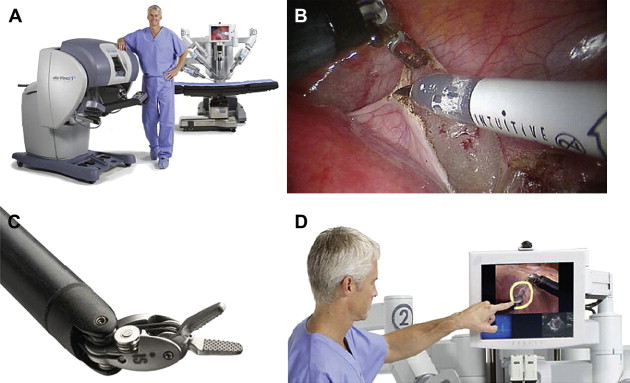
Despite these technical innovations, there still exist some limitations. First, the da Vinci ® S HD Surgical System is unable to provide haptic feedback for the console surgeon, who must necessarily base his or her intraoperative decisions on visual cues encountered during surgical dissection. Second, the large size of the robot presents some challenges to the operating staff when docking and repositioning the robot during complex procedures. Third, there remains a lack of simulator technologies available to surgeons and residents desiring familiarity with the robotic console, with their options currently limited to attending training courses that are mostly didactic in nature. Finally, the high cost of this technology may limit or slow the adoption of robotic-assisted laparoscopic surgery on a larger scale internationally.
Against this background, we now review some exciting technological advances that promise to redress some of these technical issues and bring the practice of robotic surgery into the mainstream.
Miniaturization of robotic platform
The current design of the da Vinci’s robotic cart is based on the traditional laparoscopic platform, wherein straight rigid instruments are used intracorporeally through small incisions by the surgeon maneuvering the instrument handles outside the patient’s body. As a result, the current da Vinci ® system occupies significant overhead over the sterile field, presenting spatial challenges for the patient-side assistants to overcome to operate dexterously alongside the robotic arms and cart.
In contrast, the Laprotek system (EndoVia, Inc., Norwood, Massachusetts, subsequently taken over by Hansen Medical, Inc., Mountain View, California) comprises slave instrument “motor packs” that are mechanically mounted on the existing bed rails of the operating table. The movements from these motors are then transmitted to the surgical instruments by means of stainless-steel cables. Whereas the da Vinci ® system directs its straight instruments by moving their back handles through a 3D cone, the Laprotek system uses a curved guide tube to position its instruments intracorporeally. Its design occupies significantly less space in the sterile field, reducing collisions between the robotic instruments and camera ( Fig. 2 ). Although not commercially available, it has been projected to cost significantly less than the da Vinci ® system.
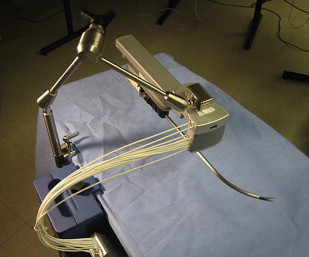
Working on the premise that the motion outside the patient should be confined to a line rather than a cone (da Vinci ® system) or a plane (Laprotek system), Dachs and Peine proposed a new design wherein the robotic instruments would have two movable joints within the body that would permit 6 df of movement without requiring corresponding external pivoting motions ( Fig. 3 ). Because the instruments would not be constrained to pivot about the port side, they could be easily mounted on a streamlined mechanical arm that supports its linear track to deliver comparable intracorporeal dexterity and precision. The advantages of their proposed design would include minimization of machinery in the exterior working envelope, giving surgical assistants more room to operate, and elimination of instrument collisions attributable to suboptimal port placement ( Fig. 4 ).
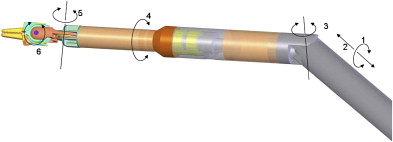
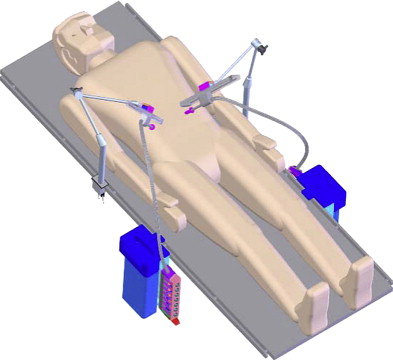
Miniaturization of robotic platform
The current design of the da Vinci’s robotic cart is based on the traditional laparoscopic platform, wherein straight rigid instruments are used intracorporeally through small incisions by the surgeon maneuvering the instrument handles outside the patient’s body. As a result, the current da Vinci ® system occupies significant overhead over the sterile field, presenting spatial challenges for the patient-side assistants to overcome to operate dexterously alongside the robotic arms and cart.
In contrast, the Laprotek system (EndoVia, Inc., Norwood, Massachusetts, subsequently taken over by Hansen Medical, Inc., Mountain View, California) comprises slave instrument “motor packs” that are mechanically mounted on the existing bed rails of the operating table. The movements from these motors are then transmitted to the surgical instruments by means of stainless-steel cables. Whereas the da Vinci ® system directs its straight instruments by moving their back handles through a 3D cone, the Laprotek system uses a curved guide tube to position its instruments intracorporeally. Its design occupies significantly less space in the sterile field, reducing collisions between the robotic instruments and camera ( Fig. 2 ). Although not commercially available, it has been projected to cost significantly less than the da Vinci ® system.
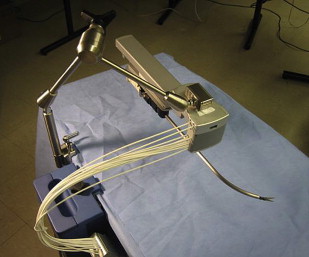
Working on the premise that the motion outside the patient should be confined to a line rather than a cone (da Vinci ® system) or a plane (Laprotek system), Dachs and Peine proposed a new design wherein the robotic instruments would have two movable joints within the body that would permit 6 df of movement without requiring corresponding external pivoting motions ( Fig. 3 ). Because the instruments would not be constrained to pivot about the port side, they could be easily mounted on a streamlined mechanical arm that supports its linear track to deliver comparable intracorporeal dexterity and precision. The advantages of their proposed design would include minimization of machinery in the exterior working envelope, giving surgical assistants more room to operate, and elimination of instrument collisions attributable to suboptimal port placement ( Fig. 4 ).
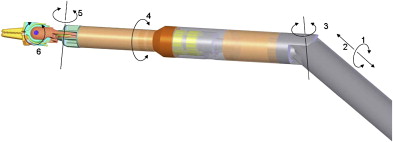
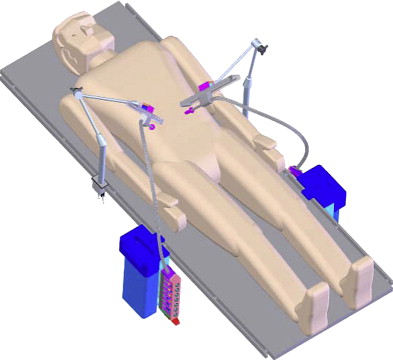
Mobile miniaturized in vivo robots
Despite improvements in high-definition real-time clarity of tissue architecture and a wider field of view with the da Vinci ® S HD Surgical System, the surgeon’s visual and operative fields remain constrained by camera and instrument placement dictated by the entry incisions. To overcome these limitations, Rentschler and Oleynikov’s group from the University of Nebraska has explored the use of in vivo microrobots. They first reported the use of miniature in vivo adjustable-focus camera wheeled robots to augment visual feedback to surgeons during laparoscopic cholecystectomy in a canine model. Mounted on two helical wheels driven by direct current motors, these microrobots had sufficient traction to move over slick deformable abdominal viscera without causing injury ( Fig. 5 ). The same investigators subsequently designed a fixed-base pan-and-tilt camera robot that permitted forward tilting at an angle of 45°. Comprising tripod legs that were spring-loadable and could be abducted during insertion through the laparoscopic port, this microrobot could then be guided into a desired intra-abdominal position with laparoscopic instruments, offering surgeons alternate views of the organs being operated on to that obtained by means of the laparoscopic camera. Joseph and colleagues recently reported their collaborative experience with these prototypes in performing laparoscopic prostatectomy and laparoscopic nephrectomy in a canine model. Rentschler and colleagues also demonstrated their use in guiding a surgically naive crew member to perform the steps of a laparoscopic appendectomy in a harsh environment by means of remote telementoring. Albeit successful, technical drawbacks encountered during these operations included the need for a separate controller to maneuver the robot into position; significant hindrance attributable to the tethered design for the robot’s continuous power; and lack of a self-cleaning mechanism for the camera lens positioned between the helical wheels, which resulted in obscured images from direct contact with intra-abdominal organs and fluids.
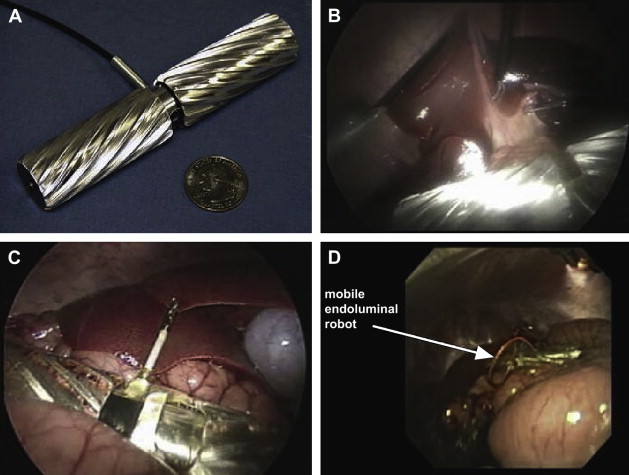
The feasibility of using multiple miniature in vivo robots for bettering spatial orientation while facilitating tasks was recently reported by Lehman and colleagues. They used three robots—a peritoneum-mounted camera robot, a lighting robot, and a retraction robot—together with a conventional upper gastrointestinal endoscope to augment the scope of natural orifice transluminal endoscopic surgery (NOTES) procedures in a porcine model.
Although still in their early stages, these and other feasibility studies have demonstrated significant potential for eventual development of wireless robotic sensors to provide composite all-round real-time images and robotic manipulators that would obviate the need for multiple incision sites for instrument access.
Advances in endoscopic navigation systems
In performing laparoscopic urologic procedures with da Vinci ® assistance, surgeons rely on visual cues instead of tactile feedback to achieve comparable outcomes to those reported for traditional open surgery. Suture tension, for example, has been dictated by the degree of deformation on the respective tissue. Imaging technologies based on virtual and augmented reality have evolved to provide real-time navigational guidance for the surgeon to perform image-guided surgery. Augmented reality may be defined as the integration of computer-generated images (from reconstructed preoperative MRI, CT, or ultrasound images) to live video or other real-time images, such as ultrasound, allowing visualization of visceral anatomy and surrounding structures.
The initial experience of such image-guided surgery in neurosurgery, maxillofacial surgery, and orthopedics was based on integrating images over fixed bony landmarks. Attempts to extrapolate its use in abdominal surgery have highlighted the challenges of imaging viscera that constantly shifts and undergoes deformation: (1) to find constant intra-abdominal reference points and (2) to merge preoperative images over constantly shifting soft tissue anatomy attributable to breathing, heartbeat, patient movement, and surgical instrumentation.
Ukimura and Gill recently described their experience of using a color-coded zonal navigation system to perform laparoscopic partial nephrectomy. Such a system affords the surgeon a 3D visual surgical roadmap based on preoperative CT images to identify safe resection margins during surgery by giving different colors to the tumor and its adjacent tissues. Ukimura and his colleagues further reported the use of intraoperative transrectal 3D ultrasonography to guide the course of laparoscopic nerve-sparing radical prostatectomy.
Attempts at incorporating conventional fluoroscopy during robotic procedures have been hindered by spatial difficulties of reconciling the sizable overhead footprint of the fluoroscopic and da Vinci ® systems over the sterile field. In addition, fluoroscopy only provides cursory images of calcified or radiopaque structures, with limited soft tissue information; the need for operating staff to wear heavy protective gowns to protect them from collateral radiation exposure is an unattractive prospect during complex laparoscopic procedures; and the anterior-posterior images afforded by fluoroscopy leave many “blind spots” during surgical dissection.
The TilePro ® system uses a “picture in picture” viewer in the da Vinci ® surgeon’s console to toggle among images from various sources. Bhayani and Snow recently described their experience with this system to assimilate images from preoperative CT or MRI scans, real-time ultrasonographic images, and 3D images viewed through the da Vinci ® surgeon console during robotic-assisted laparoscopic partial and radical nephrectomy. In 2 of 17 partial nephrectomies, superselective arterial control was possible using Doppler information fed into the surgical console during tumor resection. Although providing invaluable information during surgery, the images were static and not superimposable to the mobile operative field. Although the TilePro ® system has increased the availability of anatomic images available to the console surgeon during surgery, the ultimate direction would certainly be to integrate these images in real time onto the operative field as seen through the immersive viewer.
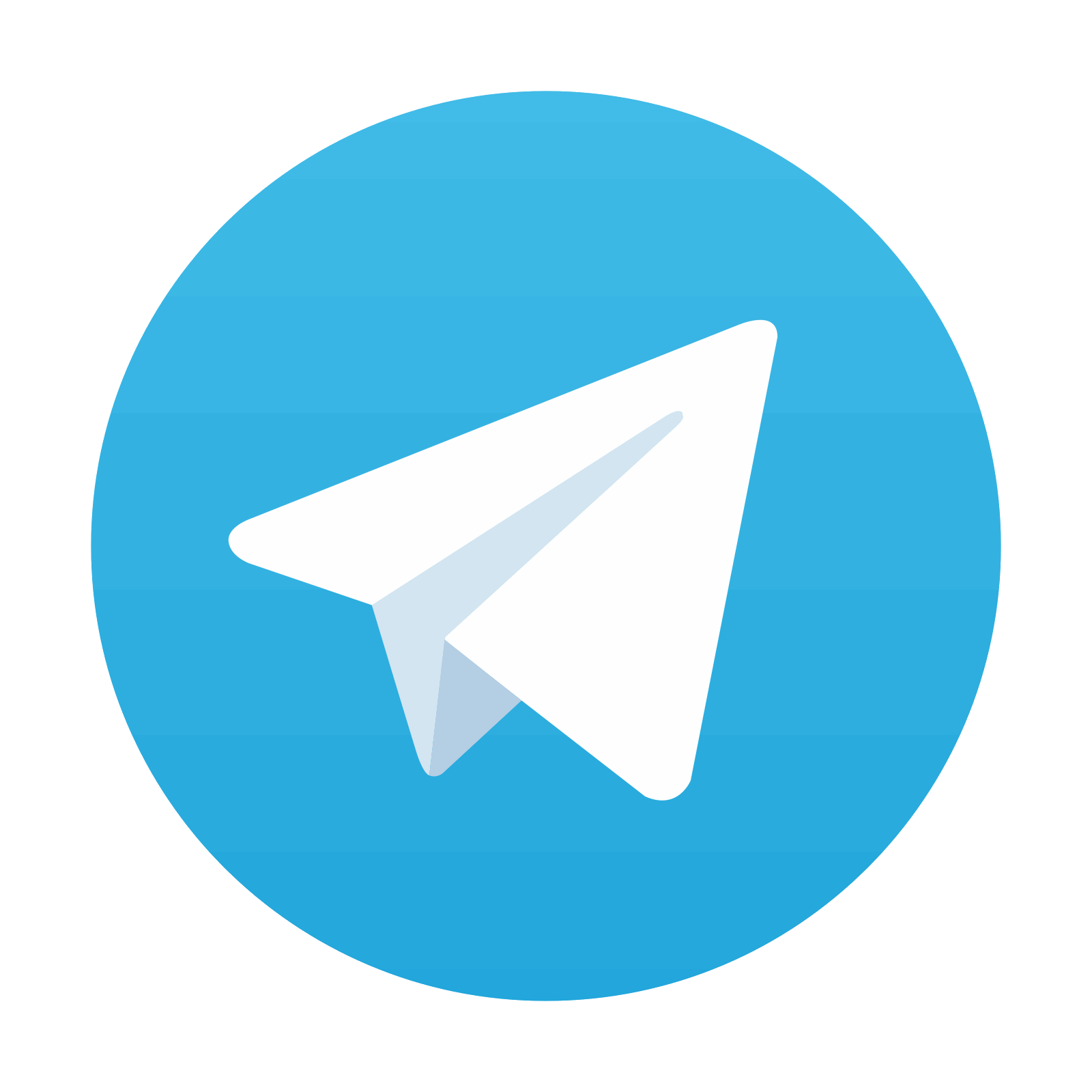
Stay updated, free articles. Join our Telegram channel

Full access? Get Clinical Tree
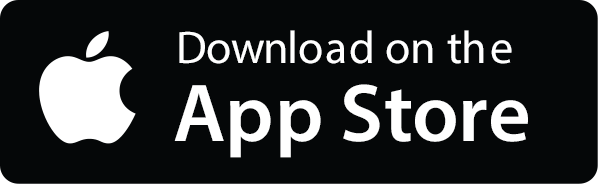
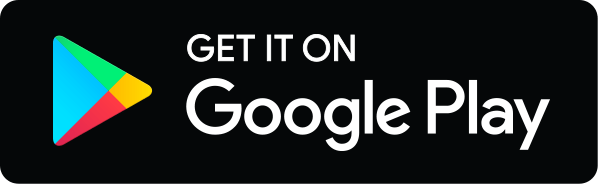