5 Hiro Ishii1, Omar M. Aboumarzouk2,3, and Hendrik Van Poppel4 1 Department of Urology, University Hospital Southampton, Southampton, UK 2 Glasgow Urological Research Unit, Department of Urology, Queen Elizabeth University Hospital, Glasgow, UK 3 University of Glasgow, School of Medicine, Dentistry & Nursing, Glasgow, UK 4 Department of Urology, University Hospital of the KU, Leuven, Belgium The kidneys are retroperitoneal organs encased by layers of fascia and fat and are responsible for excreting the end products of the metabolism and excess water to maintain the body’s electrolyte and fluid balance. With slight variation in size and location on either side of the 12th dorsal vertebra, they receive arterial supply directly from the aorta and drain directly into the vena cava. Converging to the renal sinus, the basic functional structure of the kidneys are the renal pyramids, projecting into minor calyces as papillae. The minor calyces unite to form the three or more major calyces which drain into the infundibula. From the upper, middle, and lower poles, together forming the renal pelvis, the urine is pushed downwards in a funnel‐shape pelviureteric junction that continues in the ureter. The ureter is a muscular tube that actively propulses the excreted by‐product, the urine, with a peristaltic movement and receives its blood supply from most major arteries in its course, which anastomose longitudinally. The ureter ends by burrowing into the bladder in an oblique manner, giving rise to a functional valve that prevents reflux. Keywords: kidney; renal pelvis; ureter; glomeruli; nephron; pyramids; calyx; renal artery; renal; The main function of the simplest renal system, such as the excretory vacuole of the amoeba, is to regulate osmolarity. The basic unit of the mammalian kidney is the renal pyramid. It is arranged like a bunch of flowers in a vase. The flowers are the glomeruli, the stalks are the collecting tubules, and the vase is the calix (Figure 5.1). Figure 5.1 Anterior relations the kidneys (a) on the left the duodenum and colon, (b) on the right, (c) liver. In the adult human, the pyramids are compressed so that the external surface is smooth, but the original lobulation can be seen in the foetal kidney where it resembles a bunch of grapes and is similar to aquatic mammals such as porpoises, whales, and otters [1]. Some mammals like rabbits have only one large calyx, whereas others like pigs and man have about a dozen calices. In man, the design of the pyramid is important in preventing reflux of urine up into the renal parenchyma. The kidneys lie protected from injury by the spine, ribs, and a thick packing of fat. Each kidney is about 11 cm in length, 6 cm wide, and 3 cm thick. A rough way to determine the vertical length of a kidney is to multiple the height of a vertebral body by 2.5. The left kidney is usually longer than the right kidney. The right kidney also tends to sit lower down than the left because of the liver. The average weight of a kidney is 150 g in a male and 135 g in a female. Superiorly, they are level with the upper border of 12th dorsal, and inferiorly, of the 3rd lumbar vertebra. The mid pole of the right kidney is level with the 12th rib and that of the left kidney is level with the 11th and 12th ribs. Figure 5.2 Posterior relations of the kidneys. Originally, the kidney is enveloped in a loose packing of fat (Zuckerkandl fascia) (Figure 5.3), contained within a thin, firm fascia (Gerota fascia). The Gerota fascia is a multilaminated structure that is fused posteromedially with the muscular fasciae of psoas major and quadratus lumborum [2]. This fascia then extends anterolaterally as a bilaminated sheet and then divides into the anterior lamina, which passes anteriorly to the kidney to form the anterior perirenal fascia and the thicker posterior lamina. This posterior lamina continues as the lateral conal fascia, blending ultimately with the parietal peritoneum [2]. Figure 5.3 The fasciae surrounding the kidney. Note that Gerota fascia is tough enough to tamponade haemorrhage from the ruptured kidney. Ao, aorta; IVE, inferior vena cava. Gerota fascia is the most important layer surgically. This is a layer of connective tissue encapsulating the kidneys and the suprarenal glands. Anteriorly, the fascia passes anterior to the kidney, its vessels, the abdominal aorta, and inferior vena cava and fuses with the anterior layer of the other kidney. Posteriorly, it fuses with the psoas major and the sides of the vertebral bodies. Superiorly, the anterior and posterior segments fuse to envelope the suprarenal gland and make attachments with the diaphragmatic fascia. Inferiorly, the anterior and posterior segments do not fuse, but go on separately to fuse with the connective tissue of the iliac fossa and the iliac fascia, respectively. The cross‐section of the kidney shows two distinct zones, the cortex and medulla. The cortex contains the glomeruli. The medulla is divided into an inner and outer zone according to the presence of the long or short loops of Henle (Figure 5.4). Between each pyramid is a portion of cortex, the column of Bertin (Figure 5.4). Figure 5.4 Diagrammatic section through the kidney. The paired renal arteries leave the aorta just 3–4 cm below the origin of the superior mesenteric artery. The right renal artery is longer and mostly located higher than the left, as it passes posterior to the inferior vena cava, right renal vein. The left renal artery passes behind the left renal vein, pancreatic body, and definitely lower than the splenic vein. Classically each kidney is supplied by a single renal artery. However, anatomic variations are common, where two or three renal arteries can supply the kidney with frequencies of multiple arteries ranging between 9 and 76% [3]. The arterial supply to the kidney was first mapped out by F. T. Graves in 1954 using injection‐corrosion casts of the renal vessels [4]. He found that there were five main renal segments arranged like fingers of a hand and each of these had its own artery (Figure 5.5). The segments are divided into the apical, upper, middle, lower, and posterior segments. This knowledge makes it easier to interpret angiograms, but it must be understood that variations are common; one or more of the segmental or polar arteries may spring independently from the aorta. Where the kidneys are not in their anatomical position (i.e. pelvic kidney), the segmental arteries may arise from any nearby vessels (e.g. aorta, lumbar, common, or internal iliac arteries). In addition, polar arteries occur frequently, and these need to be remembered during a nephrectomy. Figure 5.5 (a and b) Segmental arterial supply of the kidney. Each segmental artery supplies a discrete segment of the kidney; therefore, an occlusion of a branch can potentially lead to complete infarction of a complete segment of parenchyma. However, vascularity from neighbouring segments often occurs. These segments do not correspond to the pattern of the calices and pyramids, as in the bronchopulmonary segments, which makes is more difficult to plan a partial nephrectomy. However, it does make it possible to carry out any operation on the kidney in a virtually bloodless field once the main segmental renal artery is occluded, with the only slight blood loss from the venous system. Each segmental artery divides into branches that run toward the cortex where they give off arcuate arteries, from which every glomerulus receives its afferent arteriole (Figure 5.6). The efferent arterioles from the glomerulus run among the proximal and distal tubules. The efferent arteries from the innermost row of glomeruli (juxtaglomerular) send long straight branches down into the papilla, among the collecting tubules and long loops of Henle. On entering the medulla, these afferent arterioles divide into 12–25 descending vasa recta and supply the aforementioned structures. An important feature of the vasa recta is that both the ascending and descending vessels are grouped into vascular bundles, where they are intimately apposed, locating them close to the limbs of the loops of Henle and the collecting ducts. The proximity of the descending and ascending vessels with one another and adjacent ducts provides the structural basis for the countercurrent mechanism that concentrates the urine. Figure 5.6 Branches of the segmental arteries within the kidney. The renal papilla is supplied by the vasa recta as well as the spiral arteries of Baker. The vasa recta open into wide, thin walled capillaries in the tip of the renal papilla, which ramify between the ascending loops of Henle and collecting tubules. The renal veins, unlike the arteries, communicate freely with each other (Figure 5.7). Tributaries from each renal pyramid drain into larger veins around the pyramid that end in the main renal vein. Interlobular veins drain the superficial part of the cortex, and these pass to the corticomedullary junction and receive some ascending vasa recta. These then end in arcuate veins and anastomose with other neighbouring veins to form the renal vein. The extensive communication between the various veins means that obstruction of one or more of the tributaries has little effect on overall drainage. Figure 5.7 The renal veins communicate freely with each other. Not shown are the many small emissary veins from the cortex to the perirenal fat, which prevent the kidney from becoming infarcted when the main renal vein is obstructed. Usually there is one large renal vein draining each kidney. The renal vein is located anterior to the renal artery, although as is the case with much of the human anatomy, the position can vary up to 1–2 cm cranially of caudally relative to the renal artery. On the left side, the renal vein receives the suprarenal and gonadal veins joining the inferior vena cava laterally. In comparison to the right renal vein, the left enters the inferior vena cava slightly more cranially and anterolaterally than the right. On the right side, the renal vein is much shorter draining directly into the lateral to posterolateral aspect of inferior vena cava without receiving any tributaries, while the right gonadal and adrenal veins end in the inferior vena cava directly. The vascular anatomy can vary in up to 50% of kidneys (9). The most common variation is multiple renal arteries (10) and is more common on the left side. These additional arteries may enter through the hilum or as a branch of the main artery or into the parenchyma directly as a separate branch from the aorta. The presence of these polar arteries can make dissection and mobilisation of the kidney a real struggle because these arteries are often shorter than their normal counterparts. As well as making mobilisation of the kidney a challenge, these arteries, especially the lower pole arteries, can lead to extrinsic compression on the ureter at the ureteropelvic junction, leading to ureteropelvic junction obstruction. When the kidney is ectopic, the presence of multiple renal arteries is more common, and the origin of these multiple renal arteries is varied. Furthermore, polar arteries, which tend to be short and difficult to dissect, can greatly hinder the mobilisation of the kidney, in view of a partial nephrectomy, whereas lower pole polar arteries can give rise to a pelviureteric junction obstruction. Multiple renal veins are not as common as renal arteries, but they still exist (9). The most common variation is that of duplicate renal veins draining the right kidney via the right renal hilum. The other major variant is that involving the left renal vein. This may cross posterior to the aorta or may even divide and sandwich the aorta on its course to the inferior vena cava. The final surgically important variant is the lumbar veins; these may enter the renal vein on either side from a posterior position and may cause significant haemorrhage if not properly recognised and torn during surgery. The kidneys have a profuse lymphatic system that largely follow the vasculature through the columns of Bertin and then form multiple large trunks within the renal sinus. On reaching the hilum, branches from the renal capsule, perinephric tissues, renal pelvis, and upper ureter join the parenchymal branches to form three to four main trunks that run medially into the cisterna chyli. When the ureter is obstructed, these lymphatics act as an efficient safety‐valve Upon leaving the hilum, there is a slight symmetry in the drainage of the left and right side. On the left side, the main drainage is into the left lateral para‐aortic nodes, and there will occasionally be additional drainage into the retrocrural nodes, or there may be direct drainage into the thoracic duct above the diaphragm. On the right side, drainage is into the right interaortocaval and right paracaval nodes, as well as the nodes sandwiching the aorta anteroposteriorly. The kidney has the second richest innervated organ per unit of tissue mass second only to the adrenal gland [5]. Sympathetic preganglionic nerves originate from the eighth thoracic to first lumbar spinal segments. These fibres then travel to the coeliac and then the aorticorenal ganglia. The postganglionic fibres then reach the kidney via the autonomic plexus along the renal artery. The function of the autonomic innervation is the control of vasomotor activity of the kidney. The sympathetic innervation causes vasoconstriction, and the parasympathetic innervation causes vasodilatation. An important point to make is that even without this autonomic control, the kidney can function perfectly well, as demonstrated in a transplanted kidney. The nephron is the basic functional unit of the kidney, consisting of two parts: (i) the glomerulus, where the water and solutes are filtered from the blood and (ii) the tubules where the filtrate is processed. The glomerulus consists of a convoluted arteriole, which is invaginated into Bowman capsule on a stalk, the mesangium (Figure 5.8) [6]. At the base of the stalk, the afferent arteriole is surrounded by the macula densa. These are endothelial cells containing granules that are the precursor of renin. The juxtaglomerular body, which contain beta‐adrenergic receptors, is a pressure‐sensing mechanism that responds to changes in blood pressure in the afferent arteriole (Figure 5.9). Figure 5.8 The nephron. Figure 5.9 The juxtaglomerular body. The endothelium of the arteriole of the glomerulus and the epithelium lining Bowman capsule are separated by a basement membrane that serves as a filter to retain cells and proteins in the blood (Figure 5.10) [6]. This basement membrane rests on specialised epithelial cells that have interlocking foot‐processes (podocytes) (Figure 5.11) [6]. These, along with the capillary epithelium, form a selective barrier across which urinary filtrate pass. The filtrate drains into Bowman capsule and then moves to the proximal convoluted tubule. This is a thick‐walled, metabolically active structure whose brush border increases the surface area offered to the glomerular filtrate. The proximal tubule is composed of a thick cuboidal epithelium covered by dense microvilli, the brush border. The microvilli do most of the metabolic work involved in the reabsorption of salt and water. They are also the cells that give rise to renal cell carcinomas. Figure 5.10 The basement membrane lies between the endothelium of the glomerular arteriole and the epithelium of Bowman capsule. Figure 5.11 The basement membrane supported by foot processes of the epithelial cells. From the proximal tubule, the glomerular filtrate passes to the loop of Henle. Some of these loops are short, whereas others, especially those from the innermost row of nephrons next to the medulla, are long and reach to the tip of the papilla. As the loops ascend out of the medulla, the loop thickens to become the distal convoluted tubule. These tubules also have metabolically active cells, but unlike the proximal tubules, do not have a brush border. The distal tubules return to a position adjacent to the originating glomerulus and proximal tubules. Another turn and the distal tubules become the collecting tubules. The collecting tubules receive filtrates from 10 to 15 nephrons, becoming the collecting duct and descend through the medulla to open at the tip of the papilla. The collecting tubule is thin walled and its cells are metabolically inert. Each renal papilla is made up of collecting tubules, loops of Henle, the vasa recta, and veins. Typically, there are 7–9 papillae in each kidney, but can vary between 4 and 18. The papilla protrudes into the calyx and the collecting ducts open through a series of oblique slits along the sides of the papilla. The configuration is such that when the pressure is increased inside the papilla, the collecting ducts are occluded; thereby urine is not forced up into the parenchyma of the kidney (Figure 5.12). The papillae are organised in two longitudinal rows, orientated at roughly 90° to each other. Each of the papillae are cupped by a minor calyx. Figure 5.12 The normal valvular arrangement of collecting tubules in the renal papilla, which prevents intrarenal reflux when pressure rises in the calix. Not all papillae are perfectly formed, especially those in the upper and lower poles, where compound papillae are a common congenital anomaly. In such anomalies, there is no valve mechanism to prevent the reflux of urine (Figure 5.13). This malformation becomes important in reflux nephropathy, where the parenchyma becomes much scarred because of the reflux. These compound calyces are the result of renal pyramid fusion. Figure 5.13 Congenitally deformed papillae, or compound papillae, may permit intrarenal reflux causing reflux nephropathy. The blood supply to the papillae is twofold: (i) via the vasa recta, which loop down from the efferent arterioles of the glomeruli and (ii) via the smaller arteries entering at the base of each papilla from the rim of the calyx (Figure 5.6). After the cupping of a papilla, each minor calyx narrows into an infundibulum, and these combine to form two or three major calyceal branches. These calyces are commonly termed the upper, middle, and lower pole calyces. The calyces are lined by the same pattern of smooth muscle and transitional epithelium as along the whole length of the ureter. For the smooth muscle to work efficiently as a pump, it must be free to contract, expand, and move up and down (Figure 5.14). Figure 5.14 The sinus fat allows free movement for the contraction and expansion of the renal pelvis and calices. These calyces combine to form the renal pelvis. The renal pelvis can be a relatively small intrarenal pelvis, but it can also be a large extrarenal pelvis, commonly mistaken as a dilated system. The pelvis is enveloped by a slippery layer of fascia that is easily stripped off when operating on a hydronephrotic kidney. Outside this layer, there is a thick packing of sinus fat. The slippery layer of fascia continues down the ureter, and this allows it to writhe behind the peritoneum with each wave of peristalsis and to move freely up and down with respiration. The pelvis then narrows to form the ureteropelvic junction, marking the start of the ureter. The ureters are tubular structures responsible for the transportation of urine from the kidneys to the bladder. The length ranges from 22 to 30 cm, and they have a wall made up of multiple layers. The innermost layer is made up of transitional epithelium, surrounded by the lamina propria, which is a connective tissue layer. These two layers make up the mucosal lining. The next layer is made of smooth muscle that, as mentioned previously, is continuous with that of the calyces and the renal pelvis. However, one slight difference is that within the ureter, the smooth muscle layer is divided into an inner longitudinal and an outer circular layer. These muscular layers allow peristalsis of urine. The outermost layer is the adventitia, which is a thin layer enveloping the ureter, its blood vessels, and lymphatics. The ureter is often divided into three segments: the upper (proximal), middle, and lower (distal) segments. The upper segment starts from the renal pelvis to the upper border of the sacrum. The middle segment is the part between the upper border and lower border of the sacrum. The lower segment is from the lower border of the sacrum to the bladder. Each ureter lies posterior to the renal artery and vein at the ureteropelvic junction. They then descend anterior to the psoas major muscle and the ilioinguinal nerves, just anterior to the tips of the lumbar transverse vertebral processes. Approximately a third of the way down, the ureters are crossed by the gonadal vessels. They then cross the bifurcated common iliac arteries and run along the anterior border of the internal iliac artery toward the bladder, which it pierces in an oblique manner (Figure 5.15). It is this oblique entry of the ureter into the bladder, the intramural segment of the ureter that acts as a nonreturn valve preventing vesicoureteric reflux [7]. This valve can be congenitally defective such as that seen in those with short intramural segments, or rendered ineffective as a result of injury, such as surgery or disease, all of which leads to reflux. Many congenital abnormalities of this oblique tunnel are seen in association with a duplex kidney and ureterocoele. In its lower third, the ureters pass posterior to the superior vesical branch of the internal iliac artery also called ‘umbilical artery’. On the right side, the ureter is related anteriorly to the second part of the duodenum, caecum, appendix, ascending colon, and colonic mesentery. The left ureter is closely related to the duodenojejunal flexure of Treitz, descending and sigmoid colon, and their mesenteries. Figure 5.15 Main ureteral relations. To find the ureter during an operation, the surgeon’s most useful landmark is the bifurcation of the common iliac artery. When operating on the lower third of the ureter, it is helpful to first ligate and divide the superior vesical artery. In males, the ureter passes under the vas deferens just as it approaches the bladder. In females, the ureter runs through the cardinal ligament (the ligament of Mackenrodt) (Figure 5.16). They are also crossed anteriorly by the uterine arteries within the pelvis. It is at this point that the ureter can be easily damaged during operations on the uterus. Figure 5.16 (a) Ureters running through the cardinal ligament. (b) Uterine prolapsed causing compression on ureters. Because of the close proximity of the ureters to several bowel segments, they can be involved in inflammatory and malignant processes that affect the terminal ileum, appendix, right or left colon, and sigmoid colon. The normal ureter does not have a uniform calibre throughout its course. There are two areas of distinct narrowing: the ureteropelvic and the ureterovesical junctions. At the ureteropelvic junction, the renal pelvis tapers into the proximal ureter. At the ureterovesical junction, there is a true physical narrowing as the ureter burrows through the bladder wall to enter at it at the ureteric orifice, the intramural ureteric segment. As mentioned, this acts as a valve preventing vesicoureteric reflux [7]. A third point where there is possible hindering of passage of the urine bolus or a stone is where the ureter crosses the iliac vessels. This area of hindrance is caused by two factors: the extrinsic compression from the iliac vessels and the necessary angulation of the ureter to enter the pelvis. It is at these three points where urinary calculi are often lodged, causing ureteric colic. As well as narrowing, the knowledge of the angulations of the ureter is vital during ureteroscopy. The blood supply to the renal pelvis is profuse and comes from branches of all the main segmental arteries, and these communicate freely within the pelvis. The abundant blood supply allows the construction of long flaps of renal pelvis to repair hydronephrosis without the fear of necrosis [8]. The ureter receives its blood supply from multiple arteries along its course. The upper ureter is supplied by the inferior segmental branch of the renal artery and is reinforced by the gonadal artery, abdominal aorta, and the common iliac artery. On entering the pelvis, smaller branches come off the internal iliac artery or its branches; the vesical and uterine arteries to supply the ureter. Important to the surgeon is the knowledge that the abdominal ureter receives its blood supply medially and the pelvic ureter receives its blood supply laterally. Upon reaching the ureter, the arteries run longitudinally within the adventitia of the ureter to form an extensive plexus (Figure 5.17). It is this longitudinal supply that allows the mobilisation of the ureter from the surrounding retroperitoneal tissues without compromising its blood supply, as long as there is no damage to the adventitia. If the longitudinal artery of the ureter is pulled on, it becomes even narrower; hence, in any operation on the ureter, it is essential to avoid tension. Figure 5.17 Ureteral blood supply. The venous and lymphatic drainage of the ureter runs with the arterial supply; therefore, there is segmental variation in its drainage. Within the pelvis, ureteral lymphatics drain to the internal, external, and common iliac nodes, whereas in the abdomen, the left para‐aortic nodes drain the left ureter and the right ureter drains into the paracaval and interaortocaval lymph nodes. The lymphatic drainage of the upper ureter and renal pelvis joins that of the corresponding kidney. Preganglionic sympathetic fibres from the 10th thoracic to 2nd lumbar spinal segments supply the ureter. The postganglionic fibres arise from several ganglia found in the aorticorenal, superior, and inferior hypogastric (pelvic) autonomic plexuses. The parasympathetic input to the upper ureter is through vagal fibres via the coeliac plexus and fibres to the lower ureter are from second to fourth sacral segments. Fine microscopic analysis of the ureter shows a network of fine plexuses in the muscular wall of the ureter [4]. However, these do not propagate ureteral peristalsis, as this continues perfectly and is seen in a completely denervated transplanted kidney. The wave of peristalsis originates and is propagated from the intrinsic smooth muscle pacemaker sites located within the minor calyces and the excitation of the propagation is carried from one cell to the next through close junctions. The entry of the ureter into the bladder is along an oblique tunnel, which provides a nonreturn valve preventing vesicoureteric reflux. This valve can be congenitally defective or put out of use by injury or disease. Many congenital deformities of this oblique tunnel are seen in association with duplex kidney and ureterocele (Figure 5.18). Figure 5.18 The antireflux ureterovesical valve. The renal pain fibres are stimulated by distension within the renal capsule, collecting system, or ureter. As well as distension, direct irritation to any of them can stimulate nociceptors. These pain signals travel with the sympathetic fibres, and the pain is felt in the segmental distribution of the kidney or ureter (T8 to L2). The pain is felt over the distributions of the subcostal, iliohypogastric, ilioinguinal, or genitofemoral nerve fibres. This can result in pain in the flank, groin, or scrotum or labia.
Kidney and Ureter Anatomy
Abstract
5.1 Comparative Anatomy
5.1.1 Topographical Anatomy
5.1.2 Anatomical Relations
5.2 Renal Fasciae
5.3 Macroscopic Appearances
5.4 Arterial Supply
5.5 Renal Veins
5.6 Common Vascular and Anatomic Variations
5.7 Lymphatics
5.8 Innervation of the Kidney
5.8.1 The Nephron
5.8.2 The Glomerulus
5.8.3 Renal Papillae, Calyces, and Pelvis
5.8.4 The Ureters
5.8.4.1 The Anatomic Relations of the Ureter
5.8.4.2 The Calibre of the Ureters
5.8.5 Renal Pelvis and Ureteral Blood Supply
5.8.5.1 Ureteral Innervation
5.8.5.2 Renal and Ureteric Pain
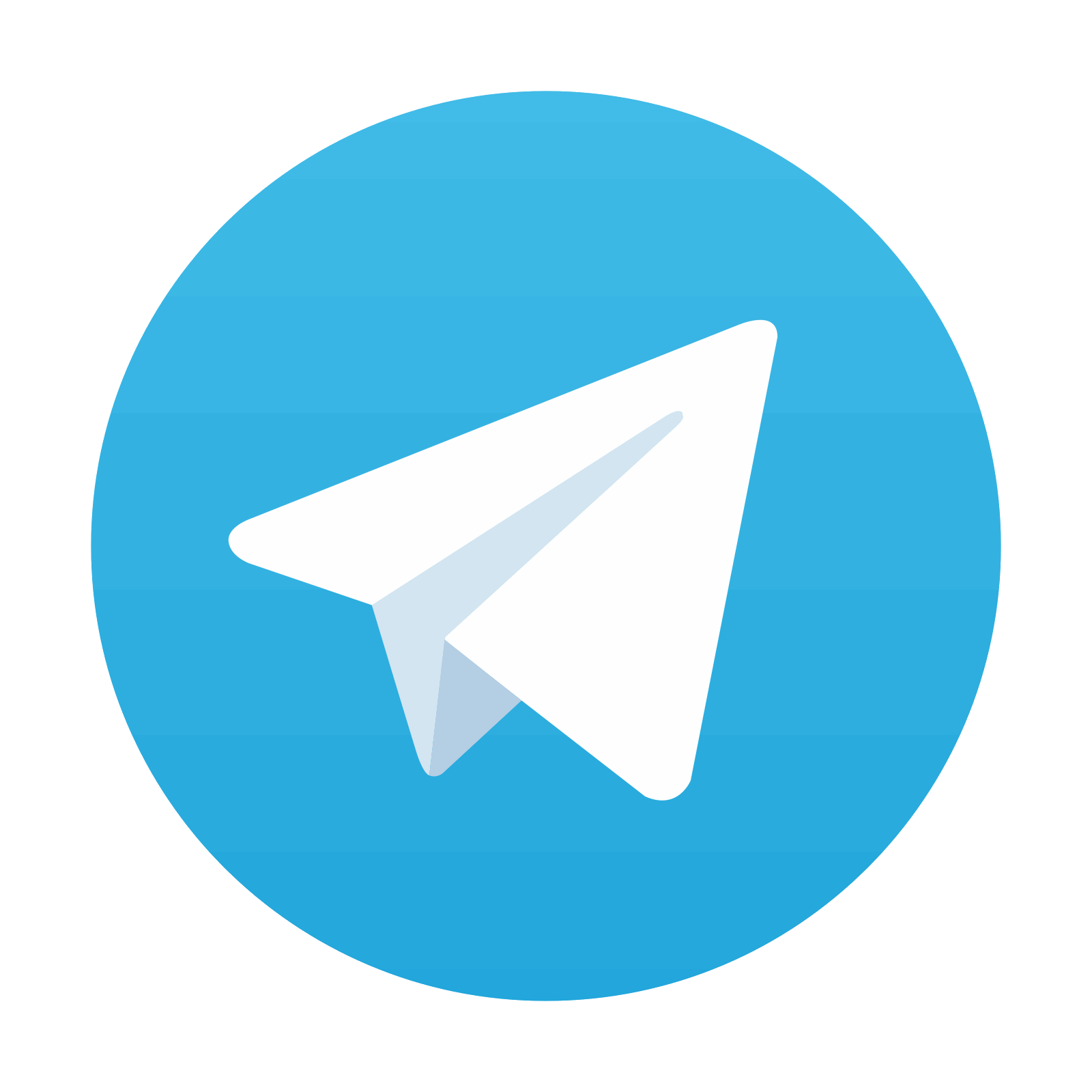
Stay updated, free articles. Join our Telegram channel

Full access? Get Clinical Tree
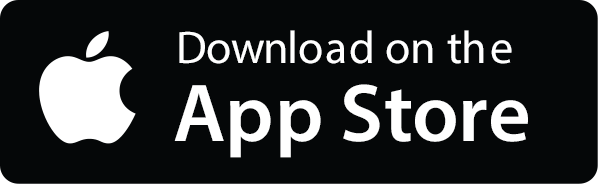
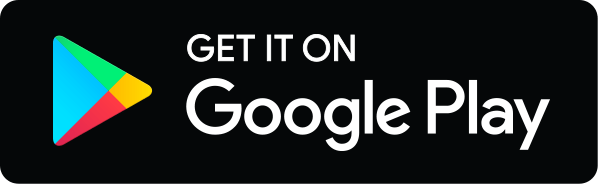