1.1
Principles of Urological Technology
Shibs Datta Motaz
University Hospital of Wales, Cardiff, UK
Abstract
Urology and technology have always been intricately related. Our specialty has been a pioneer of minimally invasive diagnostic and therapeutic approaches which have evolved in complexity and functionality. We started using endoscopes, then innovated with ample use of lasers, and have now introduced the widespread use of robotic assisted surgery. Urologists are challenged to be familiarised with a diversity of devices, which range from flexible, rigid, and semi‐rigid endoscopes to a number of lasers with different wavelengths and capabilities.
Keywords: endoscopy; laparoscopy; laser; catheters; stents; guide wires
1.1.1 Optics in Urology
Historically, urologists have been defined by their endoscopic skills. This continues to be the case, with advances in optical and camera technology ensuring that urologists remain at the forefront of surgical innovation.
1.1.1.1 The Rod‐Lens System
The first cystoscope was introduced by Maximilian Nitze in 1876. Modifications through the first half of the twentieth century changed the device so that it was lit by a heated platinum wire at its distal end [1]. The wire was soon replaced by Edison’s electric lamp. The telescope, originally made as an integral part of the instrument, was later constructed so to be removable from a separate sheath through which the instrument could be irrigated. To transmit the image, the telescope had glass lenses and prisms separated by spaces of air (Figure 1.1.1). Limitations of this design include the technical difficulties in engineering small lenses that are of high enough quality to avoid image degradation and peripheral aberration. Metal mounts required for such traditional designs would also reduce light transmission through the telescope.

Figure 1.1.1 Diagram of conventional cystoscope. The glass lenses are held in place by metal spacers and separated by air spaces.
Late in the 1950s, Professor Harold Hopkins was approached by James Gow, a renowned urologist from Liverpool, with the aim of improving this system. The rod‐lens telescope reverses the traditional glass‐lens system, such that the telescope is, in essence, a rod of glass with air gaps within that act as the lenses (Figure 1.1.2).

Figure 1.1.2 Rod‐lens telescope, with ‘lenses’ of air, separated by ‘spaces’ of glass, with no need for metal spacers.
Source: Courtesy of Professor H. H. Hopkins.
Changing the main transmission medium from air to glass doubled the light which could pass through a system of given diameter. A second doubling of light transmission resulted from the omission of the metal spacers, which were no longer needed to position the lenses; the rods could be mounted without them [2].
Furthermore, the refractive surfaces of the rods were technically easier to precisely engineer so that they could be manufactured to a high degree of optical accuracy. Hopkins complemented these improvements by making use of modern glasses, sophisticated computer‐aided design, and multilayer lens blooming. The latter minimised internal reflections within the system, improved light transmission, and suppressed stray images [3].
The result of these improvements has been a family of telescopes, each with good optical resolution through an angle of field of 70° or more, with the image quality of a microscope. The angle of view can be varied by incorporating a prism behind the objective lens. The traditional 30° telescope is a throwback to the days of distal illumination and is widely used, not only for cystoscopy, but laparoscopy as well (Figure 1.1.3). Rotating an angulated cystoscope around its axis during endoscopy therefore enables optimal visualisation of a spheroid structure, such as the bladder.

Figure 1.1.3 (a) Comparison between the angle of view offered by the convetional 30° ‘fore‐oblique’ optic and the forward viewing 0° telescope. (b) The tetroviewing 120° telescope allows the bladder neck and anterior bladder wall to be seen clearly.
The rod‐lens element of the telescope enables image transmission, but illumination is mediated by a separate system incorporated into the telescope design. Parallel to the rod‐lens element is a noncoherent fibre‐optic bundle (see below). This is not used for image transmission, but it transmits light from an external source (such as a 3000 W xenon‐arc lamp) to provide the illumination required.
Problems related to the rod‐lens telescope are relatively infrequent, given the precision engineering required in their manufacture and the workload required of them.
Reduced image contrast can occur as a result of a breach of fluid into the telescope system, resulting in internal condensation. In these circumstances, the telescope must be immediately sent away for repair or replacement because of the risk of contamination to a patient.
Poor light transmission may be the result of damage to the fibre‐optic bundles within the telescope itself that transmit the external light source from the external cable connection to the distal end of the telescope. Sometimes, poor illumination is because of the fibre‐optic cable that transmits light from the external light source to the telescope; this can be checked by qualitatively comparing the illumination coming directly from the external cable with the illumination from the telescope itself with light cable attached.
Blurring of the image or loss of some of the image field can be as a result of accretions accumulating on the proximal or distal end of the telescope. Chipping of the rod lens can result in partial blurring when the damage is nearer the eyepiece, but cracking or chipping can sometimes be seen more discretely with more distal telescope damage.
1.1.1.2 Fibre‐optic Flexible Endoscopes
Unlike rigid endoscopes which utilise rod‐lens technology, flexible endoscopes all use fibre‐optic image transmission. The basis of fibre‐optics is the passage of light along glass fibres by a process of total internal reflection, hence, why endoscopes can maintain adequate lighting and imagery (Figure 1.1.4). J. L. Baird [4] conceived the idea of using an array of fibres to transmit an image, but it was Hopkins who constructed the first working fibrescope [5].

Figure 1.1.4 (a) A flexible cystoscope looking back at itself the ‘J manoeuvre’. (b) Total internal reflection permits light to travel along a flexible glass fibre.
Each glass fibre consists of a light‐transmitting translucent core surrounded by glass cladding with a lower refractive index. Light is transmitted through the fibre via a process called ‘total internal reflection’, with reflection occurring at the interface between the two transparent materials. As there may be up to 10 4 internal reflections over a 1‐m length of fibres, even a small light loss at each one would cause a considerable fall‐off in the efficiency of light transmission were it not for the cladding present. The cladding also protects the surface of the fibre core from damage and contamination which would otherwise interfere with internal reflection. Light losses of 50% or less over a 1‐m light path can be achieved.
The glass fibre core and cladding is arranged in bundles that may be coherent or non‐coherent. Coherent bundles are arranged such that the proximal position of each fibre with respect to the entry face maps precisely to the corresponding position of the fibre distally on the exit face of the flexible instrument. This enables light focusing on the distal face of the endoscope to be transmitted precisely to create an identical image at the proximal face of the endoscope.
Noncoherent fibre bundles are such that the fibres are arranged randomly, enabling light but not image transmission. In urology, flexible cystoscopes, semi‐rigid ureteroscopes, and flexible ureteroscopes all utilise coherent fibre‐optic technology, whereas light‐transmission cables are incoherent fibre bundles. The individual fibres within endoscopes are typically smaller than for light‐transmission cables, but the principles underlying both coherent and noncoherent fibre bundles are essentially identical.
1.1.2 Surgical Energy
1.1.2.1 Diathermy
Surgical diathermy utilises the principle that as a current passes through a resistor, heat is dissipated. This results in a temperature rise, and from a surgical perspective, controlled tissue cutting and coagulation [6].
An alternating current from the domestic mains causes such tissue heating, but at 50 Hz, it can also cause electrocution and ventricular arrhythmias, resulting in a potentially fatal outcome.
The underlying principle of safe diathermy (amongst other factors) is not a function necessarily of high or low voltage or current, but of the kHz range frequency at which neurophysiological conduction and axon depolarisation seem to be refractory. A low‐frequency current as small as 1 mA can induce fatal cardiac arrhythmias, but at radiofrequencies (500–5000 kHz), neurophysiological conduction does not occur, and currents as high as 2 A can therefore be used for surgical diathermy.
Surgical diathermy exploits the heat generated when an alternating current passes through a conductor. When there is a large density of electrical current passing through tissue, the temperature rise can be enough to give a useful surgical effect. In monopolar diathermy, the surgeon uses a small active electrode to give a high current density and a large heating effect at the operative site at a frequency of about 200 kHz. Because the current density near the large return electrode, which completes the circuit, is small, it produces little heat. In bipolar diathermy the thermal effect occurs in tissue held between two small active electrodes and does not pass through the patient’s body which is not being treated. Bipolar frequency is between 250 kHz and 1 MHz.
Nerves and muscles are stimulated by alternating current of low frequency (faradism), but this faradic effect does not occur when the frequency exceeds a certain value as neurophysiological conduction becomes refractory as such frequencies. Surgical diathermy is used for both cutting and coagulation. A pure cutting current utilises an alternating current which is constant; the root mean square of such a waveform enables energy to be delivered at a sufficient level to vaporise intracellular water with cell destruction, achieving very high current densities. For electro‐coagulation, the diathermy waveform consists of bursts of alternating current between periods of rest (all still occurring at high frequency), resulting in protein denaturation (and hence thermo‐coagulation), without vaporisation. The dead tissue is shrunken and desiccated in situ – distortion of the walls of blood vessels, coagulation of plasma proteins, and stimulation of the clotting mechanism all act to check bleeding. Ideally, intracellular temperatures do not reach 100 °C so there should be no unwanted cutting.
1.1.2.2 Contact Diathermy
In contact diathermy, the main impedance (resistance) to current flow is at the interface between the electrode and tissue, where it is influenced by the type of tissue and its state of hydration. The impedance of fat is high compared to muscle, and contact diathermy works less efficiently on adipose tissue. As diathermy proceeds, the tissue in contact with the electrode dessicates and electrical impedance rises. Eventually, the current flow is insufficient to produce further heating and the surgical effect ceases. This limits the depth of penetration of diathermy applied to one spot. The effect of contact diathermy also depends on the size and shape of the active electrode. A ball electrode with a large surface area held in contact with tissue will tend to apply current at a relatively low density, giving a coagulating effect, but the depth of tissue coagulated is proportional to the square of the diameter of the ball. Contact cutting by point diathermy is mainly by physical disruption of tissue softened by coagulation and is usually less effective than noncontact cutting.
1.1.2.3 Noncontact Cutting
Contact with tissue is necessary in bipolar diathermy but most urological diathermy is monopolar and non‐contact. For current to cross a gap between a resectoscope loop and the prostate it must be driven by a sufficient voltage to ionise the intervening medium and produce a spark. (A spark is a less sustained discharge than an ‘arc’.) Once established, a spark produces the very high temperatures needed for cutting. However, most of its energy is dissipated near the tissue surface, and little is available to give the gentler heating needed for deeper coagulation and haemostasis.
The cutting current is a continuous simple sine wave. The peak voltage provided is enough to give short, intense sparks which produce enough local heat to explode cells into steam (Figure 1.1.5). There is little coagulation and the cut is ‘pure’. For coagulation, the electrical energy must be applied more slowly so that the heat to which it is converted has time to spread below the surface of the tissue (i.e. the power supplied must be reduced).

Figure 1.1.5 (a) Typical diathermy waveforms. (b) Cutting current: a continuous sine wave provides short intense sparks, which explode cells into steam, but there is little heating below the surface and little coagulation (i.e. continuous high current, low voltage) (c) Coagulating current: short bursts of sine waves provide localised heating leading to coagulation (intermittent low current, high voltage).
In contact diathermy, so long as the effective voltage is sufficient to overcome the impedance of the contact interface, coagulation can be obtained by reducing the voltage of the sine wave current. Alternatively, the current density can be reduced by increasing the contact surface of the electrode.
A high voltage is essential to drive the spark in noncontact diathermy, and a different method must be used for coagulation. The total current supplied in a given time, hence the rate of heating, is reduced by applying the current in bursts. In the gap between the bursts, no current flows. Because the coagulation current is turned off most of the time, it can have large peak voltages and currents but actually deliver much less power than a continuous cutting current. Fulguration (literally flashing like lightning) is provided by a current with an interrupted waveform. This has a high peak voltage but the effective power can be the same as a cutting current with a much lower voltage peak. The resulting sparks are longer, and there is more sustained tissue heating leading to coagulation and haemostasis. The high peak voltage can drive current through the high impedance of desiccated tissue: thus fulguration can continue until carbonisation or charring occur.
In summary, the ‘cut’ current is typically a continuous sine wave, producing sparks whose heat explodes intracellular water to steam. The ‘coag’ current is a sine wave current supplied in bursts, which allows the sustained heating in depth needed for coagulation (Figures 1.1.5b and c). Peak voltage and mean power output can be varied by adjusting the duration of bursts of current to give a combination of cutting and coagulation; this is known as ‘blended’ current.
Diathermy output settings, that can be varied, are normally measured in Watts (Joules per second) and are the product of the voltage and current.
1.1.3 Dangers
1.1.3.1 Electrocution
Diathermy machines are manufactured to national and international safety standards which minimise the risk of any part of the machine becoming live with mains current. As with any electrical device, servicing must be regular and expert.
1.1.3.2 Fire and Explosion
Ignition of alcohol‐based solutions used for skin preparation is a well‐recognised complication of diathermy which is still reported each year by the medical defence societies. It occurs when the flammable fluid is allowed to pool on or under the patient. The surgeon must take care that all excess spirit is removed before using diathermy. Better still, disinfecting fluids containing alcohol should be avoided if a suitable aqueous alternative is available. Thankfully, explosive gases are rarely used in modern anaesthesia. If they are, diathermy is an unnecessary hazard and should be avoided.
1.1.3.3 Burns
Burns are the most common type of diathermy accident. They occur when the diathermy circuit is completed in some way other than that intended by the surgeon, usually an unauthorised flow of current to earth. Most monopolar generators are ground referenced by earthing the patient plate side of the output transformer via the metal case of the device (Figure 1.1.6). If the active electrode is touched to any earthed object, current will flow. When the system works properly, heating occurs only at the tip of the active electrode. The current passes through the patient’s body and escapes safely via the return electrode. Unfortunately, this long current path offers opportunities for alternative unwanted passage of current to earth. If the patient electrode is incorrectly attached, there is a danger that the circuit might be completed by a small earthed contact point. If the current density at this point is sufficient, the patient will be burned.

Figure 1.1.6 Monopolar diathermy generators are ground referenced by earthing the patient plate to the metal case of the device. There is a risk of a burn if the patient plate is incorrectly attached, and there is contact with any other earthed point (e.g. patient monitoring equipment).
Most devices monitor the attachment of the patient plate and sound an alarm when contact is inadequate. A simple method is to attach the plate by two wires through which a small current flows: if a wire breaks, the current is interrupted and the diathermy can be automatically inactivated. This checks the integrity of the connection of the plate to the diathermy machine. It does not guarantee that the plate itself is properly attached to the patient. Another safety device uses a small direct current, which by passing through the active electrode, the patient, and the patient electrode, monitors the integrity of the whole diathermy circuit. Other machines have even more sophisticated safety measures; however, it remains the surgeon’s duty to ensure that an appropriate plate is used and to supervise its attachment to the patient.
Unfortunately, burns may still occur at small earthed contact points where current will flow at the expense of even a correctly attached return plate. All patient monitoring equipment should be isolated from the earth wherever this is possible. Electrocardiograph electrodes should be well gelled and of large enough area to disperse the current. Needle electrodes should never be used. As a general rule, the return pad should be sited as near to the operation area as possible so that the main current path will be distant from other potential routes that the current might take to ground.
Pressure on the footswitch of most machines leads to activation of all the active electrodes which are connected. Any devices which are not in use must not be in contact with the patient. An unused electrode should be safely stored in an insulated quiver where it will be safe if the footswitch is inadvertently activated.
The surgeon and assistants are also liable to suffer burns when using diathermy equipment because they constitute an effective alternative path to earth. Such burns are particularly likely in the practice of ‘touching’ the live electrode onto another metal instrument such as tissue forceps grasping a bleeding vessel. Surgical gloves are not effective insulation against diathermy current, especially if they are holed. The person holding the instrument, often an unfortunate assistant, may receive a small but deep and painful burn.
Bipolar diathermy is intrinsically safer than monopolar diathermy because current passes between two small electrodes on the same handpiece. Secondary currents induced by the main radiofrequency may leak to ground, but they are too small to cause trouble. Unfortunately, in most urological applications, bipolar diathermy is not as useful as monopolar diathermy.
1.1.3.4 Neuromuscular Stimulation: The ‘Obturator Twitch’
Although the high‐frequency current used for surgical diathermy does not cause neuromuscular stimulation, the sparks which it induces may invoke secondary currents which can do so. The sparks make random electrical ‘noise’ in the midst of which are alternating frequencies able to induce a faradic effect. Such currents can be electronically suppressed by capacitors in the circuit. However, they may be sufficient to cause trouble in the special conditions of diathermy in the region of the ureteric orifices close to the course of the obturator nerve and the psoas muscle. The problem is seen with both ‘cut’ and ‘coag’ currents and can usually be abolished by full chemical neuromuscular blockade.
1.1.3.5 Pacemakers and Diathermy
Implanted pacemakers are not uncommon in patients who are elderly and come to urological surgery. Diathermy currents can interfere with the working of pacemakers, causing possible danger to the patient. This was more of a problem with some of the previous fixed‐rate devices, which could be fooled into delivering stimulation at such a high rate that dangerous dysrhythmias could result. Modern pacemakers are designed instead to be inhibited by high‐frequency interference so that the patient may receive no pacing stimulation at all while the diathermy is in use. Some demand pacemakers revert to a fixed rate of pacing, and it is essential to have a magnet available so that they can be reset if necessary.
A number of additional precautions are wise in these patients. First, if monopolar diathermy is to be used, the patient plate should be sited so that the current path does not pass through the heart or the pacemaker. Second, the heartbeat should be monitored throughout the operation. Lastly, a defibrillator should be on hand in case a dangerous dysrhythmia develops through malfunction of the pacemaker.
Precautions to avoid complications:
- Diathermy pad: over well‐vascularised area, away from any prosthesis, underlying skin free from scarring or hair, 70–150 cm 2
- Avoid inflammatory liquids, such as alcohol‐containing skin prep
- Patient should avoid contact with any other metal (e.g. drip stand)
- Avoid touching any other instruments with diathermy
- Pacemaker/ICD
- Is surgery necessary?
- Check with cardiologist or pacemaker clinic. May need preoperative check or reprogramming (fixed rate/monitor only sometimes via clinical magnet) and postoperative check.
- Ask patients to bring their card with all details.
- Diathermy pad away from pacemaker and electrocardiogram leads, diathermy machine away from pacemaker, use bipolar diathermy if possible, continuous heart rate monitoring, defibrillator and external pacemaker to be available, short bursts of diathermy, minimise operative time.
- Prophylactic antibiotics: avoid fluid overload.
- Postoperative check if surgery was an emergency.
- Is surgery necessary?
1.1.4 Urological Diathermy
Transurethral resection requires high‐power monopolar diathermy currents which must be handled with great care. Typically, higher power output settings are required (e.g. 160‐W cutting/60‐W coagulation compared to 30–40 W for open surgery) because the use of irrigation fluid rapidly dissipates the intense heat required. There is an almost inevitable leakage of diathermy current from the loop to the metal instrument which poses a potential danger to both the surgeon and the patient. Most resectoscopes now have an all‐metal design with an insulated beak so that current that travels into the instrument is free to leak from it into the urethra. This is not usually a problem because the area of contact with the urethra is sufficient to make a burn unlikely. However, if through some fault, the loop comes into direct contact with the sheath, the full diathermy output will be applied to the urethra.
A fully insulated sheath might be expected to give protection against this hazard; unfortunately, this has dangers of its own. Damage to the insulating layer will lead to unpredictable leakage into the patient or the surgeon. If the loop should break and make contact with the metal frame of the instrument, a large current could flow to ground via the surgeon’s body. Such currents are usually prevented by the return fault circuit of the machine, but small and significant currents may pass to ground during fulguration.
Conducting lubricating gels should be used with all metal resectoscopes to avoid the possibility of preferential conduction at sites where the gel is thin or absent. By contrast, petroleum jelly or mineral oil, which do not conduct electricity, must be used only with an insulated sheath because these lubricants cannot provide an alternative path between the loop and the urethra, and they always end up smearing the lens.
1.1.4.1 LigaSure Diathermy
This utilises pressure and energy to seal vessels. It is a bipolar device which allows the administration of high current and low voltage energy (180 V) and high coaptive pressure during the generation of tissue temperature under 1000 °C, which results in sealing of vessels up to 7 mm in diameter. Once the energy is applied, the hydrogen cross‐links rupture and then renature, resulting in a vascular seal that has high tensile strength. The melted collagen and elastin in the vessels form a permanent seal.
1.1.4.2 Harmonic Scalpel
The harmonic scalpel utilises ultrasound energy to cause coagulation at lower temperatures than electrosurgical equipment (50–100 °C compared with 150–400 °C). Coagulation occurs by the coaptation or compression of the vessels walls followed by denaturing protein when the instruments blades vibrate at 55 500 Hz (i.e. 55 500 vibrations/s). The ultrasound transducer located in the hand piece is composed of piezoelectric crystal sandwiched under pressure amongst metal cylinders. The ultrasound generator converts ultrasonic energy into mechanical energy or the vibrations.
This comprises the utilisation of both the harmonic scalpel and the LigaSure, by simultaneously delivering ultrasonically generated frictional heat energy and electrically generated bipolar energy.
1.1.5 Lasers in Urology
LASER is an acronym for light amplification by the stimulated emission of radiation. Laser energy is now ubiquitous in surgery and perhaps indispensable for some purposes, such as transmitting energy down a flexible endoscope (e.g. holmium: YAG laser ablation of upper tract stones via a flexible ureterorenoscope).
Laser energy is light energy which, like electromagnetic radiation in general, may interact with matter to create heat and other phenomena. The characteristics of light is that it is that form of electromagnetic radiation defined by its ability to be perceived by the human eye, although infrared and ultraviolet radiations, which also interact with biological systems, are included within this definition. The wavelengths of visible light range from 400 nm (violet) to 760 nm (red) and form only a small part of the electromagnetic spectrum [7].
Laser light differs from conventional white light only in that it is:
- Monochromatic: consisting of light waves propagating at a single frequency
- Collimated: the photons propagate in parallel via narrow beams with little divergence, resulting in high pinpoint irradiance.
- Coherent: the propagated waves are such that wave peaks and troughs are in phase.
Electromagnetic radiation propagates in wave form, characterised by a frequency (ν), which is inversely proportional to its wavelength (λ) and related to the speed of light as follows:

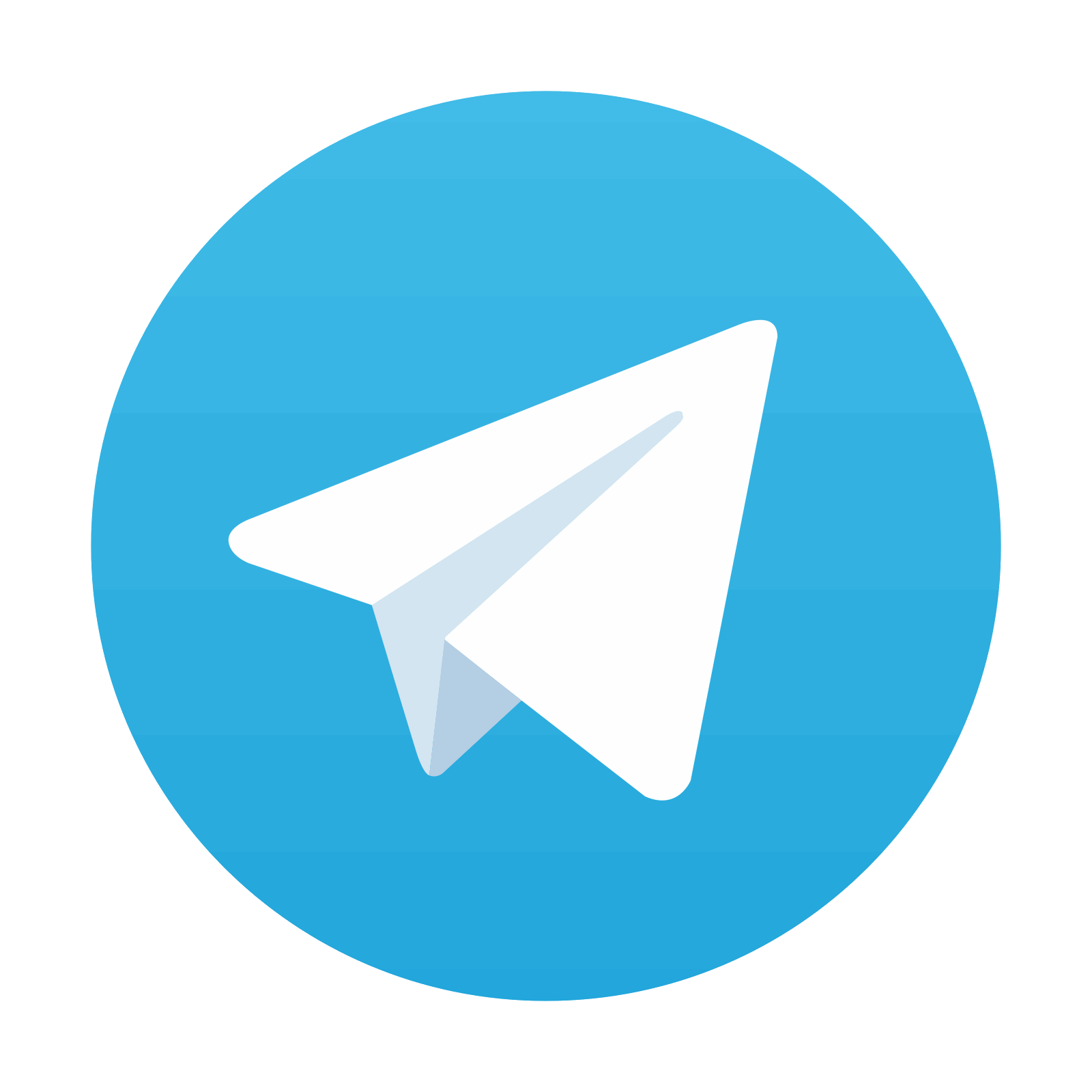
Stay updated, free articles. Join our Telegram channel

Full access? Get Clinical Tree
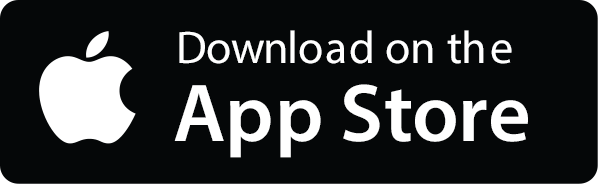
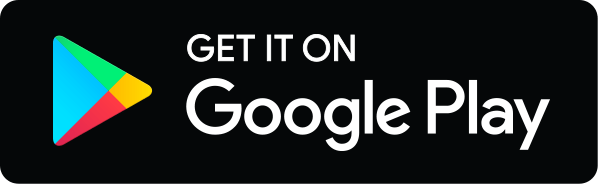