Chapter 93A Preoperative portal vein embolization
Rationale, indications, and results
Overview
Although recent advances in surgical techniques and perioperative care have significantly improved both short- and long-term outcomes after hepatic resection (Belghiti et al, 2000; Fan et al, 1999; Grazi et al, 2001; Imamura et al, 2003; Jarnagin et al, 2002), postoperative liver volume insufficiency is still a major concern after an extensive liver resection. Both the small size of the remnant liver parenchyma after an extensive liver resection and sinusoidal injury caused by an abrupt increase in portal venous flow/pressure may be implicated in an increased rate of postoperative morbidity associated with cholestasis, ascites, and impaired synthetic function (Panis et al, 1997). These conditions all may lead to a protracted recovery and extended hospital stay and may eventually result in fatal liver failure, although rarely. This concern is even more relevant in patients with obstructive jaundice or underlying liver disease; that is, in those who not only have liver fibrosis or cirrhosis but also liver injury induced by chemotherapy, originally in patients with normal liver (see Chapters 50B, 65, 87, and 90F).
In 1982, we first carried out a preoperative portal vein embolization (PVE) for a patient with hilar bile duct carcinoma with the intention of increasing the operative safety under these circumstances (Makuuchi et al, 1984, 1990). The approach was based on the concept of the hepatic atrophy-hypertrophy complex (see Chapter 5). We attempted to initiate hypertrophy of the future liver remnant (FLR) before resection to induce atrophy in the embolized part of the liver to be resected with compensatory hypertrophy of the nonembolized FLR. Satisfactory initial outcomes led us to extend the indications for PVE to patients with metastatic liver tumors of colorectal origin (Kawasaki et al, 1994a) and for patients undergoing hepatopancreatoduodenectomy (Kawasaki et al, 1994b). Because of its technical simplicity and the consistent attainment of liver hypertrophy after the procedure, PVE has become a standard preoperative intervention worldwide, and its indications have been expanded to patients with hepatocellular carcinoma (HCC) (Aoki et al, 2004; Azoulay et al, 2000a; Lee et al, 1993; Ogata et al, 2006; Tanaka et al, 2000; Yamakado et al, 1997).
Historic Background
The concept of the hepatic atrophy-hypertrophy complex dates back to 1920, when Rous and Larimore (1920) showed that ligation of a major branch of rabbit portal vein resulted in atrophy of the ipsilateral hepatic lobe and hypertrophy of the contralateral lobe. The same phenomenon was observed in experiments involving long-term bile duct obstruction (McMaster & Rous, 1921); however, in contrast to the predictable response to portal venous occlusion, the magnitude of response to bile duct obstruction differs substantially among species (Braasch et al, 1972; Schalm et al, 1956; Steiner & Martinez Batiz, 1962; Stewart et al, 1937).
In 1975, Honjo and colleagues ligated the ipsilateral portal venous branch in patients with HCC in an effort to suppress tumor growth. Although this approach did not succeed in preventing tumor growth, it did produce marked atrophy of the occluded hemiliver. Likewise, our patients experienced an uneventful postoperative clinical course after extensive hepatectomy for hilar bile duct carcinoma involving a branch of the portal vein, causing hemiliver atrophy with corresponding hypertrophy of the contralateral hemiliver (Takayasu et al, 1986). These clinical observations led one of us (M.M.) to attempt PVE application as an adjunct procedure for major hepatectomy.
Hemodynamic Consequences
Total portal venous flow volume was thought to remain unchanged before and after PVE, because liver does not have an intrinsic ability to modulate portal flow, which is a function of extrahepatic (i.e., splanchnic and systemic) factors. This principle was confirmed in a study using a rat model of hemihepatic portal vein ligation and radioactive microsphere technique (Rocheleau et al, 1999) and in a human PVE study using Doppler ultrasound (Denys et al, 2000), proving that the same volume of portal venous flow as that prior to PVE would enter nonembolized liver segments after PVE. Portal pressure in the nonembolized hemiliver is elevated immediately after PVE by 4.9 ± 2.7 cm H2O (Takayama et al, 1997). A similar increment was observed in patients with cirrhosis, who had higher baseline portal pressure (Aoki et al, 2004). Information on chronologic alterations in portal pressure after PVE is unavailable, but the elevation is believed to be transient, with pressure gradually returning to the baseline value in 2 to 3 weeks, as indicated by the portal flow velocity (cm/sec) changes measured by transcutaneous Doppler ultrasound (Goto et al, 1998).
Our concern that an abrupt increase in portal venous pressure after major hepatectomy may result in liver damage is one of the two reasons why we had attempted PVE. This concern seems to have been given little attention compared with a concern that a diminished liver may not meet metabolic demands after resection. We believe that incremental increases in portal venous flow/pressure observed after PVE may increase tolerance in patients to high portal venous flow/pressure after extensive hepatic resection, resulting in an attenuation of liver damage after the subsequent resection (Makuuchi et al, 1991). Other recent studies on living donor liver transplantation (LDLT) have focused on portal pressure and small-for-size syndrome, and high portal pressure immediately after LDLT was reported to be associated with daily volume of ascites (Konishi et al, 2008). Attempts were made to attenuate this problem by splenic arterial ligation or portacaval shunt by some groups (Boillot et al, 2002; Man et al, 2003; Takada et al, 2004; Troisi et al, 2003, 2005).
In contrast to portal venous flow, liver is known to have an intrinsic regulatory mechanism to compensate even partially for alteration in portal flow, which is referred to as hepatic arterial buffer response (Lautt, 1985). In the case of PVE with hemihepatic portal vein occlusion or thrombosis of a portal branch, hepatic arterial buffer response is thought to still operate in embolized liver based on studies using dynamic computed tomography (CT) (Fig. 93A.1; Nagino et al, 1998; Wakabayashi et al, 2001), Doppler ultrasound (Denys et al, 2000; Kito et al, 2001), and three-dimensional magnetic resonance angiography (Gülberg et al, 2004), and their findings are in line with the report of a study using a rat model (Rocheleau et al, 1999); however, controversy surrounds the maintenance of hepatic arterial buffer response in nonembolized hemiliver (Denys et al, 2000; Kito et al, 2001; Rocheleau et al, 1999; Wakabayashi et al, 2001). Total liver blood flow, as estimated by a pharmacologic method, remained unchanged throughout the post-PVE period in our previous study (Shimada et al, 2002).
Because hepatic arterial buffer response is a partial compensation, hyperperfusion in a nonembolized hemiliver occurs after PVE, in contrast to hypoperfusion in an embolized hemiliver as expressed per gram of liver tissue. Corresponding hepatocyte regeneration in the nonembolized hemiliver occurs immediately after PVE proportional to the magnitude of the stimulus; however, little is known about when and how the atrophy-hypertrophy complex is terminated. Rocheleau and colleagues (1999) reported that, in a rat model of hemihepatic portal vein ligation, the hepatic atrophy-hypertrophy complex process (i.e., liver weight changes in the ligated and nonligated lobes) continues until total lobar flow per gram of liver tissue is restored. In Western series, planned hepatectomy after PVE had been scheduled when hypertrophy of the nonembolized liver segments seemed to reach their plateau, and full hypertrophy of the nonembolized segments was obtained 7 to 9 weeks after PVE (Azoulay et al, 2000a).
Clinical Course after Portal Vein CHEMOEmbolization
In contrast to transcatheter arterial chemoembolization (TACE), signs and symptoms of postembolization syndrome—such as pain, nausea, and vomiting—are minimal. Most patients experience mild fever after PVE, which subsides within 2 to 3 days. Changes in liver function, as reflected by an increased total bilirubin value and prolonged prothrombin time, are mild and transient, returning to their baseline values 2 to 3 days after PVE. Serum aspartate aminotransferase (AST) and alanine aminotransferase (ALT) values are found to be stable in half of the patients, and in those remaining, values are mildly elevated (1.5 to 3 times the pre-PVE values) on day 1, returning to baseline values in 4 to 7 days after PVE, regardless of the embolization materials used. These findings suggest that inflammatory and necrotic reactions after PVE are minimal, if they are present at all (Imamura et al, 1999a). The exceptions are when absolute ethanol is used for embolization (Shimamura et al, 1997), and when PVE is carried out after TACE (Aoki et al, 2004; Ogata et al, 2006). In both situations, PVE is followed by marked AST and ALT elevations, although both returned to baseline values by the time of scheduled hepatectomy (Fig. 93A.2).
Some have reported on adverse events (complications) or on morbidity rates associated with PVE (Table 93A.1). A multicenter European study of 188 patients who underwent contralateral PVE reported 24 adverse events (12.8%) (Di Stefano et al, 2005). Most adverse events were associated with the PVE technique, including complete portal thrombosis (n = 1), migration of embolic material in the main left portal vein feeding the FLR (n = 2), hemoperitoneum (n = 1), hemobilia (n = 1), rupture of a metastasis into the gallbladder (n = 1), and transient liver failure (n = 6). A recent meta-analysis documented overall morbidity rate for PVE to be 2.2% (Abulkhir et al, 2008). A summary of 37 publications involving 1088 patients showed a total of 497 minor complications and 11 major complications (see Table 93A.1).
Table 93A.1 Complications Following Portal Vein Embolization
Details | |
---|---|
Complication | Number |
Minor | |
Abdominal discomfort or pain | 209 |
Fever | 250 |
Nausea or vomiting | 26 |
Ileus | 9 |
Overflow of embolization materials | 2 |
Coil displacement | 1 |
Major | |
Liver abscess | 3 |
Cholangitis | 2 |
Main or left portal vein thrombosis | 2 |
Subcapsular hematoma | 2 |
Portal hypertension | 1 |
Septic necrosis from hepatic artery injury | 1 |
From Abulkhir A, et al, 2008: Preoperative portal vein embolization for major liver resection: a meta-analysis. Ann Surg 247:49-57.
Volumetric Consequences of Portal Vein Embolization
CT scan with contrast enhancement is the most commonly used method for calculating total liver volume (TLV) and FLR volume (FLRV). The nontumorous FLRV/(TLV – Tumor volume), or FLRV/TLV ratio, is a widely used parameter to determine whether PVE is necessary before hepatic resection and to assess the degree of FLR hypertrophy. Vauthey and associates (2002) proposed the use of standard liver volume (SLV), a concept first applied to clinical situations by us in 1995 (Urata et al, 1995). SLV is an alternative method for estimating TLV from a linear regression formula, correlating body surface area or body weight and TLV in healthy subjects (Urata et al, 1995; Vauthey et al, 2002). This formula is based on a concept that optimal liver/body mass ratio in healthy subjects is constant. We first referred to this optimal liver volume as SLV and proposed the application of SLV for recipients of LDLT and also for patients with end-stage liver disease, whose actual liver volumes are either extremely large or small in terms of ideal liver volume to be grafted, such as in cases of primary biliary cirrhosis or fulminant hepatic failure (Urata et al, 1995).
Vauthey and associates (2002) emphasized the importance of SLV as determining indications for PVE, because it may be applied to patients with pathologic liver or obstructive jaundice with dilated bile ducts and may even be calculated in patients with multiple liver metastases. Nevertheless, we adhere to the use of actual TLV for several reasons. First, SLV is a virtual volume estimated by using a formula, and different formulas are used among investigators; r2 in the equation of Vauthey and associates (2002) is 0.49, indicating that their formula explains only half of the interindividual variations for TLV even in healthy subjects. Second, patients with extremely large or small livers in terms of SLV because of underlying liver diseases may not be candidates for liver resection even after PVE. Finally, up-to-date devices such as multislice helical CT with contrast administration allow accurate volumetric measurement by subtracting small tumor volumes and vasculobiliary structures, even to Couinaud’s segment level.
PVE leads to an increment of segmental volume in nonembolized hemiliver and a decrement of segmental volume in embolized hemiliver, homogeneously maintaining a constant TLV (in most studies, TLV remained unchanged after PVE). In case of right hemiliver PVE, regeneration rate of the noncirrhotic liver was 12 cm2/day 2 weeks after PVE (Lee et al, 1993; Nagino et al, 1995), decreasing to 11 cm2/day at 4 weeks (Nagino et al, 1995) and then 6 cm2/day at 32 days (De Baere et al, 1996). In cirrhotic patients, regeneration is reportedly slower, at a rate of 9 cm2/day at 2 weeks (De Baere et al, 1996; Lee et al, 1993). In noncirrhotic and cirrhotic livers, regeneration processes are thought to be blunted by approximately 50% compared with that after hepatectomy (Yamanaka et al, 1993). Results of hypertrophy of nonembolized liver segments after PVE are summarized in Table 93A.2. In most series, hypertrophy of nonembolized liver segments in normal liver was 10% to 15%, expressed in volumetric ratio of nonembolized liver segments to TLV. The figure was smaller (5% to 10%) in diseased liver.
Various factors have been reported to affect the regeneration rate after PVE. Regeneration rate depends on embolized hemiliver volume (Imamura et al, 1999a; Yamakado et al, 1997). In other words, the greater the FLRV before PVE, the smaller the volume increase after PVE. Materials used for embolization affect the results in magnitudes of hypertrophy. Hypertrophy seemed to be modest when biologic materials such as Gelfoam and fibrin glue were used, most probably as a result of the progressive recanalization they induced. Absolute ethanol was reported to achieve the highest degree of regeneration but at the expense of marked increases in AST and ALT levels secondary to liver necrosis (Shimamura et al, 1997). Among the disorders related to background patient characteristics, diabetes, obstructive jaundice, active hepatitis, and cirrhosis have been reported to hamper the regeneration process (Imamura et al, 1999a; Nagino et al, 1995; Tanaka et al, 2000).
Histologic Consequences of Portal Vein Embolization
Human liver tissue obtained 3 weeks after PVE showed almost normal microscopic structures in the embolized and the nonembolized lobes. In the embolized lobe, dilation of sinusoids with decreased hepatocyte density and hepatocyte apoptosis were observed, especially in the pericentral area (Harada et al, 1997). Nevertheless, no signs of necrosis or inflammation were apparent, except when the embolizing material used was absolute ethanol, with tissue showing clear evidence of necrosis (Shimamura et al, 1997), or cyanoacrylate, which causes peribiliary fibrosis (De Baere et al, 1996
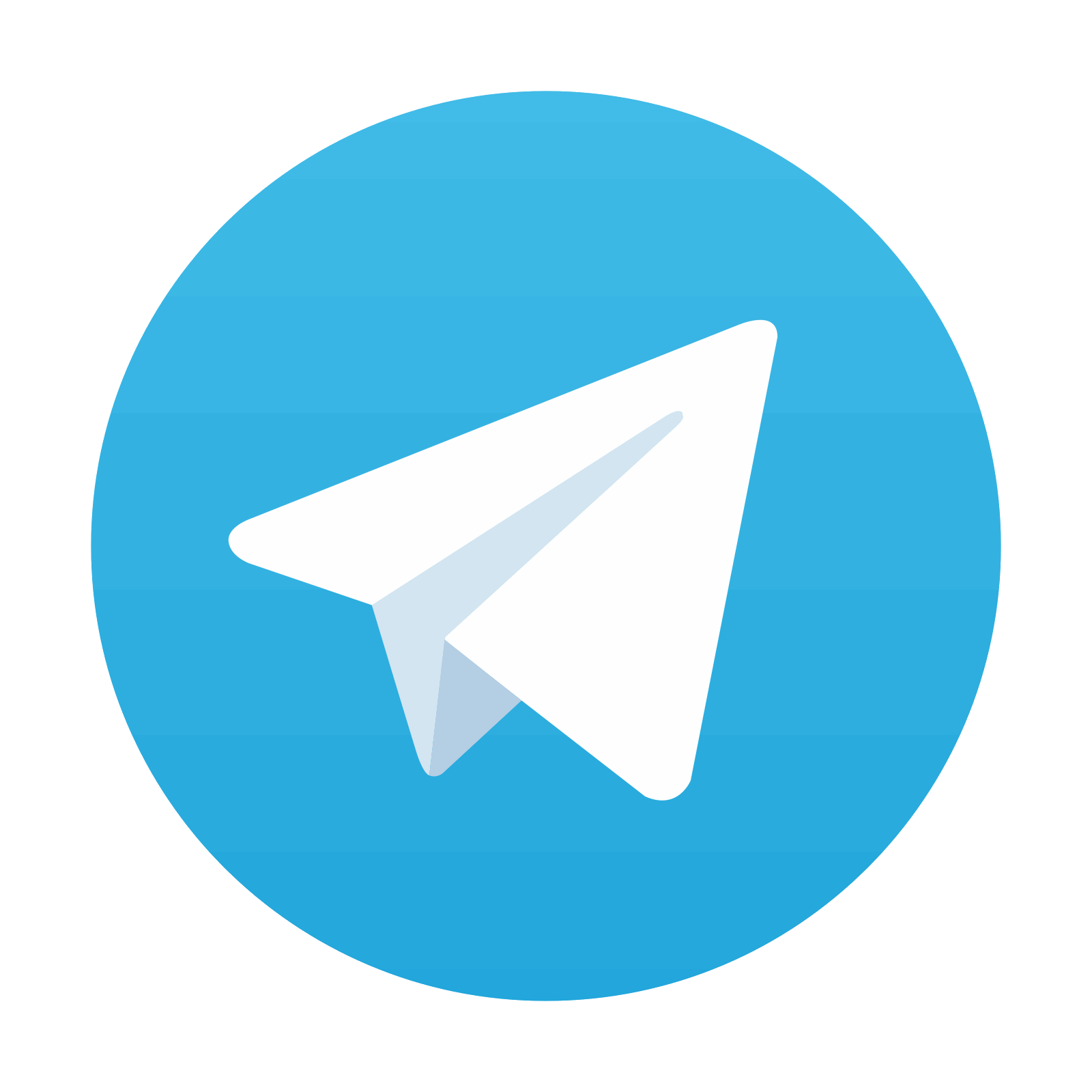
Stay updated, free articles. Join our Telegram channel

Full access? Get Clinical Tree
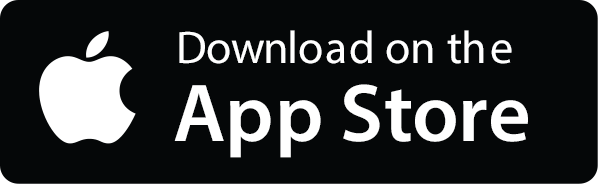
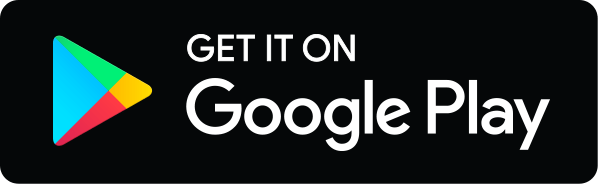