Chapter 2 STRUCTURAL BASIS OF VOIDING DYSFUNCTION
Functional behavior of the urinary bladder has been investigated for more than a century, but several aspects of the mechanism of voiding and the way it is altered in vesical dysfunction remain unresolved. This can largely be attributed to the complexity of structural organization of the bladder and its outlet and the matching complexity of their functions.1–7
The storage (i.e., filling) and expulsion (i.e., voiding) phases of micturition involve essentially opposite functions of the bladder and urethra.8 The bladder acts as a reservoir for urine during filling and as a pump for expelling its stored urine during voiding. The urethra during bladder filling is closed, sealed, and noncompliant, acting as a sphincter to maintain continence, but it opens, dilates, and becomes compliant during voiding, acting as a conduit for the urinary stream. Efficient urine storage requires a compliant and stable detrusor together with a continent bladder outlet.5,9 Compliance of the detrusor allows distention of the bladder to capacity, and its stability ensures absence of untimely contractions that could involuntarily force some urine past the closed outlet, resulting in incontinence. Complete emptying of the full bladder9 depends on optimal contractility of the detrusor so that it can mount a strong, speedy, sustained, and unitary voiding contraction; coordinated opening of the bladder outlet; and maintenance of the opened outlet as a free conduit for an uninterrupted and strong urinary stream.
The anatomy and structure of the bladder and urethra must be optimally suited to the complex dynamic events in the micturition cycle.6 Important elements in this regard are the inherent physical and biomechanical properties of the tissue components of the vesical and urethral walls and their bearing on organ distensibility and contractility.8,10–12 Two crucial elements are the topographic and microstructural organization of the musculature of the bladder wall and urethra and the elaborate system of vesicourethral innervation, with complex central cephalospinal control and intricate peripheral pathways.1–6,13,14
Various disciplines have contributed through experimental and clinical investigation to our knowledge of bladder function and dysfunction. Gross anatomy was the natural start during the previous century, and it prevailed for many decades. It resulted in some fundamental concepts that have been expanded and refined in the current century as the result of improved methods of dissection, neuroanatomic tracing techniques, and microscopic staining procedures. After the initial era of anatomic investigation, the principal approach to studies on voiding has been the characterization of physiologic and muscular responses of the lower urinary tract, mainly the bladder. It is undeniable that definition and measurement of these responses are important for understanding the overall nature of neuromuscular function of the bladder and urethra. Nonetheless, such an approach cannot define the factors that determine function of the effector organ (i.e., smooth muscle of detrusor and urethral wall) in regard to the exact mechanism and balance of their contractility, distensibility, and stability during the filling and expulsion phases of micturition. Attempts to define these factors based purely on physiopharmacologic studies are largely inferential and have generated some misconceptions. One such misconception is the idea that the sympathetic autonomic nervous system has little or no role in vesical or urethral function.15,16 This dogma prevailed through the mid-1960s, until it was invalidated by microscopic proof of sympathetic innervation of the vesicourethral muscularis, which was subsequently confirmed by innumerable physiopharmacologic observations.1,4,5,7
Landmarks in our knowledge of muscular anatomy of the lower urinary tract3,6,17,18 include continuation of the muscularis of the terminal ureters as the vesical “trigone” and beyond into the dorsal wall of the urethra; the nonlayered, interwoven organization of muscle bundles of the detrusor13; identification of a vesical sheath around the terminal ureters,19 which was eventually refined as the concept of dual ureteral sheath20,21; and the concept of the rhabdosphincter as an integral striated muscle component of urethral muscularis.1,3,6,22 Milestones in our knowledge of the innervation and neural control of the bladder and urethra include1–5 definition in the spinal cord of a sacral parasympathetic and a lumbar sympathetic nucleus for subcephalic bladder control, as well as a sacral cord nucleus supplying peripheral somatomotor innervation of the volitional urinary sphincter23–26; multilevel localization of centers of bladder control in the brain, their interconnections, and their spinal neurotract projections27–30; description of the topographic organization of peripheral sympathetic and parasympathetic outflows, respectively, through the hypogastric and pelvic nerve or plexus pathways and their differences in different species23,31,32; recognition of dual sympathetic and parasympathetic innervation of the bladder and urethra and introduction of the functional concept of bladder body versus bladder base33,34; localization of the origin of intrinsic vesicourethral innervation in peripheral ganglia close to and within the organs, including the concept of sympathetic and parasympathetic effector short neurons35,36; concepts of infraspinal interaction of sympathetic and parasympathetic pathways within peripheral ganglia (through collaterals and interneurons)34–39 and the vesicourethral muscularis (through axoaxonal synapses at the effector cell level)40–42; recognition of auxiliary autonomic innervation of the rhabdosphincter in animals and humans22,43–45; and recognition of neuropeptides as a class of putative neurotransmitters or modulatory cotransmitters in peripheral vesicourethral innervation.5–7,46,47
Full knowledge of the structure of an organ is key to the understanding of how it functions. A corollary of this axiom is that alteration of the structure of an organ is reflected in alteration of its function. The axiom and its corollary should be fundamental premises in studies of the bladder in view of its unique function and intimate anatomic relationship to two other organs of different but closely integrated function—the ureter supplying it with urine and the urethra serving as the conduit for its expulsion. Tacit awareness of these premises stimulated research at the biochemical and molecular levels during the past few decades. This research has yielded important information on the biomechanics, energetics, and neuroreceptor attributes of the bladder and urethra. Not unexpectedly, such information has not fully clarified the basis of normal or abnormal smooth muscle function of either organ.
ULTRASTRUCTURE OF THE VESICOURETHRAL MUSCULARIS
The few reports on vesical ultrastructure available before the 1980s presented general, vague, or imprecise information and therefore were largely noncontributory. A notable exception was a study on the distribution of intrinsic afferent (sensory) nerves in the cat bladder, including the relative contributions of sympathetic and parasympathetic pathways.48,49 The observations reported in this study confirmed and supplemented earlier accounts of the cholinergic and adrenergic suburothelial nerves demonstrated histochemically in the cat bladder.33 The existence of nerve terminals within the urothelium is ultrastructurally indisputable in animals and humans.1 A proposal for distinguishing suburothelial sensory nerves by electron microscopic counting of axonal synaptic vesicles50 remains unfulfilled.
Studies on the detrusor and “internal sphincter”51–53 have provided detailed information about their intrinsic innervation and have shown that their muscle cells have the ultrastructural features of smooth muscle in general.54–56 Definitions of the various terms and structural parameters have been provided in other reports.51,56–58
Muscle Cells
Ultrastructurally, each of the grossly recognizable bundles of vesicourethral muscularis in various animals and in humans is composed of incompletely separated and imperfectly outlined compact groups (fascicles) of muscle cells.51,56,58 The muscle cell profile (Fig. 2-1) has a smooth contour and a polygonal to cylindrical configuration, depending on the plane of sectioning relative to its long axis. Nuclei of typical appearance are centrally located and rarely have nucleoli. Mitoses are ordinarily absent in muscle cells of the adult bladder.59
The sarcoplasm is packed with evenly distributed myofilaments of uniform orientation and alignment, with evenly dispersed dense bodies of uniform cigar-shaped appearance in cylindrical cell profiles of longitudinally sectioned cells. The myofilaments are slanted at an approximately 10-degree angle from the long cell axis and are anchored to dense bands of sarcolemma. Organelles of typical structure, mainly mitochondria and endoplasmic reticulum, are aggregated in a conical zone capping each nuclear pole (in cylindrical profiles); some mitochondria, cisternae of reticulum, and clusters of ribosomes are also scattered in sarcoplasm, particularly beneath sarcolemmal caveolae.
Individual cells within muscle fascicles are separated by spaces of uniform width (usually <200 nm). The intercellular space contains small amounts of amorphous material similar to basal lamina and a few isolated collagen fibrils that are mainly attached to sarcolemmal dense bands of related muscle cells. Contiguous muscle cells have intermediate-type junctions (e.g., attachment plaques, zonulae adherentes) of typical appearance and length and with 30- to 60-nm separation gaps. Some also have zones (with approximately 15-nm gaps) of simple apposition of sarcolemma.57,60 Contrary to one report,61 it is generally accepted that gap junctions (i.e., nexus) are absent or only sparsely encountered in the normal vesicourethral muscularis of animals and humans.51,53,60,62
Intrinsic Nerves
Ultrastructural studies have confirmed dual innervation of the normal bladder and urethra by cholinergic and adrenergic axons and provided morphologic details of the relationships between these axons and muscle cells.1–5 Nerve elements within the vesicourethral muscularis course in its interstitium as Schwann cell–ensheathed axon bundles. Varicosities of some axons become exposed along their course in these bundles to face smooth muscle cells. The main vehicle of muscular innervation is provided by individual preterminal axons that “break away” from the bundles to establish contact with the muscle cells. Many muscle cells have cholinergic or adrenergic neuroeffector junctions, some have both (diautonomic junctions), and some have neither.2,4,51 Clefts of neuroeffector junctions (i.e., spaces between axon and muscle cell) typically are 15 to 80 nm wide.
It seems certain that no part of the vesicourethral muscularis has a 1 : 1 nerve-to-muscle ratio,1,5,59 as inferred in the original histochemical study on vesical innervation.33 The basis for upholding that impression in one study on the human bladder53 is questionable.4,6,59 Synaptic contacts between cholinergic and adrenergic or probable copeptidergic axons (i.e., axoaxonal synapses) have been discovered as a unique feature of intrinsic neuroplexuses of vesicourethral muscularis in animals40,41 and humans (A Elbadawi, unpublished data).
Interstitium
Collagen and elastic fibers are the main components of interstitium in the vesicourethral muscularis. Partitions of interstitium surround and separate muscle bundles.56,58 These partitions extend into each bundle as microsepta that incompletely delineate its component fascicles. Vascular and neural elements supplying the muscularis are contained in interstitium between and within the muscle bundles.
Microstructural Basis of Vesicourethral Muscular Function
Conceptually, the vesicourethral muscularis comprises three integrated microstructural compartments: one for generating, another for modulating, and a third for coordinating its functional responses.9,63
The generator compartment consists of smooth muscle cells, which enable the vesicourethral muscularis to respond appropriately to various stimuli, particularly to generate the neurally triggered unitary detrusor contraction of voiding. Several microstructural elements play specific roles in the excitationcontraction coupling mechanism of smooth muscle.9,52,54–56 Proper composition, organization, and intracellular disposition of myofilaments bearing the contractile proteins actin and myosin ensure generation of an optimal contractile force. A sufficient complement of intact mitochondria provides the enzymes necessary for generating the necessary ATP-dependent energy. Mitochondria and an intact endoplasmic reticulum provide and store intracellular calcium ions (Ca2+), which are necessary for muscle cell contraction. An intact sarcolemma provides anchorage for myofilaments at its dense bands to transmit the contractile force to the cell surface. The function of sarcolemmal caveolae has not yet been established. The close spatial association between caveolae and cisternae of subsarcolemmal endoplasmic reticulum, a site of Ca2+ binding and storage,54,64 suggests that they are involved in the mechanism of muscle contraction. There is evidence that caveolae are sites of active ion (Na+, K+, Ca2+) transport, Na+-Ca2+ exchange, or passive Ca2+ binding, all of which are involved in the excitation-contraction coupling mechanism.65
The modulator compartment comprises intrinsic neuroplexuses and their neuroeffector junctions. Neural influence through the latter involves induction, facilitation, inhibition, or disinhibition of chemical neurotransmission to muscle cells under central cephalospinal and peripheral autonomic ganglionic control.1,5,9 Intact structure of intrinsic axons is necessary for conduction of neural impulses throughout their course within the detrusor. Structural integrity of neuroeffector junctions, including the predominant small (clear or dense-core) vesicle content of their axonal components, is necessary for chemical neurotransmission to the target muscle cells. Axoaxonal synapses modulate prejunctional, reciprocal cholinergic-adrenergic inhibition of effector neural influence in the normal mammalian vesical muscularis, including that of humans.9,40,41 From the structural standpoint, the efficacy and net effect of neural influence on this muscularis depend mainly on the distribution and relative density of the various functional types of structurally intact axons (i.e., cholinergic versus adrenergic) and the width and content of junctional clefts of preserved neuroeffector junctions. Alteration of the inherent structure or spatial relationship of axons within the bladder may hinder one normal neurally triggered neural response or another, or it may generate incongruous, uncoordinated, or spurious muscle cell responses.9
The coordinator compartment is in part muscular and in part interstitial. It is responsible for maintaining spatial topographic organization of muscle cells relative to each other within muscle fascicles and bundles of the detrusor and urethral muscularis. Moreover, it provides the basis for tridimensional distensibility of the bladder, which allows it to be filled to capacity with minimal rise in luminal pressure (i.e., compliance).11,66 Compliance is determined by the relative proportions of muscular and interstitial elements and by the delicate balance of the rigid and elastic components of interstitium—collagen and elastin, respectively.10,66–68
The main role of the coordinator compartment is to synergize and unify muscle cell responses in the entire detrusor or urethral wall. The most important functional expression of this role is the neurally triggered, coordinated, unitary detrusor contraction that initiates voiding.1,4,9 Only a fraction of muscle cells of the detrusor are innervated and are directly excitable by neural stimuli, despite its overall dense innervation.1,4,6 Voiding contraction of the detrusor, which is in essence a synergized, summated, and sustained contraction of its component fascicles and bundles, requires transmittal of contraction from the innervated to all noninnervated muscle cells (i.e., muscle cell coupling).
Cell coupling in smooth muscle is electrical or mechanical.54,55,69 Electrical coupling propagates the electrical signal (i.e., action potential), heralding contraction of a muscle cell through the gap junctions (and to some extent through simple sarcolemmal appositions) of muscle cells; these junctions (or appositions) provide low-resistance pathways that directly “connect” and allow exchange of ions between sarcoplasms of the adjoined muscle cells. A smooth muscle system endowed with an electrical coupling mechanism behaves functionally as a virtual syncytium through which contraction of the component cells can be elicited in near synchrony. The relatively slower mechanical coupling transmits the force generated in a contracting muscle cell through intermediate junctions with adjacent cells. Contrary to one review,61 cell coupling in normal vesicourethral muscularis is achieved by mechanical cell coupling because its muscle cells have intermediate junctions but no or sparse gap junctions.51,53,60 Active force generated by the contractile apparatus of a muscle cell is discharged to the entire cell surface through insertions of myofilaments into sarcolemmal dense bands, resulting in cell shortening. The tension developed is transmitted through intermediate junctions to adjoined cells, resulting in their deformation and subsequent contraction. Collagen fibrils juxtaposed to the cell surface in the intercellular space contribute to mechanical transmittal of force from the contracting cell to adjacent cells. Summation of minuscule tensions transmitted from cell to cell and from muscle cells to microsepta of interstitium culminates in contraction of entire muscle bundles—and ultimately in contraction of the entire organ in unison—by subsequent force transmittal through partitions of interstitium between the bundles.60
ULTRASTRUCTURE OF THE RHABDOSPHINCTER
The feline rhabdosphincter is composed of a mixture of fast- and slow-twitch myofibers, which have the same distinguishable ultrastructure as ordinary somatic mammalian striated muscle.44 Contrary to one report,70 the human rhabdosphincter appears to be composed of both types of myofibers (Mathews, Light, Wheeler, et al, unpublished data).
The concept of triple (i.e., somatomotor plus dual parasympathetic-sympathetic autonomic) innervation of the feline rhabdosphincter based on histochemical study22 was validated by electron microscopy in the cat,44,71 and its adrenergic autonomic component was also confirmed in humans.45 Two forms of the nerve-myofiber relationship are present in the feline rhabdosphincter with intact innervation. One has the classic sole plate differentiation of the myofiber facing a cholinergic neuroterminal, which is characteristic of somatomotor neuromuscular junctions at large. In the other, a cholinergic or adrenergic axon terminal is closely apposed to the surface of a myofiber and has a functionally plausible, narrow neuromuscular cleft but no sole plate differentiation. These surface contacts are considered autonomic because their morphology is identical to those of autonomic neuroeffector junctions in the vesicourethral muscularis and other mammalian smooth muscle systems and because they persist, despite disappearance of axons innervating sole plates, after bilateral sacral ventral rhizotomy—the known source of somatomotor innervation through the pudendal nerves (discussed later).71–73
Functional Pathology of the Detrusor
Changes in the three microstructural compartments of the detrusor have been described in various forms of voiding dysfunction. Those described in histologic preparations are largely nonspecific and have been of limited usefulness in regard to correlation with the functional abnormalities.56,58 The constellations of changes identified ultrastructurally in different dysfunctions tend to predominate in one compartment or another and are distinctive for each dysfunction.56,58 For this reason, accurate definition of the microstructural correlates of a voiding dysfunction requires study of the smooth muscle, interstitium, and intrinsic neural compartments of the detrusor with equal attention and scrutiny.9,56
NEUROPATHIC VOIDING DYSFUNCTION
Practically all information on the microstructural basis of neuropathic voiding dysfunction has been derived from experimental studies in the cat. These studies have dispelled some traditionally held dogmas and have laid the foundation for a new concept in pathophysiology of the lower motor neuropathic bladder.74–79 According to this concept, abnormal behavior of such a bladder does not merely reflect its release from cephalospinal neural control but is largely the result of dynamic, profound changes in intrinsic nerves and to some extent muscle cells—the two structural elements that jointly determine that behavior. These neuropathic structural changes can be defined fully and accurately only by electron microscopy,79 and they readily distinguish a decentralization from a denervation functional type of lower motor neuropathic bladder. The same studies also validate the concept of triple innervation of the feline rhabdosphincter.71–73,80
The Decentralized Bladder
Occurring beyond and across preganglionic synapses, short-term degeneration of axons duplicates the well-known phenomenon of primary transsynaptic nerve degeneration in the brain81 and represents the first example of this phenomenon in the peripheral autonomic nervous system.74 The axonal degeneration results in loss of cholinergic neuroeffector junctions and is associated with transjunctional degeneration of detrusor muscle cells, a previously unknown phenomenon.76 The term transjunctional
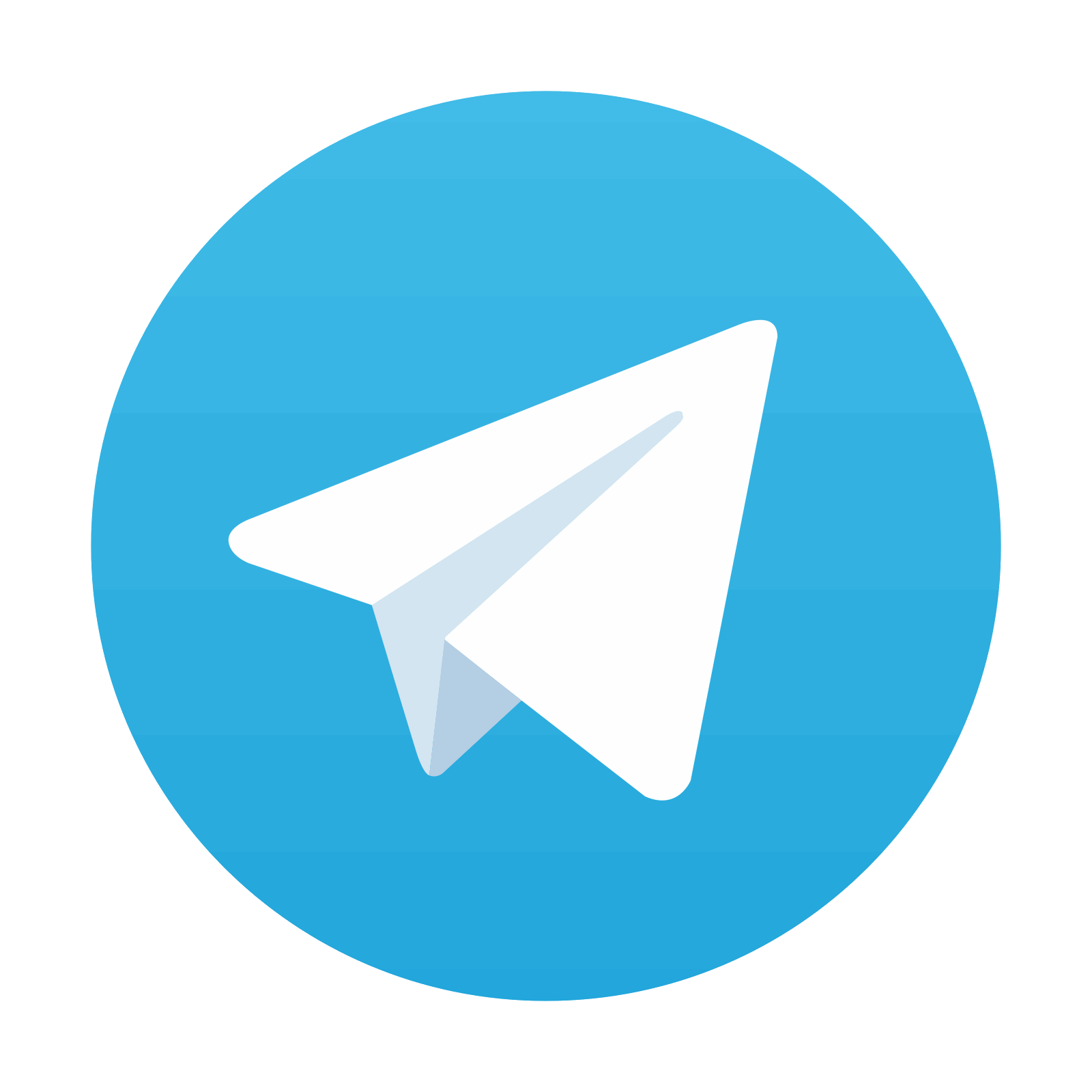
Stay updated, free articles. Join our Telegram channel

Full access? Get Clinical Tree
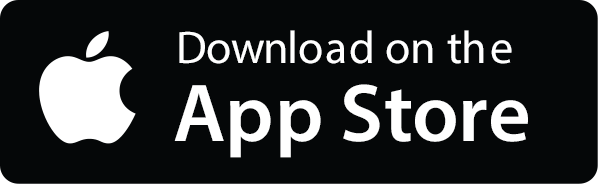
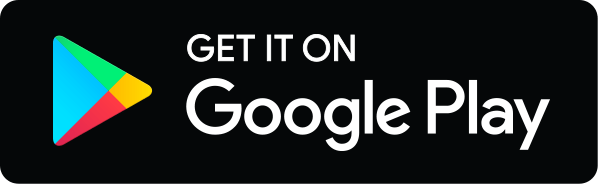