Complex surgical technologies, restricted resident work hours, and limited case volumes in surgical practice have created new challenges to surgical education. At the same time, maintenance of established skills and development of new skills are becoming increasingly important for surgeons, especially skills related to technically challenging minimally invasive surgical therapies. In addition, minimally invasive therapies are highly dependent on uniquely specialized teams of health care workers. For all of these reasons, simulation is gaining attention in surgical education for the development and refinement of minimally invasive surgical skills and technique. This article summarizes developments and challenges related to simulation in surgical education, especially as it relates to minimally invasive surgical therapies in the field of urology.
The rapid development of complex surgical technologies, particularly in minimally invasive therapy, has resulted in challenging learning curves for surgeons. In addition, limited case experience as a result of restricted resident work hours and limited case volumes in surgical practice add additional challenges to surgical education in the 21st century. Meanwhile, surgeons are finding it increasingly important to not just maintain skills, but to develop new skills, especially those new technically challenging skills related to minimally invasive surgical therapies. In addition, minimally invasive therapies are highly dependent on uniquely specialized teams of health care workers. For all of these reasons, simulation is gaining increasing attention as an additional component to the surgical education armamentarium for the development and refinement of minimally invasive surgical skills and technique .
Technical skills education in urology, as in many of the technically oriented fields has its primary roots in the operating room, augmented by cadaver and live animal models and, in select cases, inanimate artificial tissues. In fact “simulators” were used by Susrutha in ancient India in the form of inanimate artificial tissues and physical models . With the advent of digital imaging techniques (CT and MRI), visual-spatial anatomic understanding and “preoperative” planning rose to a whole new level both from a clinical and an education standpoint. With the advent of fiber optics and the video monitor, the trainee and trainer were able to comfortably view the same endoscopic image simultaneously, allowing for more rapid training. Renewed interest in urologic simulation and a paradigm shift in surgical education came about with a series of closely related events. The “To Err is Human” report and the increased public awareness of surgical errors have brought medical care in general under greater scrutiny . Restrictions on residency work hours, the surge of minimally invasive training procedures in urology, and the acute rise in health care costs have all led administrators to seek alternative learning environments to the operating room for training. Technologies offering virtual reality, a term coined in 1987 by Jaron Lanier, opened up new possibilities in medicine for creating a practice environment with intelligent data-collection and mentoring. Unfortunately, this concept was overpromised and underdelivered for many reasons: limitations of computer processing, lack of organized structure and understanding around curriculum design, lack of defined “standards” and metrics, the high cost of development, the multidisciplinary nature of such projects, and a lack of fundamental modeling and validation data during the development process. One of the largest technical challenges is the application of this technology. As much as we like to make the analogy with flight simulation, the human body is not an airplane. The human body is a very complex “model,” with many unknown components, and so is difficult to accurately and consistently simulate. Such complexity becomes magnified when one considers the relatively unknown dynamic impact that the brain has on the body at the microcellular, macrocellular, tissue, physiologic, and behavioral levels.
Simulation is the technique of imitating the behavior of some situation or process by means of a suitable analogous situation or apparatus especially for the purpose of study or training . This has been used extensively in economics, the military, and the aeronautics and space industry and is now becoming an important part of medical education. Learning devices that produce simulation are training simulators. The terms low- and high-fidelity refer to how closely the simulator itself and the environment in which the training occurs represent reality. To be most effective, high-fidelity simulation must lead learners to suspend their disbelief. To do this, the interactive sights, sounds, smells, and feel of the simulated experience must mimic reality and subsequently elicit the emotions and generate the behaviors that would be observed in a real situation. The difference between a low-fidelity simulation and a high-fidelity simulation can be significant. A low-fidelity trainer, for example, may be a simple abstract box-model for use in a nonclinical environment, such as a living room, to practice a procedure. By contrast, the highest-fidelity simulation currently for the same procedure would involve an operation on a live animal in a real operating room under supervision. Anatomic models made in virtual reality represent an attempt to recreate the environment of real tissue. However, unlike either the abstract box model or the live-animal situation, they have the exciting capability of generating data on everything the user does in the environment and of incorporating a virtual instructor. These simulation formats can be used for skills training, task training, and procedure training. They can also provide high reliability team training for such environments as the operating room, the intensive care unit, and the emergency department. Many surgical courses now rely on simple abstract material-based trainers to teach novice surgeons basic skills and techniques. These are excellent tools as long as proper techniques are conveyed. Courses use higher-fidelity tissue or virtual reality trainers to make assessments and provide training for tasks requiring higher-level skills and to provide a means to practice acquired skills. Virtual reality trainers that are software-based and can incorporate curricula, assess formative metrics, recognize errors, and contain management features are gaining more interest as a means of learner-centered, independent, and deliberative practice with feedback.
One of the newest additions to continuing medical education is high reliability team training . Much can be learned by experiencing errors as they occur and studying how these errors can be successfully managed by the team vis-à-vis a debrief exercise. As such, simulation has a profound ability to provide significant advantages as a platform for repetitive practice by both the team and the individual in a no-risk environment away from patients. Simulation technologies can build in every potential error and thereby provide acute-scenario training so that the trainees can learn to recognize, avert, and resolve these events in a no-risk environment. This better prepares them for a similar clinical situation. In addition, virtual reality and other dry-laboratory simulation exercises are helping to reduce the reliance on animal and cadaver laboratories, and have shown the capability to reduce operative times and errors in the operating room .
In clinical teaching, it is essential that the teacher be focused on the patient first and the trainee second. Dedicated facilities with a curriculum embedded with simulation enable teachers to focus all of their attention on learners. The curriculum involves creating a comprehensive knowledge base so that the trainee understands what is to be learned, why it should be learned, and how it can be learned. Of equal importance is teaching the trainee how to recognize an error, why it is an error, and what to do to avoid and recover from the error. It is the combination of basic knowledge and manipulative skills, along with the development of judgment during clinical experiences, that creates the master surgeon. Simulation provides both an opportunity to study and commit to memory how master surgeons attain and perform their skills and an environment by which learners can refine and perfect their skills to achieve such mastery.
The adult learner is a unique student in the environment of health care education. These learners tend to have a problem-centered orientation to their learning and a readiness to learn, as well as increased self-directedness . Residents and fellows as a group of learners comprise a unique group of students who are in Erickson’s seventh stage of development . These middle adult learners have a significant need to develop knowledge and skills, and to be able to apply them effectively to their clinical practice as part of their quest for generativity rather than stagnation. However, as learners, they also have weaknesses and therefore strategies must be devised to overcome these. Research has shown that physicians are pretty awful at self-assessment. It is part of the human condition to suppress our inadequacies to both others and ourselves. As such, these learners like the reward that comes from success and, left to their own devices, gravitate to studying things they enjoy and are already good at. While self-directed learning may be appropriate for an evening pottery class, it is less appropriate for the learning and application of medicine and surgical skills. People increasingly expect doctors to have a reasonable amount of cognitive and technical skill before embarking on the practice of medicine and surgery.
An effective curriculum must clearly identify the goals of the educational program. This is based on a defined desired outcome and a needs assessment that directs the development of the educational program. This assessment generates the specific learning objectives of the curriculum as they relate to the cognitive and skills performance that is to be learned in the training program. The objectives clearly outline not only the material to be covered but also the format that will be used to assess the student for each of the curricular objectives. The curriculum will include a clear delineation of all teaching methods and strategies as they relate to the curricular objectives. Appropriate formative student assessment must be incorporated into the curriculum to provide ongoing feedback to help guide and shape learning, The curriculum must also include summative assessment opportunities to determine if the student has achieved the required level of proficiency before advancing to the next stage of training. Finally, the curriculum must be subject to ongoing evaluation by both the students and the instructors to direct curriculum growth and improvement.
Several researchers have shown that didactic lectures by themselves do not play a significant role in immediately changing physician performance or improving patient care . Education programs that use interactive techniques are generally more effective in changing physician performance or improving patient care outcomes. In addition, educational sessions delivered in a longitudinal or sequenced manner also appear to have a more beneficial impact on physician learning . The provision of enabling methods to facilitate implementation of the new knowledge or skill in the practice setting appears to have a positive effect on the success of medical education programs.
Gallagher and colleagues have clearly shown the importance of the knowledge and psychomotor curriculum in surgical education. Humans have a limited capacity for attention. This limitation becomes extremely important in planning the education of novice surgeons or in learning a new surgical technique. These surgeons are trying to gain additional knowledge while comprehending instruction from their mentor in the operating room. At the same time, they are dealing with the challenges of depth and spatial judgment as this affects their psychomotor performance, especially during minimally invasive therapy. Add to this the demand for operative judgment and decision-making and they may well exceed their attention capacity, which results in their inability to cope with an error or complication. In contrast, the master surgeon has the fundamental knowledge, requires less attention for already well-developed spatial judgment and surgical skills, and therefore can devote more attention to a situation in which an error may be about to occur. Simulation can provide an opportunity for trainees to gain the knowledge and develop better psychomotor and spatial judgment skills so that they too can apply more attention to other skills and learn more about operative judgment and decision–making in the clinical setting. As surgeons, we have long understood our lifelong commitment to education and this concept must be further instilled in our trainees as they become self-directed learners. “Practice makes perfect” is a concept that is well understood by pilots learning to fly, dancers rehearsing complex dance routines, and golfers perfecting their swings. The concept is just as valid in the field of surgery.
Surgical practice is a concept that has been highlighted in a recent report by Kahol and colleagues in their study on the effect of short-term, pretrial practice on surgical proficiency in simulated environments. In this study, subjects performed standardized exercises as a preoperative warm-up, after which the standardized exercises were repeated in a random order. The experimental group was allowed to warm up with the standardized exercises, after which a different task (electrocautery stimulation) was performed. The study participants included experienced laparoscopic surgeons, fellows, and residents. The results of this study provide substantial evidence that short-term practice (warm-up) with exercises designed to target both the psychomotor and cognitive skills involved in surgical procedures can greatly enhance surgical proficiencies during a follow-up procedure. Similar practice techniques enhanced gesture proficiency and made general movements and movements involving tools smoother. Interestingly, the participants of varying experience levels all benefited from the warm-up exercises. Perhaps most importantly, error rates fell significantly following the warm-up exercises. The time required to complete a task, which is one important indicator of efficiency, was also reduced significantly in this study population.
Simulation is also being proposed as an assessment and credentialing tool similar to that in the aviation industry . This proposal comes from a need to measure more than just knowledge, to model real-life situations, and to standardize the assessment of surgeons. Simulation has the potential to provide the “vehicle” to measure skills. However, for simulation assessment to be of value, it must have a scoring system that aligns with expert agreement on what and how a task should be performed and that is subject to strict research to confirm construct and predictive validity. As an appropriate device for surgical evaluation, the curriculum must be able to distinguish the novice from the expert laparoscopic surgeon, or have construct validity. In addition, the simulation-embedded curriculum must be able, on the basis of the performance of the trainee, to predict the surgeon’s performance in the corresponding clinical setting and predict the student’s likelihood of eventual success if being used for screening applicants.
The demonstration of consistent performance at validated criterion levels within the curriculum is a clear indicator that the trainee is ready to advance to the operating room to perform the procedure. The establishment of such criterion levels is often done by having experts go through the curriculum and adjust these based on their performance and consensus . The curriculum will judge proficiency based on such factors as economy of motion, target acquisition, and procedural judgment, rather than just operative time and subjective performance assessment. Hence a surgeon’s advancement up the training ladder would be more precisely determined, thereby providing clarity to trainees and their teachers as to trainees’ skill levels and learning needs, and ensuring that patients are cared for by doctors with documented expertise in the procedures they are planning to perform. Confirmation of predictive validity then allows a simulator device to be used in the certifying and recertifying processes for the subspecialty to determine surgeon competency. Validation studies are essential to determine whether a simulation-embedded curriculum can teach what it is supposed to teach, to demonstrate whether the data generated can distinguish between the novice and the experienced surgeon, and, most importantly, to predict the current and future performance of the surgeon in the clinical operating room.
The development of a comprehensive and effective teaching program depends on the collaborative efforts of many educators across multiple institutions to ensure a robust curriculum that addresses all the needs of the learner, teacher, and end user or patient. Multiple, interactive educational encounters that are longitudinally sequenced will provide more productive learning for the health profession student. Such encounters, combined with immediate, task-specific feedback as to performance and errors, will allow these learners to better learn new skills and effectively integrate them into their clinical practice. However, simultaneous curriculum evaluation is essential to the creation of durable and relevant education programs. It is through evaluation combined with discussion and deliberative inquiry that curriculum development will be achieved. Continuous curriculum revision and renewal can be expected to meet the educational demands of a rapidly advancing, technology-oriented, surgical subspecialty . Curriculum development should be considered a long-term, dynamic, and continuous process that anticipates many revisions and changes to the initial curriculum template. Sustaining the effort of curricular reform will only happen from a combined bottom-up and top-down approach. Commitment at the departmental level by academicians who recognize the advantages of a standardized curriculum and the importance of collaborative communication will lead to the development of robust surgical curricula. The incorporation of appropriate curricular evaluation will allow constructive, proactive response to the expectations of society and the policies of the boards mandating such standardization. The American College of Surgeons’ recent Accreditation of Education Institutes program is an example of this association’s commitment to the incorporation of simulation for surgical residency training programs and the development of dedicated skills training curricula .
The creation of a simulator for training begins with agreement on what is the desired outcome and the development of learning goals and objectives. Learning objectives are specific and contain action verbs describing what learners should be able to do once they have completed the exercise. The simulation tool is designed specifically to meet these objectives . The process of task deconstruction into the learning domains described in Fig. 1 allows for the development and application of the appropriate simulated experience.
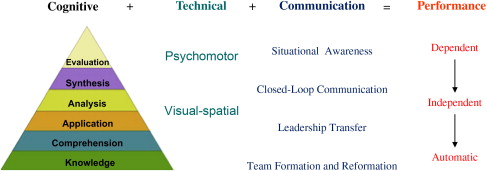
The cognitive domain is underappreciated in surgical education and simulation. It has been estimated that performing an operation properly is 75% decision-making and 25% dexterity . Bloom’s taxonomy describes the transition from the baseline memorization of material (knowledge) to synthesis (comprehension). The ability to appropriately apply this knowledge represents the next level in ascent toward the higher-cognitive functions of analysis, synthesis, and evaluation associated with self-reflection and teaching. Cognitive “simulation” in surgery has existed in a “passive form” for over a century in the form of surgical atlases, for decades in the form of videos, and more recently in the form of the Fundamentals of Laparoscopic Surgery, with its well-validated curriculum and strong cognitive component . It is widely used across the United States in skills centers. With interactive video technology, SimPraxis (Red Llama, Seattle Washington; www.redllamainc.com ) has designed a novel platform that integrates an entire cognitive curriculum for procedures into an interactive multimedia trainer that provides virtual mentorship, error tracking, and scoring .
The technical domain has been a focus of computer-based simulation devices. With a stepwise curricular approach that is not procedure-specific, such basic skills as camera navigation precedes more complex manipulative skills, such as transfer and cutting exercises . Box trainers and abstract virtual reality models have been found to be useful for training these skills. Subsequently, such simple tasks as dissection, clipping, and dividing a vessel can be performed on slightly more complex virtual reality models, inanimate or animate tissue, or human cadaver models. It is only when combined with the cognitive and judgment components of a curriculum that a full procedural trainer can be created. Outside of the standard training on actual anesthetized patients, live animal models remain the gold standard. The role of virtual reality for training advanced surgical procedures continues to evolve. The traditional method of evaluating surgical competence has been the In-Training Evaluation Report, which is filled out at the end of rotations by faculty. Unfortunately, these reports do not provide formative feedback to the trainee. Many programs are shifting toward the Objective Structured Assessment of Technical Skills evaluation whereby the learner is evaluated on multiple domains, such as tissue handling and flow of the operation. This type of assessment is being done in both the operating room and in skills-training laboratories . While operating room competency evaluation in urology residency training programs is encouraged but not required by the Accreditation Council for Graduate Medical Education , programs struggle with whether or not to use the Objective Structured Assessment of Technical Skills (OSATS) evaluation, which is an effective, reliable tool for formative feedback provided immediately in the form of a debrief. OSATS is more effective for guiding the surgical trainee than the less labor-intensive method of summative end-of-the-rotation feedback. Criterion levels and standards are yet to be established by the governing bodies and societies for urology as to competence for specific procedures and, therefore, these are currently established at the individual or institutional level.
If one were to mention the word simulation to an anesthesiologist or an emergency medicine physician, a much different image would come to mind than would come to the mind of the surgeon. Dozens of truly “high-fidelity” simulation scenarios have been designed for mass-casualty and emergent crisis situations. The most common domain studied and practiced in these scenarios pertains to communication. The standardized Objective Structured Clinical Examination allows this domain to be assessed and is commonly used in medical school curricula . Its use during urology residency/credentialing for this domain has been described and is encouraged by the Accreditation Council for Graduate Medical Education . Gettman and colleagues recently published a study using a high-fidelity simulation environment to assess the “communication” domain for delivering the news of an unexpected death to a urology patient’s family. These scenarios can either be done in a simulation center, which has the advantage of a more “standardized” and controlled environment like that of Gettman’s study, or they can be done in situ, as described in work by Riley and colleagues , whereby situational awareness, closed-loop communication, leadership transfer, and team formation and reformation are studied and practiced with artificial scenarios at the actual location where real events normally occur. This approach increases the fidelity and has value for discovering latent conditions.
All of these domains ultimately contribute to performance where the trainee makes the transition from dependence toward independence and finally can perform skills automatically. This process of learning establishes the ability of the trainee to shift more of his or her attention toward more advanced decision-making or “judgment.” While all skill sets have a cognitive component, some clearly rely on the judgmental aspect more than others.
“Skill sets” specific to urology that have been successfully simulated include such cognitive skill sets as pelvic lymph node dissection skills and laparoscopic skills. The fundamentals of laparoscopic surgery contain cognitive training modules to accompany their skills modules that have been well validated by the American College of Surgeons. More recently Red Llama has built and validated an interactive video-based trainer for training pelvic lymph node dissection ( Movie 1: SimPraxis® open pelvic lymph node trainer ∗
∗ Videos for this article can be accessed by visiting www.urologic.theclinics.com . In the online table of contents for this issue, click on “add-ons.”
). Technical skills trainers focusing on the Seldinger technique; endoscopic procedures, such as cystoscopy, transurethral resection, and ureteroscopy; and basic laparoscopic and robotic skills have been designed and are being integrated into curricula primarily focused at the junior urology resident level. Basic open and laparoscopic surgery skills simulation exercises in the form of Objective Structured Assessment of Technical Skills examinations are being administered at select institutions and typically use inanimate tissue models with proctors.Until recently, there has been a general lack of training tools and curriculum development for even the most basic of skill sets in urology. Simulation has primarily consisted of manikin-based catheterization simulators for the female and male urethra as well simulators for digital rectal examinations (Simulution, Prior Lake, Minnesota; www.simulution.com ). However, validation studies are lacking.
The skills necessary to perform flexible ureteroscopy, not unlike skills for other endoscopic procedures, are quite amenable to simulation. Current systems reported in the curriculum of training programs and courses include low-fidelity bench models, such as the one described by Matsumoto , which consists of a urethra represented by a Penrose drain, a bladder represented by an inverted polystyrene plastic cup, and ureters modeled with latex-embedded straws. Commercially available bench models include the Uro-Scopic Trainer (Limbs & Things, Bristol, United Kingdom; www.limbsandthings.com ) and the Scope Trainer (Medi Skills, Edinburgh, United Kingdom; www.mediskills.com ) . McDougall and colleagues have developed a bench-top ureteroscopic model that is being validated ( Movie 2: University of California Irvine (UCI) ureteroscopy trainer ∗ ). Uro Mentor (Simbionix, Lod, Israel; www.simbionix.com ) is the only currently available virtual reality simulator for ureteroscopy ( Movie 3: Uromentor® ureteteroscopy trainer ∗
∗ Videos for this article can be accessed by visiting www.urologic.theclinics.com . In the online table of contents for this issue, click on “add-ons.”
). It allows the user to navigate the ureter and perform various upper urinary tract procedures, such as lithotripsy, laser incision of a stricture, and tumor ablation with tools, including baskets, graspers, intracorporeal lithotripters, guide wires, catheters, stents, biopsy devices, and dilation devices. Several studies have examined its validity and have established that it has educational value at the novice end of the learning curve, but less on the more experienced end . Matsumoto compared performance on Uro Mentor with a high-fidelity bench model and showed a similar level of skill improvement using either training device. Chou and colleagues demonstrated a similar finding in comparing the bench model Uro-Scopic trainer with the Uro Mentor in a group of 16 medical students for teaching basic ureteroscopy skills.Only 11% of urologists obtain their own renal access , even though it has been shown that stone-free rates are higher when a fellowship-trained urologist obtains access compared with rates when a radiologist obtains access . Three training methods have been presented as options for training to acquire the skills needed for using two-dimensional fluoroscopic images to understand three-dimensional space. Such skills are essential for performing percutaneous access to the kidney. Hammond and colleagues describe an innovative model using porcine kidneys with preimplanted artificial stone material in an intact chicken carcass. Using real-time C-arm fluoroscopy, the residents gain needle access, perform dilation, and do fragmentation/extraction of the stone material. Hacker and colleagues took this model one step further by cannulating the ureter, renal artery, and renal vein for continuous perfusion. They surround the “perfused” kidney with ultrasound gel before putting it in the chicken carcass. Acceptability studies of Hammond’s model were encouraging. However, again validation studies are lacking. Strohmaier and Giese also use a porcine renal unit, but describe embedding it in silicone for teaching percutaneous renal access. Medi Skills offers a manikin-based percutaneous-access model. The PercMentor (Simbionix) is a hybrid manikin–virtual reality simulator focused on training the percutaneous renal access portion of the procedure. The force-feedback interface consists of a manikin silicone flank with ribs underneath. The learner controls a virtual C-arm with corresponding virtual fluoroscopic images and a needle is tracked in virtual space with respect to the model. This training model also collects data on cognitive decision-making with regards to choice of instrumentation and choice of calyceal access. Once the needle access is obtained, guidewires, catheters, and tubes are also employed to gain access to the collecting system. These are registered in the virtual space. Knudsen and colleagues prospectively randomized 63 trainees into training versus no training using the PercMentor simulator as the pre- and posttest assessment tool. Performance was assessed using a subjective global rating scale and key metrics generated by the simulator. Subjects who underwent 60 minutes of training on the PercMentor outperformed their peers on PercMentor for both the subjective and objective parameters.
Transurethral resection of the prostate and bladder remain the mainstay skill sets for urologists. Virtual reality cystoscopy models are present as modules in both the Uro Mentor (Simbionix) validated by Gettman and colleagues , the Uro-Trainer (Karl Storz, Tuttlingen, Germany; www.karlstorz.com ), and the SurgicalSIM transurethral resection of the prostate (TURP) simulator (Medical Education Technologies, Inc. [METI], Sarasota, Florida; www.meti.com ). A physical model by Limbs & Things, proposes to train TURP cutting skills on a disposable model and is primarily used for booth demonstrations by endoscopic vendors. Validation of this simulator in educational programs has not been reported. A full TURP skills curriculum is available on the METI’s SurgicalSIM TURP, the commercially available version of the University of Washington TURP trainer designed and validated at the American Urological Association by Sweet and colleagues . This simulator logs important metrics, such as grams resected, blood loss, irrigation used, and critical errors. ( Movie 4: Virtual reality transurethral resection of the prostate skills trainer ∗ ). The Uro-Trainer simulator includes simulation capabilities for TURP and transurethral resection of bladder tumor, but lacks a standardized curriculum. Like the University of Washington TURP trainer, however, the Uro-Trainer has force-feedback and simulates bleeding. Preliminary validity studies have been conducted . Simulution offers a third virtual reality system, PelvicVision for training TURP. Validation studies for this trainer are currently lacking.
The development and validation of trainers for laparoscopic psychomotor skills have primarily stemmed from the general surgery literature, driven by the establishment of laparoscopic cholecystectomy as the standard-of-care procedure for removing gallbladders. The Fundamentals of Laparoscopic Surgery program provides a well-validated, standardized curriculum comprised of cognitive and psychomotor tasks. It has been incorporated into the curricula of many of the American College of Surgeons–accredited simulation centers of excellence. Video-box trainers are also popular and useful for basic tasks, such as navigation, transferring, suturing, and knot tying. These skills can then be transferred to the cadaver models and the human patient . Unfortunately, these low-fidelity models require a trained observer to be in attendance to provide assessment and formative feedback for the trainee. ProMis (Haptica, Dublin, Ireland; www.haptica.com ) also offers a hybrid video box–virtual reality trainer that has undergone rigorous validation studies and has been found to be more acceptable than many of the pure virtual reality trainers ( Movie 5: ProMis® laparascopic skills trainer ∗ ). METI’s SurgicalSIM LTS offers a video-box trainer with a self-contained computer assessment component ( Movie 6: Surgical Sim LTS® laparascopic skills trainer ∗ ). The exercises include peg manipulation, ring manipulation, ductal cannulation, lasso-loop formation and cinching, knot tying, knot integrity, and circle cutting. Virtual reality–based simulation trainers have the distinct advantage of both formative and summative assessment capability. The Minimally Invasive Surgical Trainer–Virtual Reality (MIST-VR; Mentice, Göteborg, Sweden; www.mentice.com ) consists of six abstract tasks focused on training navigation, grasping, translocation, and diathermy. Seymour and colleagues showed for the first time that training on a virtual reality laparoscopic trainer (MIST-VR’s diathermy task) translated to improved performance in diathermy tasks in the operating room. Their randomized blinded-design using videotapes and blinded evaluators was a model study-design for simulation validation studies to follow. While procedure-specific virtual reality models are now only available for general surgery and gynecologic applications, most of the laparoscopic virtual reality platforms provide skills exercises that translate to basic skill sets in urology. Examples of such virtual reality platforms include the SurgiSIM platform (METI) ( Movie 7: Surgical Sim VR laparascopic skills trainer ∗
∗ Videos for this article can be accessed by visiting www.urologic.theclinics.com . In the online table of contents for this issue, click on “add-ons.”
). Surgical Science AB’s LapSim (Surgical Science, Göteborg, Sweden; www.surgical-science.com ), the LaparoscopyVR System (Immersion Medical, Gaithersburg, Maryland; www.immersion.com ), and LapMentor (Simbionix) ( Table 1 ) .