25 Adam Cox1, Matthew Jefferies1,2,3, and Raj Persad4 1 Department of Urology, University Hospital of Wales, Cardiff, UK 2 European Cancer Stem Cell Research Institute, Cardiff University, Cardiff, UK 3 Institute of Cancer & Genetics, Cardiff University School of Medicine, Cardiff, UK 4 Bristol Urological Institute, Southmead Hospital, Bristol, UK The prostate gland is a largely androgen‐sensitive exocrine gland of the male mammalian reproductive system. A sound understanding of its structure and function will equip the urologist with the foundation on which to build experience in open, endoscopic, laparoscopic, and robotic‐assisted laparoscopic prostate surgery, all of which are relevant in the emergency and elective setting alike. The following chapter focuses on the embryology, anatomy, physiology, and histology of the normal human prostate. Keywords: prostate; prostate development; prostate anatomy; prostate physiology; prostate capsule Our closest mammalian kin are the primates. Here we find variations on a common underlying theme (Figure 25.1). There are two distinct ‘prostates’ – cranial and caudal – shaped like croissants rather than doughnuts. There is a gap between them for the vasa deferentia and common ejaculatory ducts to enter the urethra on either side of the verumontanum which also contains the vestige of the fused müllerian ducts, the utriculus masculinus. Figure 25.1 The fundamental ‘blueprint’ of the primate prostate. There are two distinct prostates, cranial and caudal between which pass the ejaculatory ducts. Note also the two distinct parts to the external sphincter. Just downstream of the caudal prostate is the external sphincter; it is made of two muscular components – unstriated and striated – which are both cephalad and distinct from the levator ani sheet of the pelvic floor. In man, the cranial prostate fits into the caudal prostate like an egg in an egg‐cup (Figure 25.2); both prostates are deficient in front where glandular tissue is replaced by a fibromuscular septum (Figure 25.3). Figure 25.2 The cranial prostate is fitted into the caudal prostate like an egg in an egg cup. Figure 25.3 The gap in the front of the prostate in man is filled by a fibromuscular septum. The normal pattern of growth and development of the prostate gland depends on testosterone. To become activated, testosterone must be converted to dihydrotestosterone by the enzyme 5‐alpha‐reductase within the prostatic cells. In early foetal life, the mesenchyme of the prostate differentiates into fibrous tissue and muscle, but there are few glandular elements. During the postnatal period and under the influence of androgens, glandular elements bud out to form ducts from the posterior wall of the urethra on either side of the verumontanum, and displace the urethra forwards (Figure 25.4). Ducts form true patent lumens, and the epithelial cells lining the acini fully differentiate to synthesise a variety of secretory products essential for sperm survival. During puberty, serum testosterone increases to induce epithelial proliferation and the formation of a mature adult prostate, resulting in elongation of the urethra (Figure 25.5) [1, 2]. Figure 25.4 The prostatic ducts grow (a) back, out, and round from either side of the verumontanum, and (b) displace the urethra forwards. Figure 25.5 Diagram of structure of normal adult prostate. There is a stroma made of smooth muscle and fibrous tissue, into which run the prostatic ducts and acini. At around the age of 40 years, small nodules of hyperplastic acini and muscle begin to appear in the transitional zone of the gland (Figure 25.6). These nodules grow and coalesce to become the common pathological entity benign prostatic hyperplasia (BPH). Figure 25.6 (a) Around the age of 40 nodules of hyperplastic acini and muscle begin to appear in the inner zone which may (b) form a ring of muscle or (c) a bulky mass of ‘adenoma’. The size of the ‘normal’ prostate is difficult to quantify because the prostate volume increases with age under the effect of androgens. In the young adult male, the prostate is about the size of a chestnut, weighing 10–15 g, and by age 50 years its size could be likened to that of a ping pong ball (approximately 30 g). The urethra bends slightly (by about 30°) as it passes through the gland (Figure 25.7). The prostate is thin in front (the fibromuscular septum) where it is devoid of acini. The openings of the prostatic ducts are found along the posterior half of the prostatic urethra, and the glands curve round on either side. There is virtually no glandular tissue in the midline anteriorly. Figure 25.7 Sagittal section through the prostate showing anatomical relations. Most of the normal adult gland lies behind the urethra. It is separated into an inner cranial and an outer caudal zone by the entry of the ejaculatory ducts. The innermost part of the inner cranial part is the paraurethral zone. Cranial to the prostate within the true bony pelvis are the trigone, ureters, and base of the bladder, and behind lie the seminal vesicles and vasa deferentia. The two layers of peritoneum that form the fascia of Denonvilliers separate the prostate and vesicles from the rectum behind. The deep veins of the penis and the symphysis pubis are located anterior to the gland. Posterolateral to the prostate are the neurovascular bundles which supply the corpora cavernosa of the penis (Figure 25.8) [3]. The inferior‐most aspect of the prostate, the apex, is directed downward and is intimately related to the superior part of the urogenital diaphragm. Figure 25.8 Diagrammatic cross‐sections through the prostate at two levels showing the situation of the neurovascular bundles to the penis. The adult prostate consists of fused zones that are anatomically distinct, have characteristic histological features, and importantly, have typical predisposition to benign or malignant change [4]. These are the anterior fibromuscular stroma, which is devoid of glandular components, the periurethral transitional zone (TZ), which comprises of about 5–10% of the glandular tissue, the central zone (CZ; 20–25%), and the peripheral zone (PZ; 70%) (Figure 25.9) [5, 6]. The central zone surrounds the ejaculatory ducts and comprises the portion of the prostate that extends from the base proximally to where the ejaculatory ducts enter the urethra, near the prostatic utricle at the verumontanum distally. The TZ is located interiorly between the urethra and the surrounding peripheral and CZs, while the PZ is found on the posterolateral aspects of the prostate. The main morphological differences between the TZ and PZ are few; however they are easily identifiable pathologically because the TZ is chiefly affected by BPH. Figure 25.9 Anatomy of the normal human prostate. (a) Sagittal section through the prostate demonstrating the orientation of the base (most cranial aspect) and the apex (most caudal aspect). Source: Adapted from McNeal [7] in Valkenburg and Williams [8] (b) Axial section through the base, illustrating its intimate relation to the pubis (anteriorly) and rectum (posteriorly). Source: Adapted from Walz, Burnett, Costello, et al. [9]. (c) A haemotoxylin and eosin (H&E) section through the base of a prostate removed following an open radical retropubic prostatectomy for localised prostate cancer. The impressive demarcation delineating the transition (T) and peripheral (P) zones are due the patient’s young age. In older men, these planes would be more indistinct. A, anterior fibromuscular stroma; ED, ejaculatory duct; U, urethra. H&E slides courtesy of Dr. David Griffiths, consultant histopathologist, UHW, Cardiff. Although lobes are recognisable in the developing human prostate, the lobar classification is still employed by urologists, particularly when describing the prostate at transurethral resection (TUR; Table 25.1). Table 25.1 Each prostate lobe corresponds to part(s) of an anatomical zone. The terminology relating to prostate lobar anatomy is commonly used for surgical description. Prostate lobes do not precisely correspond to individual prostate zones; indeed the lateral lobes (readily visible during TUR) span all zones. There are three separate entities that share the term ‘prostate’, with potential to confuse the urologist. The prostate is surrounded by a capsule that is ill‐defined in an anatomical sense. It consists of concentrically layered fibromuscular fascicles that are inseparable from the prostatic stroma anteriorly [10–12]. Vessels and nerves penetrate the capsule laterally [11, 12]. The prostate capsule is discontinuous: it is non‐existent at both the apex and the base of the prostate [11]. At its apex, the prostate stroma merges with the muscle fibres of the urinary sphincter and at the base with the smooth muscle fibres of the detrusor [11, 13]. In the early days of prostatectomy after the adenoma was enucleated from its shell with the finger, at first it was thought that the entire prostate had been removed from within its anatomical capsule [14]. Before long the error was recognised, and it became clear that the shell left behind was a layer of compressed prostatic tissue, often containing adenomatous nodules [15]. This technique was subsequently adopted by surgeons such as Millin [16, 17] to enucleate adenomas causing symptoms in BPH, prior to the advent of TUR. TUR later became, and continues to be, the gold standard surgical procedure for BPH. To this day, ‘simple’ open prostatectomy (which can be anything but!) remains an important technique for large, vascular prostates causing bothersome symptoms or haematuria. Endoscopically, this capsule is the limit of enucleation to the urologist that practices holmium laser enucleation of the prostate (HoLEP). This is the term given to the lacy veil of tissue recognisable at TUR. The skill of TUR rests on being able to remove all the adenoma without perforating this ‘capsule’, outside which lies fat and veins. The layer of tissue is in fact thinner than the wire of the resectoscope loop. Capsular perforations at TUR are identifiable by persistent bubbles adherent to the fat outside the capsule, whereas bleeding from peri‐prostatic veins is not so difficult to identify. They can cause bleeding which is not seen when the bladder is full but brisk when the bladder is empty. This should be managed with careful diathermy in the first instance, with or without a large catheter balloon (up to 30–40 ml) or intermittent catheter traction. If refractory or heavy bleeding ensues then open packing of the prostate is occasionally required [18]. The prostate has a rich blood supply entering the gland in two main leashes on either side from the inferior vesical branches of the anterior branch of the internal iliac (hypogastric) arteries. The prostate artery then divides into the urethral and capsular arteries. From the urethral group arise Flock’s and Badenoch’s arteries, both of which supply the TZ. Flock’s arteries approach the bladder neck at 1 and 11 o’clock and Badenoch’s approach it is at 5 and 7 o’clock (Figure 25.10). Awareness of these arteries is useful when performing a transurethral resection of the prostate (TURP) to help prevent bleeding. The capsular branches of the prostatic artery run with the cavernosal nerves [19, 20]. In addition to the prostatic arteries, the prostate is supplies by a small branch of the middle rectal artery. Figure 25.10 (a) The main arterial supply to the prostate from the inferior vesical and middle rectal arteries. (b) At transurethral resection of prostate (TURP) the main vessels are to be expected at 11, 1, 5, and 7 o’clock. Initially, the dorsal vein of the penis passes beneath the symphysis pubis emerging in between the puboprostatic ligaments. The dorsal vein then trifurcates into a central superficial branch that runs in the midline over the prostate and two lateral plexuses that runs along the side of the prostate running within the pelvic fascia. During a radical retropubic prostatectomy, after division of the puboprostatic ligament, to avoid blood loss the dorsal vein should be divided distally, before it trifurcates. The superficial branch drains the anterior prostate. The lateral plexuses drain the remaining prostate as well as the rectum and communicate with numerous vesical veins prior to draining into the internal iliac (hypogastric) vein. In the pelvis, numerous venous communications exist via valveless veins between the prostatic plexus and veins draining the pelvic bones and the vertebral plexus, affording an easy route for spread of cancer [21]. The lymphatic drainage of the prostate primarily drains to the obturator and the internal iliac lymphatic chains that travel with their accompanying vessels; they also communicate directly with the bone marrow of the vertebrae, pelvis, and femora. This explains why bone metastasis to these areas is more common. There is also lymphatic communication with the external iliac, presacral, and the para‐aortic lymph nodes. The autonomic innervations of the prostate arise from the pelvic plexus formed by the parasympathetic fibres that arise from sacral levels (S2–S4) and sympathetic fibres from lumbar levels (L1–2). The parasympathetic and sympathetic fibres travel to the prostate via the cavernous nerves, running postero‐lateral within then pierce the thin capsule along with the fine branches of the arteries. The parasympathetic nerves end on the acini leading to increased prostatic secretion during sexual arousal. The sympathetic nerves end on α‐1 receptors on smooth muscle of the capsule which are stimulated during ejaculation and closing the preprostatic sphincter and relax during voiding. Nerve supply to the prostate is involved in glandular secretion and emission, with no active role in continence. The adrenergic fibres end in the stromal tissue (98%), being mainly α1 (90–60% are α1a) and α2 (10%). Cholinergic fibres end in the epithelium and help regulate secretions. The pudendal nerve is the major nerve supply, leading to somatic innervations of the striated sphincter and the levator ani. The function of the prostate gland is often overlooked by its association with benign enlargement and malignancy. Its main function, however, is the production of an acidic secretion (pH ~6) containing zinc, citrate, and polyamines, in addition to strong proteolytic enzymes such as prostate‐specific antigen (PSA) and prostatic acid phosphatase (PAP) which liquefy the seminal coagulum. PSA is an androgen‐regulated 34 kDa glycoprotein enzyme belonging to the kallikrein family of serine proteases. It is produced primarily by prostate ductal and acinar epithelium and is secreted into the lumen, where its function is to cleave semenogelin I and II in the seminal coagulum [22]. As the coagulum dissolves, the sperm simultaneously become highly motile, facilitating its journey from the vagina to the ova. Nearly 75% of PSA is bound to plasma proteins (i.e. α‐1 anti‐chymotrypsin and α‐2 macroglobulin) and metabolised in the liver with the remaining 25% free and is excreted in the urine. Its major relevance in oncology is as a biomarker to detect prostate cancer (PCa) and to assess responses to treatment. With PCa, there is less PSA production; however, a characteristic of early PCa is disruption of the basal cell layer and basement membrane, causing loss of normal glandular architecture and allowing PSA direct access to the peripheral circulation as oppose to the lumen, giving rise to a significantly higher serum level count [23, 24]. It is measured by radioimmunoassay in the serum and identified by immunohistochemistry of histological section, thus clearly identifying metastases that have risen from the prostate. Serum PSA’s half‐life is nearly two. Two days and levels vary with age (Table 25.2) and race [25]. Table 25.2 Age‐specific reference range guide. PSA, prostate‐specific antigen. One must remember that the PSA is prostate specific and not PCa specific, with many factors causing PSA levels to read high. More common factors include, BPH, urinary retention, catheterisation, prostatic biopsies or TURP, and UTIs, especially prostatitis. PAP is a prostate epithelium‐specific secretory protein that is found in large amounts in the seminal fluid. PAP was the first tumour marker used for prostate cancer [26] and used in the pioneering work by Huggins and Hodges, demonstrating the beneficial effects of castration on PCa bone metastases [27]. Elevated PAP levels have been reported in 70% of metastatic disease [28]; however, it is reported to be elevated in only 1% of patients with localised disease [29] and may be significantly artefactually elevated after digital rectal examination (DRE). During sexual arousal, spermatozoa are transported from the ampulla of the vasa into the prostatic urethra, this is called ‘emission’. The sperm mixes with fluid from the prostate, seminal vesicles, and bulbar‐urethral (Cowper) glands to form semen. During ejaculation, the smooth muscle of the prostate capsule contracts synchronously with the bulbospongiosus muscle, along with the pelvic floor muscles and the ischocavernosus muscles. The rhythmic contractions propel the semen distally through the urethra. During erection, the bladder neck (preprostatic sphincter) closes (under sympathetic stimulation) and prevents retrograde flow of semen into the bladder; bladder neck closure is more marked just before ejaculation. Hence why it is difficult to urinate with an erection or after ejaculating. If the bladder neck has been cut, the sympathetic nerves have been divided or the α‐1 drive to the bladder neck has been blocked by medication, then semen will leak back into the bladder (retrograde ejaculation) producing a more or less ‘dry ejaculation’. The fructose‐rich seminal fluid provides the nourishment for the sperm and helps to wash the sperm through the ejaculatory duct and urethra. The seminal secretions contribute to approximately two‐thirds of the semen volume. The bulbar‐urethral glands produce a pre‐ejaculate that lubricates the urethra to facilitate sperm passage. At the neck of the bladder there is a ring of α‐1 adrenergic smooth muscle intertwined with prostatic acini and fibrous tissue (Figure 25.11). In middle age, this ring of muscle, often referred to as the bladder neck, although distorted by adenoma, remains under the influence of α‐1 agonists. Stimulation of the presacral nerve causes it to contract, whereas α‐1 blockers cause it to relax. Figure 25.11 The three components of the sphincter of the prostate. The bladder neck is made up of alpha‐adrenergic smooth muscle mixed with prostatic acini and some fibrous tissue. Caudal to the verumontanum, there is a second sphincter, the external sphincter, and hence why the ‘veru’ is a key landmark in TUR (i.e. to prevent resection distally risking damage to the sphincter). It is contained in the few millimetres of tissue immediately surrounding the urethra and is quite distinct from the striated pubococcygeal part of the levator ani. During operations on the bulbar urethra (e.g. resection for cancer or repair of a ruptured posterior urethra), this intramural external sphincter can be clearly seen above and moving independently from the hiatus in the levator ani sheet (Figure 25.12). Figure 25.12 At urethrectomy or urethroplasty the ring of the levator ani can be seen to be quite distinct from the supramembranous sphincter which is part of the wall of the membranous urethra – hence ‘intramural sphincter’. The external sphincter has two components: (i) an inner sleeve of smooth muscle which is continuous with that of the prostate (and thought to be supplied by α‐1 adrenergic nerves) and (ii) an outer layer of striated muscle (Figure 25.13), a special striated muscle made up of small cells, classified as the slow‐twitch type and can maintain contraction for long periods. Electron microscopy shows these small slow‐twitch fibres to have a high content of lipid and mitochondria which make them able to maintain contraction for a long period. Figure 25.13 Schematic view of the prostate showing the situation of the supramembranous intramural sphincter. Every urologist should appreciate how small and vulnerable is this precious sleeve of muscle on which male continence depends after operations on the bladder neck or prostate. At the beginning of normal micturition the bladder neck relaxes, and at the same time, the detrusor contracts. When the bladder is empty, the external sphincter closes first and the prostatic urethra is emptied by milking back the small quantity of urine it contains into the bladder and then the bladder neck closes (Figure 25.14). Figure 25.14 (a) During micturition all the sphincters are open. (b) On completion of voiding the rhabdosphincter closes. (c) Then the intramural sphincter closes and the prostatic urethra milks back the urine. (d) Finally, the bladder neck closes and the external sphincter relaxes. Both components of the intramural sphincter are innervated by the pelvic splanchnic nerves.
Prostate Structure and Function
Abstract
25.1 Comparative Anatomy
25.2 Maturation of the Prostate
25.3 Anatomy of the Prostate
25.3.1 Topographical Anatomy
Prostate lobe
Corresponding prostate zone
Anterior
Mainly transitional zone
Median
Central and transitional zones
Lateral
Spans all zones
Posterior
Predominantly the peripheral zone
25.3.2 Capsule of the Prostate
25.3.2.1 Anatomical Capsule
25.3.2.2 Capsule at ‘Simple’ Open Prostatectomy for Benign Prostate Hyperplasia (Millin Prostatectomy)
25.3.2.3 Capsule at Transurethral Resection
25.3.3 Arteries
25.3.4 Veins
25.3.5 Lymphatics
25.3.6 Nerves
25.4 Prostate Physiology
25.4.1 Function
25.4.2 Prostate‐Specific Antigen
Age (years)
PSA (ng ml−1)
40–49
2.5
50–59
3.5
60–69
4.5
70–79
6.5
25.4.3 Prostatic Acid Phosphatase
25.4.4 Emission and Ejaculation
25.4.5 Prostate and Sphincters
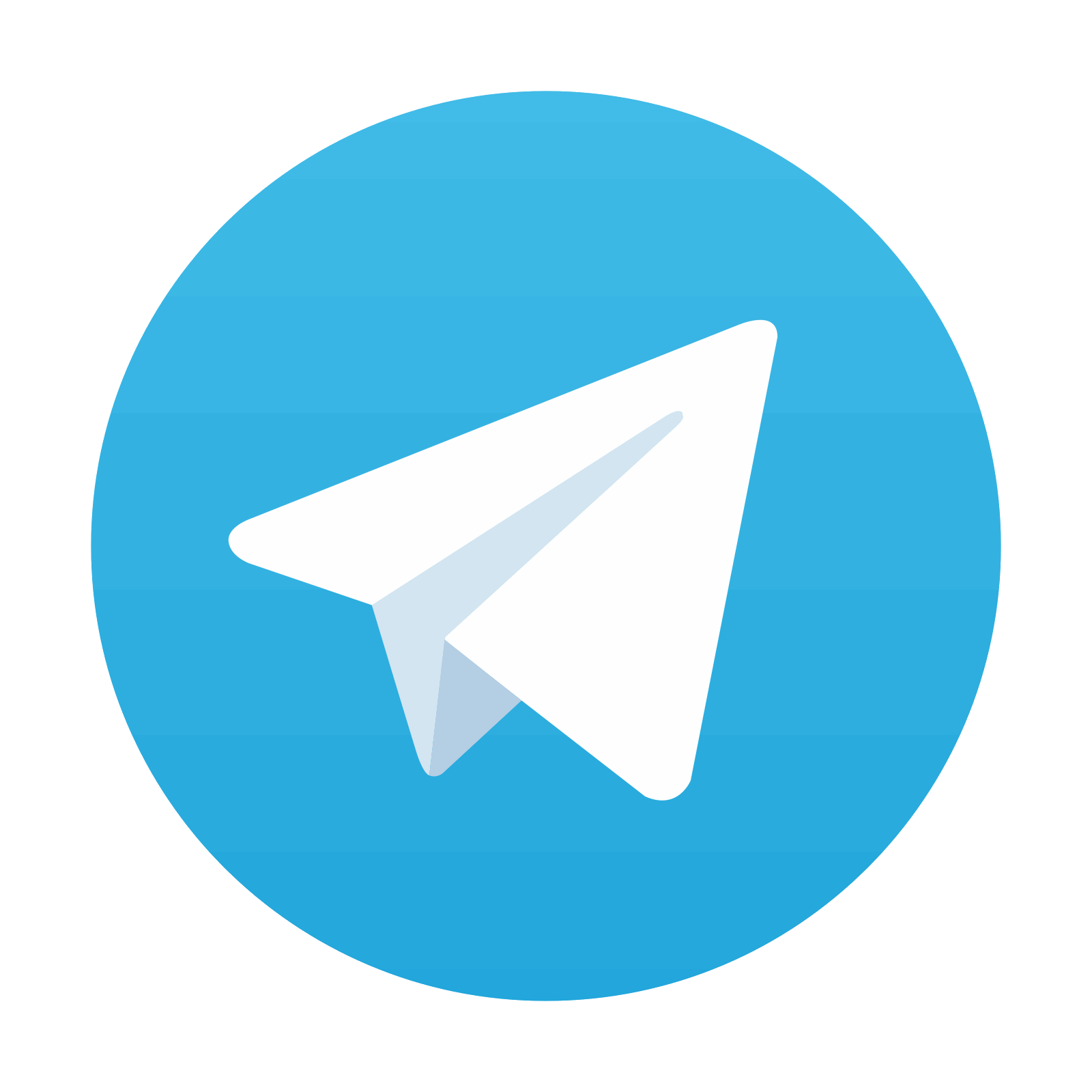
Stay updated, free articles. Join our Telegram channel

Full access? Get Clinical Tree
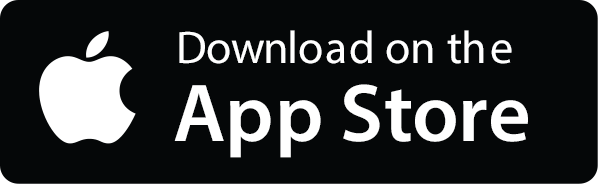
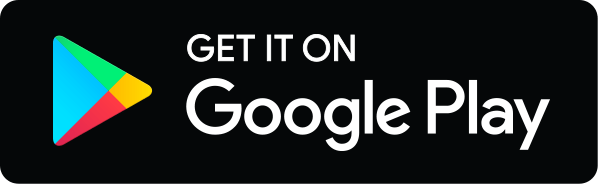