Chapter Outline
BICARBONATE REABSORPTION, 234
BICARBONATE GENERATION, 246
ACID-BASE SENSORS, 256
DIURNAL VARIATION IN ACID EXCRETION, 256
Acid-base homeostasis involves two separate but related processes, bicarbonate reabsorption and new bicarbonate generation. The first relates to the reabsorption of bicarbonate filtered by the glomerulus. The second relates to the need to generate “new bicarbonate” to replenish bicarbonate that is used to neutralize endogenous and exogenous fixed acid loads. Finally, a number of pathophysiologic conditions generate acid or alkali loads that the kidneys must respond to in order to maintain acid-base homeostasis.
Bicarbonate Reabsorption
Bicarbonate reabsorption involves coordinated transport events in multiple nephron segments ( Figure 9.1 ). The proximal tubule reabsorbs the majority of filtered bicarbonate. Little-to-no bicarbonate reabsorption occurs in the thin descending limb of the loop of Henle, moderate reabsorption occurs in the thick ascending limb of the loop (TAL), and the remaining filtered bicarbonate is reabsorbed in distal sites, including the distal convoluted tubule (DCT), connecting segment (CNT), initial collecting tubule (ICT), and collecting duct.

Proximal Tubule
General Transport Mechanisms
Proximal tubule bicarbonate reabsorption involves several distinct, but interconnected, processes ( Figure 9.2 ). First, protons (H + ) are secreted into the luminal fluid. Multiple proteins mediate H + secretion; the apical Na + -H + exchanger, NHE3, and an apical H + -ATPase (hydrogen ion adenosine triphosphatase) are the primary mechanisms of proton secretion in the adult kidney. In the neonatal kidney, the Na + -H + exchanger, NHE8, appears to substitute for NHE3. In the adult kidney, NHE3 is responsible for 60% to 70% of H + secretion, and H + -ATPase accounts for the majority of the remainder.

Secreted H + combines with luminal HCO 3 − to form carbonic acid (H 2 CO 3 ). Luminal carbonic acid dissociates to water (H 2 O) and carbon dioxide (CO 2 ). Although this process can occur spontaneously, the spontaneous dehydration rate is inadequate to support normal rates of proximal tubule bicarbonate reabsorption. The dehydration reaction is catalyzed by carbonic anhydrase IV (CA IV), a membrane-bound carbonic anhydrase isoform present in the proximal tubule brush border.
Luminal CO 2 then moves across the apical plasma membrane into the cell. Although this process has traditionally been thought to occur through lipid-phase diffusion, the integral membrane protein, aquaporin 1 (AQP1), may mediate about 50% of CO 2 transport across the apical plasma membrane. Cytosolic CO 2 is then hydrated, forming carbonic acid, through a process accelerated by the cytosolic carbonic anhydrase, carbonic anhydrase II (CA II). Cytosolic carbonic acid spontaneously dissociates to H + and HCO 3 − , “replenishing” the H + secreted across the apical plasma membrane by apical NHE3 and H + -ATPase.
Cytosolic HCO 3 − is transported across the basolateral plasma membrane. In the S1 and S2 segments of the proximal tubule, the primary HCO 3 − transport mechanism is a sodium-coupled, electrogenic bicarbonate cotransporter, NBCe-1A. Because NBCe-1A is electrogenic, generation and regulation of the transmembrane voltage between cytoplasm and interstitium are important and appear to be related to extracellular pH-dependent activation of the basolateral K + channel, TASK2. In the S3 segment a Na + -dependent, Cl − -HCO 3 − exchanger appears be the primary mechanism of basolateral HCO 3 − transport, although NBCe-1A may also contribute.
In addition to active or secondarily active H + secretion and bicarbonate reabsorption, the proximal tubule exhibits passive H + and bicarbonate transport. Because bicarbonate reabsorption decreases the luminal bicarbonate concentration and increases the luminal H + concentration relative to the peritubular space, this passive transport limits bicarbonate reabsorption and generation of an acidic luminal pH. The molecular mechanisms of bicarbonate backleak are unclear, but several functional aspects are known. It is quantitatively less in newborn than in the adult kidney. The backleak may be regulated; for example, the hormone angiotensin II (Ang II) decreases bicarbonate backleak. Finally, it is known to be partially transcellular and involves specific membrane proteins, but it does not involve NHE3.
Transporters Involved in Proximal Tubule Bicarbonate Reabsorption
Na + -H + Exchangers
Na + -H + exchangers (NHEs) are expressed widely in the kidney, where they function in intracellular pH regulation, transepithelial bicarbonate reabsorption, and vacuolar acidification. All utilize the extracellular-to-intracellular Na + gradient to enable secondary active, electroneutral H + secretion. Although the preferred ions are Na + and H + , Li + can substitute for Na + , and NH 4 + can substitute for H + . The latter process, which enables Na + -NH 4 + exchange, is important in proximal tubule NH 4 + secretion.
NHE3, the primary apical Na + -H + exchanger in the proximal tubule, mediates the majority of luminal bicarbonate reabsorption. Multiple mechanisms regulate NHE3. The best-studied hormonal mechanisms involve parathyroid hormone (PTH), dopamine, and Ang II. Both PTH and dopamine inhibit NHE3 activity, whereas Ang II has a biphasic effect, stimulatory at low concentrations and inhibitory at high concentrations. Both PTH and dopamine increase intracellular levels of cyclic adenosine monophosphate (cAMP), leading to decreased NHE3 activity, and dopamine also has protein kinase C (PKC)–dependent effects. Ang II decreases cAMP levels and activates PKC, tyrosine kinase, and phosphatidylinositol-3-kinase.
NHE3 phosphorylation is an important regulatory mechanism. Serine 552 (in the rat sequence) is a consensus protein kinase A (PKA) phosphorylation site, and phosphorylation of this site causes localization to the coated pit region of the brush border membrane, where NHE3 cannot contribute to bicarbonate reabsorption. Similarly, phosphorylation of rabbit serine 719 regulates insertion into the plasma membrane. Dephosphorylation, mediated by the serine/threonine phosphatase 1 (PP1), but not PP2, at serines 552 and 605, and at other novel phosphorylation sites, stimulates NHE3 activity.
Movement of NHE3 between different subcellular locations, including microvilli, intermicrovillar clefts, endosomes, and the cytoplasm, is an important regulatory mechanism. Only NHE3 in microvilli contributes to bicarbonate reabsorption. Redistribution within these domains is regulated by a variety of factors, including renal sympathetic nerve activity, glucocorticoids and insulin, Ang II, dopamine, and PTH. This process involves a number of cellular proteins, including dynamin, NHE regulatory factor 1 (NHERF-1), clathrin-coated vesicles, calcineurin homologous protein-1, ezrin phosphorylation, G-protein alpha subunits and G-protein beta-gamma dimers.
NHE8 is a second Na + -H + exchanger found in the proximal tubule. Under normal conditions, NHE8 is mostly intracellular in the adult kidney, but in the absence of NHE3, NHE8 expression increases and the exchanger contributes to bicarbonate reabsorption. In the neonatal kidney, NHE8 expression is increased and NHE3 expression is decreased, suggesting that NHE8 is the primary mechanism of apical Na + -H + exchange activity in the neonatal kidney.
H + -ATPase
A second mechanism of proximal tubule apical H + secretion involves the vacuolar H + -ATPase. H + -ATPase is expressed in the brush border microvilli, the base of the brush border, and apical invaginations between clathrin-coated domains. In addition, H + -ATPase acidifies proximal tubule endosomes and lysosomes, senses endosomal pH, and is involved in recruiting trafficking proteins to acidified vesicles, thereby assuring appropriate progression from early endosomes to lysosomes. Proximal tubule H + -ATPase activity is increased by Ang II, increased axial flow, and chronic metabolic acidosis. H + -ATPase has a direct binding interaction with aldolase, which may underlie the development of proximal renal tubular acidosis (RTA) in individuals with hereditary fructose intolerance.
Electroneutral Sodium-Bicarbonate Cotransporter
Basolateral bicarbonate exit largely is mediated by the electroneutral sodium-bicarbonate cotransporter NBCe1-A. In humans, three splice variants of the NBCe1 gene are known; only NBCe1-A, also known as kNBC1, is expressed in the kidney, where it is found exclusively in the basolateral plasma membrane in the proximal tubule. In mice, two additional splice variants exist. NBCe1-A has large cytoplasmic amino- and carboxy-termini tails, 14 transmembrane domains and two glycosylation sites.
NBCe1-A in the proximal tubule mediates the coupled net movement of Na + and HCO 3 − in a 1 : 3 ratio of Na + and HCO 3 − equivalents. Under normal circumstances intracellular Na + and HCO 3 − concentrations are less than peritubular concentrations, meaning that reabsorption via NBCe1-A moves these solutes against their concentration gradients. Because the cytoplasm is negatively charged relative to the peritubular compartment, these electrical gradients provide the moving force. However, the coupling ratio of Na + and HCO 3 − is critically important: a 1 : 3 coupling mediates net HCO 3 − efflux, whereas with a 1 : 2 ratio the net electrochemical gradient favors HCO 3 − influx. Indeed, some cases of proximal RTA appear to result from NBCe1-A mutations that alter the coupling ratio.
Metabolic acidosis increases proximal tubule HCO 3 − reabsorption and basolateral HCO 3 − transport activity but does not alter NBCe1-A expression. Instead, phosphorylation regulates NBCe1-A activity in response to metabolic acidosis. A number of signaling molecules regulate NBCe1-A. These include PKA, cAMP, PKC, Mg 2+ , Ca 2+ , adenosine triphosphate, carbonic anhydrases I through III, inositol 1,4,5-trisphosphate (IP 3 ) receptor (IP 3 R)–binding protein released with IP 3 (IRBIT), and phosphatidylinositol 4,5-bisphosphate.
Defects in NBCe1-A are the most common cause of autosomal recessive proximal RTA (pRTA). In addition to causing severe pRTA, NBCe1 defects can cause growth and mental retardation, basal ganglia calcification, cataracts, corneal opacities (band keratopathy), glaucoma, elevations of serum amylase and lipase, and defects in the enamel suggestive of amelogenesis imperfecta. In mice, homozygous NBCe1-A deletion causes a very severe phenotype, with severe metabolic acidosis, marked volume depletion, and death within a few weeks of birth. Heterozygous deletion gives rise to a milder phenotype but still causes development of pRTA.
Carbonic Anhydrase
Carbonic anhydrases are a family of zinc metalloenzymes that catalyze the reversible hydration of CO 2 to form carbonic acid (H 2 CO 3 ), reaction A in the following equation:
C O 2 + H 2 O ⇔ A H 2 C O 3 ⇔ B H + + H C O 3 −
Carbonic Anhydrase II.
CA II, the predominant carbonic anhydrase in the kidney, is also the predominant carbonic anhydrase in the proximal tubule. It is expressed in the cytoplasm of the proximal tubule, in addition to multiple other sites in the kidney, including thin descending limb, TAL, and intercalated cells. In the mouse kidney, CA II is also expressed in collecting duct principal cells.
Carbonic Anhydrase IV.
CA IV is found in the proximal tubule and in intercalated cells in the collecting duct. CA IV is linked to the plasma membrane via a glycosylphosphatidylinositol lipid (GPI) anchor and extends into the extracellular compartment; the active site is thus extracellular, not intracellular. In the proximal tubule, CA IV is expressed in both apical and basolateral plasma membranes where, by facilitating HCO 3 − interconversion with CO 2 , it contributes to transepithelial bicarbonate reabsorption.
Regulation of Proximal Tubule Bicarbonate Reabsorption
Systemic Acid-Base
Changes in extracellular acid-base status profoundly alter proximal tubule bicarbonate reabsorption. Both metabolic acidosis and respiratory acidosis increase bicarbonate reabsorption, and alkalosis has the opposite effect. These changes occur with both acute and chronic pH changes, although the effect is substantially greater with chronic acidosis. It is important to note that these effects are mediated through changes in interstitial, that is, peritubular, HCO 3 − and pCO 2 . Changes in luminal HCO 3 − have the opposite effect on proximal tubule bicarbonate transport, a manifestation of glomerular-tubular balance.
Studies have begun to elucidate the mechanisms through which extracellular bicarbonate and CO 2 regulate proximal tubule bicarbonate reabsorption. Changes in CO 2 and HCO 3 − , but not changes in pH when the other two components are constant, alter bicarbonate reabsorption. These effects are specific to bicarbonate reabsorption, as fluid reabsorptive rates do not change. At least in part, this process involves members of the ErbB receptor tyrosine kinase family. They also involve the intrarenal angiotensin system, because peritubular CO 2 stimulates intracellular production and luminal secretion of Ang II, which acts through an apical Ang II AT 1 receptor to stimulate bicarbonate reabsorption.
Chronic metabolic acidosis increases proximal tubule bicarbonate reabsorption more than acute metabolic acidosis. This adaptive increase involves increased NHE3 expression and activity and increased H + -ATPase activity, but not detectable changes in NBCe1 expression. Glucocorticoid levels rise with chronic metabolic acidosis, and glucocorticoid receptor activation enhances acidosis-induced increases in NHE3 expression and apical trafficking.
Luminal Flow Rate
Renal bicarbonate reabsorption changes in parallel with glomerular filtration rate and luminal flow. Increased luminal flow enhances apical plasma membrane NHE3 activity. In addition, increased flow minimizes changes in the luminal bicarbonate concentration, thereby maintaining a higher mean luminal bicarbonate concentration, which facilitates bicarbonate reabsorption. Proximal tubule brush border microvilli may function as flow sensors, with drag force transmitted through the actin filament, altering cytoskeletal elements and regulating transport.
Angiotensin II
Ang II is an important regulator of proximal tubule ion transport, including bicarbonate reabsorption. Low Ang II concentrations increase but high concentrations inhibit bicarbonate reabsorption. Both luminal and peritubular low doses of Ang II stimulate bicarbonate reabsorption, mediated predominantly through apical and basolateral AT 1 receptors. An increase in AT 1 receptor expression due to acidosis may contribute to adaptive changes in bicarbonate reabsorption.
Potassium
Chronic hypokalemia stimulates and chronic hyperkalemia inhibits proximal tubule bicarbonate reabsorption. The inhibition is associated with parallel changes in apical Na + -H + exchange and basolateral sodium-bicarbonate cotransport activity and involves increased apical and basolateral plasma membrane AT 1 receptor expression. Acute changes in extracellular potassium concentration, however, do not alter proximal tubule bicarbonate transport.
Endothelin
Endothelin has important and direct effects on ion transport in a variety of renal epithelial cells, including the proximal tubule. Endothelin can be produced in the proximal tubule and exhibits an autocrine effect to stimulate NHE3. In particular, metabolic acidosis–induced increases in NHE3 expression may require endothelin type B (ET-B) receptor activation.
Parathyroid Hormone
PTH immediately inhibits proximal tubule bicarbonate reabsorption through activation of adenylyl cyclase and increased intracellular cAMP production. Short-term systemic PTH administration leads to metabolic acidosis, but long-term administration to metabolic alkalosis. The short-term effect is due primarily to increased urinary bicarbonate excretion, likely as a result of changes in proximal tubule bicarbonate reabsorption; the long-term effect is due to increased titratable acid excretion, which is likely a result of increased excretion of dihydrogen and hydrogen phosphate.
Calcium-Sensing Receptor
The calcium-sensing receptor (CaSR) is present in the apical membrane in the proximal tubule. CaSR activation, through either increased luminal calcium or calcimimetic agents, increases bicarbonate reabsorption through a mechanism likely involving the activation of apical NHE3. CaSR activation may modulate the effects of PTH in proximal tubule bicarbonate reabsorption; hypercalcemia resulting from excess PTH has the opposite effect of PTH alone on bicarbonate transport.
Loop of Henle
The TAL reabsorbs approximately 15% of the filtered bicarbonate load. The overall schema in this loop is fundamentally similar to that in the proximal tubule. Apical Na + -H + exchange and vacuolar H + -ATPase secrete H + . Quantitatively, apical Na + -H + exchange activity is the major H + secretory mechanism; vacuolar H + -ATPase activity is present but has at most a minor role in bicarbonate reabsorption. Two Na + -H + exchanger isoforms are present in the TAL, NHE2 and NHE3, but NHE3 appears to be predominant. Secreted H + reacts with luminal HCO 3 − , forming H 2 CO 3 , which dissociates to CO 2 and H 2 O. Whether luminal CA IV is present is unclear, as reports in the literature are conflicting. Luminal CO 2 moves down its concentration gradient across the apical plasma membrane into the cell cytoplasm. Cytoplasmic CA II catalyzes CO 2 hydration to form H 2 CO 3 , which dissociates to H + and HCO 3 − , thereby recycling the H + secreted across the apical plasma membrane. Cytosolic HCO 3 − exits via basolateral Cl − /HCO 3 − exchange—possibly anion exchanger 2 (AE2)—via an electroneutral sodium-bicarbonate cotransporter (NBCn1), and possibly via basolateral Cl − channels.
Several plasma membrane proteins either directly or indirectly alter bicarbonate reabsorption. Inhibiting the apical Na + -K + -2Cl − cotransporter, NKCC2, increases bicarbonate reabsorption. This increase may occur because inhibiting NKCC2 decreases Na + entry, thereby decreasing intracellular Na + and increasing the Na + uptake gradient for apical Na + -H + exchange. Inhibiting basolateral Na + -H + exchange activity decreases bicarbonate reabsorption through cytoskeletal alterations that reduce apical NHE3 expression.
Regulation of Thick Ascending Limb Bicarbonate Reabsorption
A variety of stimuli regulate TAL bicarbonate reabsorption. Metabolic acidosis increases TAL bicarbonate reabsorption, but whether the effects are specific to metabolic acidosis or due to other mechanisms is not clear. Metabolic acidosis induced by NH 4 Cl and chloride loading with NaCl have similar effects on bicarbonate transport, raising the possibility that chloride loads, not acid loads, regulate TAL bicarbonate transport. Support for acidosis regulation of TAL bicarbonate transport comes from the finding that NH 4 Cl-induced metabolic acidosis, but not equivalent chloride loading with NaCl, increases TAL NHE3 expression. Further supporting a role of the TAL in acid-base regulation is the observation in experimental models that metabolic alkalosis decreases bicarbonate reabsorption.
Several hormones regulate bicarbonate reabsorption. Ang II stimulates TAL bicarbonate reabsorption, likely through activation of AT 1 receptors. Glucocorticoid receptors are present in the TAL, and glucocorticoids are necessary for normal bicarbonate reabsorption. Mineralocorticoids, at high concentrations, stimulate bicarbonate reabsorption, but their absence does not alter basal transport. Arginine vasopressin (AVP) inhibits bicarbonate reabsorption through prostaglandin E 2 –mediated inhibition of apical Na + -H + exchange activity. PTH inhibits bicarbonate reabsorption, but the effect is less than the effect of AVP.
Cytokines regulate bicarbonate transport. Lipopolysaccharide (LPS) inhibits transport; this effect involves the cytokine receptor Toll-like receptor-4 (TLR4) as well as separate pathways activated by luminal and peritubular LPS. Luminal LPS involves the mTOR (mammalian target of rapamycin) pathway, whereas peritubular LPS functions through the MEK/ERK (mitogen-activated protein kinase/extracellular signal–regulated kinase) pathway.
Another important regulatory factor is the medullary osmotic gradient. Increased tonicity inhibits and decreased tonicity stimulates bicarbonate reabsorption; these effects occur through phosphatidylinositol 3-kinase–mediated changes in apical Na + -H + exchange activity. AVP, which contributes to the development of the medullary osmotic gradient, inhibits bicarbonate reabsorption.
Acid-Base Transporters in the Thick Ascending Limb
Many of the major H + and HCO 3 − transporters were discussed previously in relation to the proximal tubule and are not described again here.
Electroneutral Sodium-Bicarbonate Transporter 1
NBCn1 facilitates the electroneutral, coupled transport of Na + and HCO 3 − in a 1 : 1 ratio. In the kidney, NBCn1 is found in the basolateral plasma membrane in the TAL, the intercalated cells of the outer medullary collecting duct (OMCD), and the terminal inner MCD (IMCD). Because the concentrations of Na + and HCO 3 − are generally lower in the cytoplasm than in the interstitium, basolateral NBCn1 likely mediates peritubular HCO 3 − uptake. Moreover, both metabolic acidosis and hypokalemia increase TAL NBCn1 expression. Thus, NBCn1 is unlikely to mediate a critical role in bicarbonate reabsorption. Instead, it is likely to contribute to ammonia reabsorption, as discussed later.
Distal Convoluted Tubule
The DCT consists of two cell types, DCT cells and intercalated cells, which employ different mechanisms of bicarbonate reabsorption. DCT cells express apical NHE2, and NHE2 inhibitors decrease bicarbonate reabsorption. Basolateral HCO 3 − exit likely involves AE2. A basolateral Cl − channel that has limited HCO 3 − permeability may also contribute. Cytosolic CA II is present, but not apical CA IV. In the late DCT intercalated cells are present. Quantitatively, intercalated cells constitute only a very small proportion of all cells in the DCT, about 4% and 7% in mouse and rat kidneys, respectively. The majority of intercalated cells in the DCT are type A and non-A, non-B intercalated cells (see “ Cell Composition ”).
Collecting Duct
The renal collecting duct, the final site of bicarbonate reabsorption, has the capacity to both reabsorb and secrete luminal bicarbonate. Specific proteins in specific epithelial cell types, which vary in type and frequency in different collecting duct segments, mediate these processes.
Collecting Duct Segments
Technically, the collecting duct begins with the initial collecting tubule (ICT), immediately distal to the CNT, and extends through the IMCD. The CNT arises from a different embryonic origin from that of the ICT and the remainder of the collecting duct. However, the CNT is included in the discussion of the role of the collecting duct in acid-base regulation because it has cell types and acid-base transport mechanisms similar to those in the collecting duct. Different portions of the collecting duct are identified by where they reside: cortical collecting duct (CCD), outer medullary collecting duct in the outer stripe (OMCDo), outer medullary collecting duct in the inner stripe (OMCDi), and the IMCD.
Cell Composition
Collecting duct segments contain several distinct epithelial cell types, and the cellular composition differs in the various collecting duct segments. Two distinct cell types, intercalated cells and principal cells, are present. Principal cells account for about 60% to 65% of cells, and intercalated cells account for the remainder in the ICT, CCD, and OMCD. The proportion of intercalated cells is less in the IMCD and decreases progressively as one moves from the outer medullary–inner medullary junction to the papillary tip. In the terminal IMCD, the epithelium is composed of IMCD cells, a cell distinct from both intercalated cells and principal cells. The CNT contains both intercalated cells and a cell type specific to the CNT, termed the connecting segment cell; in some species, principal cells are also present.
At least three distinct intercalated cell subtypes exist; the type A intercalated cell, the type B intercalated cell, and the non-A, non-B intercalated cell ( Figure 9.3 ). In the CNT, both type A and non-A, non-B intercalated cells are present, and type B intercalated cells are uncommon. In the CCD, both type A and type B intercalated cells are present, and the non-A, non-B cell is uncommon. In the OMCD and IMCD, only the type A intercalated cell is present under normal conditions.

Type A Intercalated Cell
The type A intercalated cell is involved in H + secretion, HCO 3 − reabsorption and ammonia secretion. The proteins involved in these processes are, in general, different from those in the proximal tubule and TAL ( Figure 9.4 ).

Both vacuolar H + -ATPase and P-type H + -K + -ATPases are involved in H + secretion. H + -ATPase is abundant in the apical plasma membrane and in apical cytoplasmic tubulovesicles in type A intercalated cells. H + -ATPase undergoes redistribution between the cytoplasmic compartment and the apical plasma membrane; this mechanism, rather than changes in total protein expression, appears to be the major adaptive response to acid-base disturbances. In addition to having a major role in H + secretion, H + -ATPase also has an essential role in cell volume regulation and maintenance of intracellular electronegativity, replacing the Na + -K + -ATPase which provides these functions in most other cell types.
A second means of H + secretion is electroneutral, depends on luminal K + , and is mediated by P-type H + -K + -ATPase proteins. At least two H + -K + -ATPase α-isoforms are present. One, HKα 1 , is similar to the α-isoform involved in gastric acid secretion. The other, HKα 2 , is similar to the α-isoform in the colon. K + reabsorbed via apical H + -K + -ATPase can either recycle across the apical plasma membrane or exit the cell across the basolateral plasma membrane, and relative movement across the apical versus basolateral plasma membranes is regulated by dietary K + intake.
A truncated isoform of the erythrocyte anion exchanger, the kidney anion exchanger (kAE1), is present in the basolateral plasma membrane and mediates basolateral bicarbonate exit. Cl − that enters the cell via basolateral Cl − -HCO 3 − exchange exits via the KCl cotransporter, KCC4 ; a basolateral Cl − channel, presumably ClC-Kb in humans and ClC-K2 in rodents, also contributes to Cl − recycling.
Cytoplasmic CA II is abundant in type A intercalated cells and enables intracellular generation of H + , for apical secretion, and HCO 3 − , for basolateral transport. In addition, membrane-associated carbonic anhydrases are present in the apical region (CA IV) and, at least in mouse and rabbit, in the basolateral region (CA XII) of intercalated cells.
Type B Intercalated Cell
The type B intercalated cell mediates a major role in HCO 3 − secretion and luminal Cl − reabsorption. It contains basolateral H + -ATPase and an apical Cl − -HCO 3 − exchanger, pendrin. H + -ATPase is also present in vesicles throughout the cell, but it is not present in the apical plasma membrane. As in type A intercalated cells, H + -ATPase, rather than Na + -K + -ATPase, prevents cell swelling and maintains intracellular electronegativity in type B cells. Type B intercalated cells also express H + -K + -ATPase. In the rabbit and mouse an apical H + -K + -ATPase activity is present, whereas in the rat a basolateral H + -K + -ATPase activity has been suggested. The type B cell also has cytoplasmic CA II, which facilitates intracellular H + and HCO 3 − production. Figure 9.4 summarizes the proteins involved in type B intercalated cell acid-base transport.
The type B intercalated cell also has the ability to secrete H + and reabsorb luminal HCO 3 − . As noted previously, most studies indicate that the type B intercalated cell has an apical H + -K + -ATPase activity, and functional studies have shown that all CCD intercalated cells with apical Cl − -HCO 3 − exchange activity, that is, all type B intercalated cells, also have basolateral Cl − -HCO 3 − exchange activity.
The type B intercalated cell has several roles in acid-base and ion transport homeostasis. Genetic deletion of apical pendrin impairs HCO 3 − secretion, luminal Cl − reabsorption, and, through a mechanism involving coordinated function with the principal cell, luminal Na + reabsorption. The type B intercalated cell may also contribute to H + secretion and luminal HCO 3 − reabsorption. Ammonia, which is increased in metabolic acidosis and hypokalemia, increases apical H + -K + -ATPase and basolateral Cl − -HCO 3 − activity, a change that would result in increased net HCO 3 − reabsorption.
Non-A, Non-B or Type C Intercalated Cell
A third intercalated cell subtype, generally termed the non-A, non-B cell (sometimes called the type C cell), is present in the CNT and ICT. This cell has several features that distinguish it from both type A and type B intercalated cells. These differences include the expression of both pendrin and H + -ATPase in the apical plasma membrane and in apical cytoplasmic vesicles, the absence of basolateral plasma membrane H + -ATPase and basolateral AE1, the presence of apical but not basolateral Rhesus (Rh) glycoprotein Rhcg, and the absence of basolateral Rhbg (see Figure 9.3 ). Thus, this cell differs significantly from both type A and type B intercalated cells. Studies of the developing kidney show that non-A, non-B cells and type B intercalated cells arise simultaneously, but from different foci. This cell type was termed “non-A, non-B cell” in early studies. However, its unique transporter expression, distribution, and developmental origin suggest it is a third distinct intercalated cell subtype.
Principal Cells
Principal cells have indirect and direct roles in acid secretion. Indirectly, principal cell–mediated Na + reabsorption leads to luminal electronegativity, which facilitates H + secretion by the electrogenic, and thus voltage-sensitive, H + -ATPase. In addition, principal cells have direct roles. Functional studies show that principal cells have apical H + secretory and basolateral Cl − -HCO 3 − exchange activities, and they express H + -ATPase and both the HKα 1 and HKα 2 isoforms of H + -K + -ATPase. In the mouse and rat kidney, principal cells in the OMCDi and IMCDi express both carbonic anhydrase activity and CA II protein. Finally, the ammonia transporters Rhcg and Rhbg are both present in principal cells in the CCD and OMCD.
IMCD Cell
The IMCD cell is a distinct cell type and is the predominant cell present in the terminal IMCD. It exhibits carbonic anhydrase activity, both H + -ATPase and H + -K + -ATPase activity, and basolateral Cl − -HCO 3 − exchange. In vitro microperfusion studies have demonstrated directly that the IMCD secretes H + and reabsorbs luminal HCO 3 − .
Functional Role of Different Collecting Duct Segments
Connecting Tubule and Initial Collecting Tubule
Relatively little information is available on the functional role of the CNT and ICT in acid-base homeostasis. Morphologic and immunolocalization studies suggest that the CNT and ICT contain type A, type B, and non-A, non-B intercalated cells. Under basal conditions, the CNT, at least in the rabbit, secretes bicarbonate through a Cl − -, carbonic anhydrase–, and H + -ATPase–dependent mechanism that likely involves apical pendrin, cytosolic CA II, and basolateral H + -ATPase.
Cortical Collecting Duct
Unlike the OMCD and IMCD, which can only secrete acid, the CCD both reabsorbs and secretes bicarbonate. The basal direction of bicarbonate transport varies among species, but both bicarbonate absorption and secretion can be induced in response to systemic acid or alkali loading. The ability to secrete bicarbonate, which is not found in the OMCD or IMCD, correlates with the presence of type B intercalated cells in the CCD, but not in the OMCD or IMCD. Mineralocorticoids stimulate CCD bicarbonate secretion, likely in relation to generation of metabolic alkalosis and to stimulation of pendrin expression.
Outer Medullary Collecting Duct
The OMCD is responsible for approximately 40% to 50% of the net acid secretion in the collecting duct. Both intercalated cells and principal cells contribute to acid secretion, although intercalated cells are believed to be the primary type responsible for OMCD acid secretion.
Inner Medullary Collecting Duct
The IMCD secretes H + and reabsorbs luminal bicarbonate. However, the number of type A intercalated cells is substantially less than in other collecting duct segments. In the rat they account for only 10% of cells in IMCD1, and in all species examined the prevalence diminishes distally such that no intercalated cells exist in the distalmost portion of the IMCD (IMCD3). Nonetheless, bicarbonate reabsorption occurs in the terminal IMCD and basolateral Cl − -HCO 3 − exchange is present in cultured IMCD cells. H + secretion is at least partly mediated by H + -K + -ATPase. In rats fed a potassium-deficient diet, H + -K + -ATPase activities ware upregulated, but H + -K + -ATPase accounts for only about 50% of bicarbonate reabsorption in the IMCD, indicating that other mechanisms of luminal acidification also contribute, likely including H + -ATPase. The IMCD expresses carbonic anhydrase IV and luminal, cytoplasmic, and lateral membrane–associated carbonic anhydrase activity has been reported.
Proteins Involved in Collecting Duct H + /Bicarbonate Transport
Collecting duct H + and HCO 3 − transport involves the coordinated activity of multiple transporters in conjunction with specific carbonic anhydrase isoforms. This section reviews the specific proteins involved.
H + -ATPase
Electrogenic apical H + secretion in acid-secreting intercalated cells and basolateral proton transport by bicarbonate-secreting intercalated cells are mediated by the vacuolar H + -ATPase. Intercalated cells in the OMCD and initial IMCD and type A intercalated cells in the CCD contain H + -ATPase in the apical plasma membrane and in an apical cytoplasmic vesicle pool, and redistribution between the cytoplasmic vesicle pool and the apical plasma membrane is a major mechanism regulating H + secretion. H + -ATPase is also present in the apical region of principal cells and connecting segment cells, but its expression is much less than in intercalated cells. The role of apical H + -ATPase in nonintercalated cells has not been clearly defined; it may be involved in endosomal trafficking and fusion, and in the OMCDi it can also mediate apical H + secretion.
Vacuolar H + -ATPase is an assembly of multiple subunits that form two main domains; the V 1 domain is extramembranous and hydrolyzes ATP, and the V 0 domain is transmembranous and transports protons. The V 0 domain is composed of six subunits; the V 1 domain is composed of eight subunits and is linked to the V 0 domain via a stalk region comprising subunits from both V 0 and V 1 . Distinct isoforms and splice variants have been identified for many of these H + -ATPase subunits and their cell specific distribution may contribute to cell specific regulation of proton and bicarbonate transport.
Genetic defects in several H + -ATPase subunit mutations can cause distal RTA (dRTA) in humans. Defects in the B1 subunit in the hydrolytic V 1 domain resulting from ATP6V1B1 gene mutations can produce early-onset hearing loss with autosomal recessive, severe dRTA. Mice with B1 subunit deletion have incomplete dRTA. In these mice, the B2 subunit can substitute partially for the B1 subunit, enabling partial compensation. Mutations in the a4 subunit (ATP6V0A4) in the H + -translocating V 0 domain also produce recessive, severe early-onset dRTA, with variable onset of hearing loss.
H + -K + -ATPase
The second mechanism of collecting duct H + secretion involves electroneutral H + -K + exchange. The active protein is a heterodimer composed of α- and β-subunits. The α-subunit is an integral membrane protein with multiple membrane-spanning domains and contains the catalytic portion of the enzyme. Two α-subunit isoforms have been identified. HKα 1 , also termed the gastric isoform, was identified originally in the stomach. HKα 1 forms heterodimers with its specific β-subunit, HKβ. The β-subunit has only a single membrane-spanning region and is necessary for targeting of the α-subunit to the plasma membrane and for transport function. HKα 2 was identified originally in the colon and is also referred to as the colonic isoform . Three splice variants of HKα 2 have been identified in the kidney. HKα 2 forms heterodimers with the β 1 -subunit of Na + -K + -ATPase.
HKα 1 , HKα 2 , and HKβ are expressed throughout the collecting duct, with greater expression in intercalated cells than in principal cells. Physiologic studies suggest that both HKα 1 and HKα 2 are present in type A as well as type B intercalated cells. However, immunohistochemistry studies have yielded variable results with respect to the precise cellular distribution of the HKα 1 and HKα 2 isoforms. HKα 1 immunoreactivity was found in both AE1-positive (type A) and AE1-negative intercalated cells in both rat and rabbit kidneys, but in human kidneys, diffuse HKα 1 immunoreactivity was present in both intercalated and principal cells. HKα 2 immunoreactivity was consistently apical, but in different cell types in different studies. It was found exclusively in the connecting segment cell in rabbits in one study and exclusively in the OMCD principal cell in rats in another. A third study found the splice variant, HKα 2c , in intercalated cells, principal cells, and connecting segment cells from the CNT through the initial IMCD in rabbit kidneys.
Multiple physiologic conditions alter H + -K + -ATPase expression and activity. Metabolic acidosis increases H + -K + -ATPase activity in the CCD and HKα 1 and HKα 2 mRNA expression in the OMCD, suggesting that H + -K + -ATPase contributes to H + secretion. Specific studies have identified apical, but not basolateral, H + -K + -ATPase activity in both types A and type B intercalated cells in mouse and rabbit kidney. Extracellular ammonia, which increases with both metabolic acidosis and hypokalemia, enhances apical H + -K + -ATPase–mediated H + secretion in both type A and type B intercalated cells in the CCD.
Pendrin is an electroneutral Cl − -HCO 3 − exchanger present exclusively in type B and non-A, non-B intercalated cells. It is found in the apical plasma membrane and in apical cytoplasmic vesicles in type B and non-A, non-B intercalated cells in the CNT, ICT, and CCD. Under basal conditions, pendrin is predominantly expressed in the apical plasma membrane in non-A, non-B intercalated cells and in subapical cytoplasmic vesicles in type B intercalated cells, and redistribution between these two sites is an important regulatory mechanism. Pendrin is regulated by Ang II, nitric oxide, and cAMP. In addition to bicarbonate secretion, pendrin mediates an important role in extracellular fluid volume and blood pressure regulation. This role appears to involve participation in both transcellular Cl − reabsorption and, through luminal alkalinization due to HCO 3 − secretion, activation of the principal cell epithelial Na + transporter, ENaC.
Carbonic Anhydrase
Three carbonic anhydrase isoforms, CA II, CA IV, and CA XII, are present in the collecting duct. CA II is cytosolic in proximal tubular cells, discussed previously, in intercalated cells, and in principal cells in mouse collecting ducts. CA II is present in all intercalated cell types, but expression is generally greater in the type A than in type B intercalated cells.
CA IV is an extracellular, membrane-associated carbonic anhydrase tethered to the membrane through a glycosylphosphatidylinositol (GPI) lipid anchoring protein. It is expressed apically in the majority of cells in the OMCD and IMCD and in type A intercalated cells in the CCD in rabbits. In the OMCDi, luminal carbonic anhydrase inhibition decreases bicarbonate absorption, suggesting an important role for CA IV in acid-base homeostasis.
CA XII is another extracellular, membrane-associated carbonic anhydrase found in the collecting duct. In contrast to CA IV, CA XII is an integral membrane protein with a single membrane-spanning region. Basolateral CA XII immunoreactivity has been reported in principal cells in human kidneys, and in the mouse, basolateral CA XII immunoreactivity is found in type A intercalated cells in the CCD and OMCD.
Kidney Anion Exchanger 1
The major basolateral anion exchanger in type A intercalated cells is kAE1. In the human, rat, and mouse kidney kAE1 is expressed almost entirely in the basolateral plasma membrane. In the rabbit kidney under basal conditions, kAE1 is present in intracytoplasmic multivesicular bodies as well as in the basolateral plasma membrane; metabolic acidosis decreases intracellular kAE1 and increases basolateral kAE1, suggesting that regulated trafficking contributes to bicarbonate reabsorption.
Several mutations in AE1 cause human autosomal dominant and autosomal recessive dRTA. Autosomal dominant dRTA can be caused by a trafficking defect leading either to mistargeting to the apical plasma membrane or to failure of plasma membrane insertion. Autosomal recessive dRTA due to AE1 mutation is commonly due to mutations that lead to intracellular protein retention.
KCl cotransporter KCC4 is a member of the SLC12 family of solute transporters and mediates electroneutral, coupled transport of K + and Cl − . Basolateral KCC4 expression has been shown in the proximal convoluted tubule (PCT), TAL, DCT, CNT, and type A intercalated cells. In the type A intercalated cell, KCC4 likely contributes to basolateral Cl − recycling. Metabolic acidosis increases KCC4 expression in type A intercalated cells in the OMCD, suggesting a role in the response to metabolic acidosis, and KCC4 deletion causes development of dRTA, suggesting that KCC4 is necessary for both basal and acidosis-stimulated acid-base homeostasis.
Cl − Channel
Cl − entering via basolateral kAE1 recycles across the basolateral plasma membrane. In addition to KCC4, the Cl − channel, ClC-K2, is present in the basolateral plasma membrane of type A intercalated cells and likely contributes to this recycling.
Other Anion Exchangers
Several other anion transporters, including anion exchangers and sodium bicarbonate cotransporters, are present in the collecting duct, but their roles in acid-base homeostasis are less completely understood. AE2 is expressed in collecting ducts, particularly in the basolateral plasma membrane of IMCD cells. Another Cl − -HCO 3 − exchanger, Slc26a7, is found in the basolateral plasma membrane of OMCD intercalated cells. Slc26a7 messenger RNA (mRNA) and protein expression increases with acid loading, suggesting that it may contribute to regulated bicarbonate reabsorption. Anion exchanger AE4 (Slc4a9) has been reported in the collecting duct, but both its location and function are in question. One study reported that AE4 immunoreactivity is exclusively apical in type B intercalated cells in rabbit kidneys, whereas another found apical and lateral AE4 immunoreactivity in rabbit type A intercalated cells, and basolateral immunoreactivity in both type A and type B intercalated cells in the rat CCD.
Sodium-Bicarbonate Cotransporters
Several sodium bicarbonate cotransporters (NBCs) are expressed in the collecting duct. NBC3 (Slc4a7) is found in the apical region of OMCD intercalated cells and type A intercalated cells in the CCD and in the basolateral region of type B intercalated cells. It appears to contribute to intracellular pH regulation but not to transepithelial bicarbonate transport. As previously mentioned, NBCn1, also an SLC4A7 gene product, is an electroneutral sodium-bicarbonate cotransporter, and its basolateral expression is found in the terminal IMCD and in OMCD intercalated cells. NBCe2 (Slc4a5), an electrogenic sodium-bicarbonate cotransporter, is apical in intercalated cells in the medullary collecting duct.
Regulation of Collecting Duct Acid-Base Transport
The collecting duct is the final site controlling renal acid-base regulation. It responds quickly to physiologic conditions to increase acid or bicarbonate excretion as needed to maintain systemic acid-base homeostasis.
Acidosis
The collecting duct response to metabolic acidosis includes adaptations in all segments of the collecting duct and the connecting segment. Increased acid secretion in the collecting duct during acidosis is mediated primarily by H + -ATPase. Both metabolic acidosis and respiratory acidosis increase apical plasma membrane H + -ATPase expression and activity in acid-secreting collecting duct intercalated cells. Redistribution of H + -ATPase from a subapical vesicle pool to the apical plasma membrane is the primary means of activation of proton secretion, and it involves vesicular trafficking that requires SNARE (soluble N -ethylmaleimide-sensitive factor attachment protein receptor) proteins and Rab GTPases (guanosine triphosphatases). In most models of metabolic acidosis, total renal H + -ATPase mRNA and protein expression do not change, but examination of OMCD segments from acid-loaded mice found increased mRNA expression of several H + -ATPase subunits, including the β1 and α4 subunits.
During metabolic acidosis, AE1 mRNA and AE1 protein expression in the basolateral plasma membranes of OMCD and CCD type A intercalated cells is increased. In rats and mice, AE1 is present in the basolateral membrane under basal conditions, and the subcellular distribution does not change with metabolic acidosis. In rabbits fed a normal diet, AE1 is in both intracellular multivesicular bodies and in the basolateral plasma membrane in type A intercalated cells. Metabolic acidosis increases the amount of AE1 immunoreactivity in the basolateral plasma membrane and reduces intracellular AE1.
During metabolic acidosis, both net HCO 3 − secretion and type B intercalated cell–mediated unidirectional HCO 3 − secretion decrease. This decrease is associated with reductions of pendrin expression in type B and non-A, non-B cells as well as of apical Cl − -HCO 3 − exchange activity in type B intercalated cells in the CCD. Reduced bicarbonate secretion by type B cells during acid loading thus contributes to increased net bicarbonate reabsorption.
Carbonic anhydrase activity and the expression of CA II and CA IV in the collecting duct are increased by metabolic acidosis. CA IV expression is upregulated in the OMCD, whereas CA II expression is upregulated in the CNT, CCD, and OMCD.
The collecting duct response to respiratory acidosis appears to be similar to that to metabolic acidosis. Respiratory acidosis stimulates structural changes in OMCD and CCD type A intercalated cells that are consistent with translocation of H + -ATPase–bearing membrane from the apical vesicle pool to the apical plasma membrane. Respiratory acidosis also stimulates N -ethylmaleimide (NEM)–sensitive ATPase activity and bicarbonate reabsorption in isolated CCDs, a finding consistent with activation of H + -ATPase–mediated proton secretion. In addition, chronic respiratory acidosis increases kAE1 mRNA. Pendrin expression decreases during respiratory acidosis, a decrease that likely mediates decreased bicarbonate secretion.
Alkalosis
Metabolic alkalosis induces coordinated changes in acid-base transport throughout the collecting duct. In the OMCD of bicarbonate-loaded animals, bicarbonate reabsorption is lower than in control animals and in the IMCD, bicarbonate loading abolishes acid secretion. In the CCD, bicarbonate loading in animals produces net bicarbonate secretion. However, no studies have shown the development of HCO 3 − secretion by the OMCD or the IMCD in response to metabolic alkalosis, and absence of this development correlates with the lack of pendrin-expressing type B and non-A, non-B intercalated cells in these segments.
The cellular response to alkalosis in OMCD and CCD type A cells entails essentially the reverse of processes that occur to stimulate acid secretion. H + -ATPase is redistributed from the apical plasma membrane into the apical vesicle pool, and basolateral AE1 immunoreactivity decreases. Depending on the animal model, alkalosis increases pendrin expression and its apical distribution in type B and non-A, non-B intercalated cells as well as pendrin-mediated CCD bicarbonate secretion. However, pendrin expression, subcellular location, and functional activity are regulated by other factors independent of acid-base status, particularly chloride balance and luminal chloride delivery.
Hormonal Regulation of Collecting Duct Acid-Base Transport
In addition to extracellular pH, multiple other factors regulate collecting duct acid-base transport. Importantly, in vivo acid-base changes cause greater adaptations than equivalent in vitro changes, suggesting that in vivo regulatory mechanisms mediate a critical role in the response to acid-base disturbances. Several hormones and receptors regulate bicarbonate transport in the collecting duct, particularly aldosterone and its analogs, and Ang II.
Aldosterone is an important regulator of collecting duct bicarbonate transport. Both in vivo and in vitro mineralocorticoids increase OMCD bicarbonate reabsorption. This increase involves, at least in vitro, increased H + -ATPase activity and apical translocation in OMCD intercalated cells, stimulated through a nongenomic pathway not inhibited by mineralocorticoid receptor blockade. Mineralocorticoids also increase CCD bicarbonate secretion; this increase depends on luminal chloride, is mediated by pendrin, and involves increased pendrin mRNA and protein expression and pendrin redistribution from cytoplasmic vesicles to the apical plasma membrane in type B intercalated cells. Probably because of parallel stimulation of both acid and bicarbonate secretion, mineralocorticoid therapy usually has modest effects on systemic acid-base homeostasis.
Ang II exerts effects on the proximal tubule, TAL, DCT, and collecting ducts. The collecting duct expresses apical AT1 (AT 1a ) receptors in both principal cells and intercalated cells. In mouse OMCD and CCD, Ang II in vitro increases H + -ATPase activity in acid-secreting intercalated cells by trafficking H + -ATPase to the apical plasma membrane. In mouse OMCD, Ang II stimulates H + -ATPase activity through a G protein–coupled PKC pathway. However, in other studies, Ang II in vivo and in vitro decreased bicarbonate reabsorption in rat OMCD but Ang II in vitro decreased H + -ATPase activity via AT1 receptors ; this apparent discrepancy has not been resolved.
Endothelin has important effects on collecting duct acid-base transport that are mediated partly by nitric oxide. Dietary protein intake stimulates urinary acidification through a process involving H + -ATPase activation, mediated by endothelin and nitric oxide. Endothelin-1 (ET-1) is synthesized by the collecting duct, and endothelin receptors ET-A and ET-B are present in the collecting duct. ET-B activation regulates both type A and type B intercalated cell responses to metabolic acidosis.
The calcium-sensing receptor (CaSR) is apical in inner medullary collecting duct cells and in type A intercalated cells and mediates luminal Ca 2+ -stimulation of H + -ATPase. Luminal acidification stimulated by this pathway may inhibit calcium precipitation and minimize development of nephrolithiasis.
Activation of the vasopressin type 1A (V1a) receptor is an additional regulatory mechanism. The V1a receptor is expressed in the medullary TAL (MTAL) and throughout the collecting duct, with expression in both intercalated cells and principal cells in the CCD and only in intercalated cells in the OMCD. Metabolic acidosis increases V1a receptor expression in the MTAL and the OMCD in the inner stripe. Genetic deficiency of the V1a receptor causes development of type IV RTA and diminishes mineralocorticoid stimulation of H + -K + -ATPase and Rhcg.
Several other hormones and drugs also alter collecting duct acid-base transport. Kallikrein inhibits bicarbonate secretion. Calcitonin stimulates H + -ATPase–dependent bicarbonate reabsorption in the rabbit CCD. Isoproterenol stimulates bicarbonate secretion by type B intercalated cells.
Paracrine Regulation
Several compounds produced and/or transported in the proximal tubule and TAL have downstream effects that regulate collecting duct acid-base transport. Presumably, this arrangement enables these segments, which exist in an area with very high blood flow and thus rapid exposure to changes in systemic acid-base and potassium, to regulate transport in collecting duct segments in the outer medulla and inner medulla, sites of low blood flow and thus reduced exposure to changes in systemic acid-base and potassium homeostasis. The paracrine molecules most extensively studied are ammonia and α-ketoglutarate (α-KG).
Ammonia, discussed in detail later regarding its role in net acid excretion, is produced in the proximal tubule and undergoes regulated transport in both the proximal tubule and the TAL in response to both acid loading and hypokalemia. In addition to its roles in bicarbonate generation, ammonia stimulates CCD bicarbonate reabsorption in a concentration-dependent fashion. Ammonia stimulates both type A intercalated cell acid secretion and inhibits type B intercalated cell bicarbonate secretion. Its stimulation of proton secretion involves stimulation of H + -K + -ATPase, not H + -ATPase.
The Krebs cycle intermediate, 2-oxoglutarate (α-KG), may have an important role in acid-base homeostasis. Changes in acid-base loading change the net direction of transport in the proximal tubule and the loop of Henle from reabsorption (acid-loaded) to net secretion (alkali loading). In the CNT and CCD, luminal 2-oxoglutarate regulates net bicarbonate and sodium chloride reabsorption, acting through its receptor, Oxgr1, in type B and non-A, non-B intercalated cells. Thus, 2-oxoglutarate can function as a paracrine mediator, enabling functional coordination of the proximal tubule and the TAL with the collecting duct.
Cellular Adaptations to Acid-Base Perturbations
In addition to changes in the abundance and subcellular distribution of membrane transporters, adaptive responses to some physiologic disturbances may involve changes in the numbers of intercalated cells. Several studies have shown that chronic metabolic acidosis and chronic hypokalemia increase intercalated cell numbers in medullary collecting ducts, whereas others have found no change in intercalated cell number in these conditions. Long-term administration of lithium or acetazolamide also increases intercalated cell numbers in the OMCD.
Increases in intercalated cell numbers could result from intercalated cell proliferation or from principal cell proliferation followed by conversion into intercalated cells. Studies using proliferation markers show that metabolic acidosis, hypokalemia, and lithium administration are each associated with increased proliferation of collecting duct cells, some showing increased proliferation in type A intercalated cells and others showing the proliferating cells to be principal cells. The latter studies suggest that principal cells and OMCD intercalated cells may interconvert on the basis of observations of rare cells with immunohistochemical and ultrastructural characteristics of both cell types.
With respect to the CCD, some studies have suggested there may be interconversion of type A and type B intercalated cells. An early paper examining the rabbit isolated perfused CCD equated apical endocytosis with alpha (type A) intercalated cells and used apical peanut lectin binding as a marker of beta (type B) intercalated cells. Long-term NH 4 Cl loading in vivo increased the number of intercalated cells exhibiting apical endocytosis in microperfused CCDs and decreased the number of cells that bound peanut lectin; the interpretation was that intercalated cell subtypes in the CCD could interconvert, with the type B intercalated cells reversing polarity to meet the physiologic demand for increased acid secretion. Subsequently, some studies reported that acidosis, lithium administration, and carbonic anhydrase inhibition alter the relative numbers of intercalated cells identifiable as type A or type B, although none have shown cells in native tissue with either apical AE1, basolateral pendrin, or coexpression of these two transporters. Other studies of acid-base disturbances find regulation of the abundance and distribution of transport proteins specific to the A and B intercalated cell types and changes in cell morphology, but no change in the relative or absolute numbers of specific intercalated cell subtypes. The explanation for these different findings could include differences in the experimental models, species examined, sensitivity and specificity of intercalated cell identification, and cell quantitation methods.
In vitro studies have implicated the extracellular matrix protein hensin and the prolyl isomerase activity of cyclophilin in the process of intercalated cell remodeling. In mice with intercalated cell–specific hensin deletion there is development of dRTA, lack of type A intercalated cells, and an increased number of type B intercalated cells. Hensin’s effects on type A intercalated cell development appears to require the activation of β 1 integrin.
Bicarbonate Generation
Acid-base homeostasis requires not only reabsorption of filtered bicarbonate but also the generation of new bicarbonate to replace the bicarbonate used for buffering of endogenous and exogenous fixed acids. There are two major components of bicarbonate generation, titratable acid excretion and ammonia excretion. In addition, organic anion excretion is biologically important. Organic anions can be metabolized to form HCO 3 − ; accordingly their excretion is physiologically equivalent to bicarbonate excretion.
Titratable Acid Excretion
Titratable acids are urinary solutes that buffer secreted protons, enabling H + excretion without substantial changes in urine pH. Titratable acid excretion constitutes about 40% of net acid excretion under basal conditions. Metabolic acidosis increases titratable acid excretion by as much as 50% above baseline ( Figure 9.5 ).

Multiple buffers contribute to titratable acid excretion. An ideal urinary buffer has a pK a lower than systemic pH, so that the majority of the filtered component is in the base form, and a pK a higher than urine pH, so that the majority of the urinary component is in the acid form. Phosphate is the predominant titratable acid and typically accounts for more than 50% of total titratable acid. Citrate and creatinine also contribute to titratable acid excretion, but to a lesser extent. Ammonia is frequently termed a urinary buffer, but because of its high pK a it does not contribute substantially to titratable acid excretion. The role of ammonia in new bicarbonate generation is considered separately later. Figure 9.6 shows both the relative contributions of major urinary buffers to titratable acid excretion and the effect of changes in urine pH after the amount excreted under normal conditions and the pK a of each buffer is taken into account.

Phosphate as a Titratable Acid
Titratable acid excretion in the form of phosphate is the amount of HPO 4 2− that is filtered, not reabsorbed, and that buffers secreted H + , forming H 2 PO 4 − . Phosphate exists, under physiologically relevant conditions, in equilibrium between two forms, H 2 PO 4 − and HPO 4 2− . The relative amount of these two forms is given by the following formula:
10 pH − 6.8 = [ HPO 4 2 − ] [ H 2 PO 4 − ]
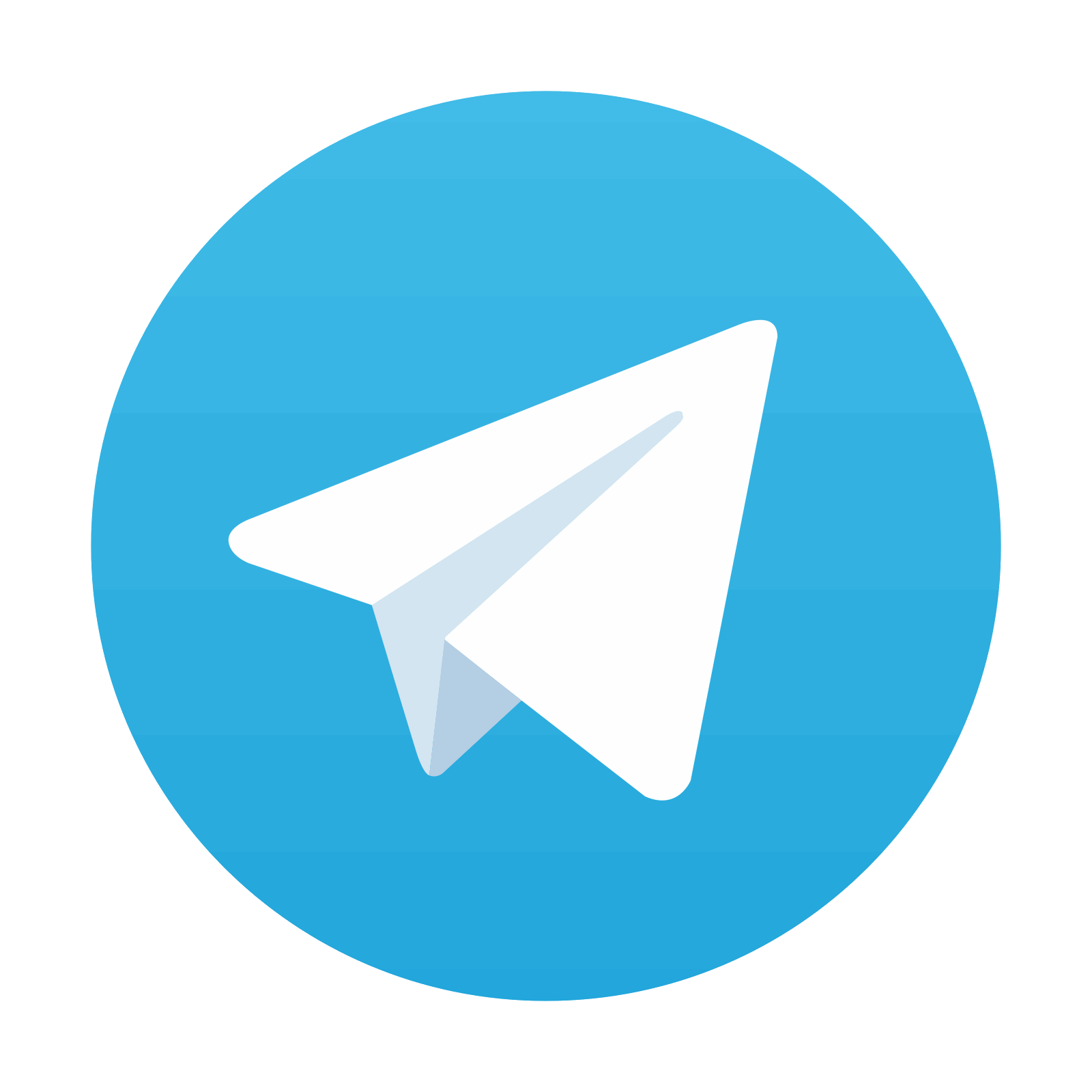
Stay updated, free articles. Join our Telegram channel

Full access? Get Clinical Tree
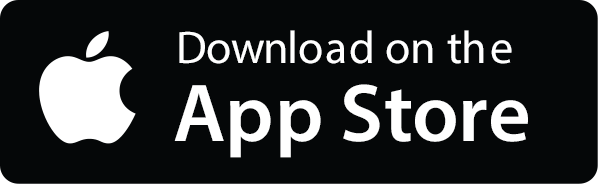
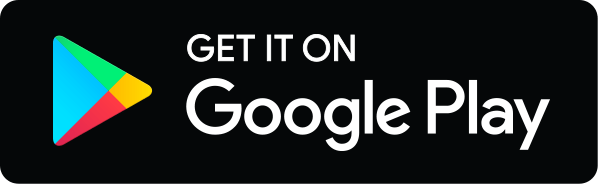