The adult kidney functions to keep the organism in a steady state and protect against changes in the volume and composition of the extracellular fluid. Unlike the adult, the neonate must be in positive balance for several solutes to promote growth.There are several developmental changes that occur during the postnatal maturation of the kidney that affect glomerular filtration as well as the mechanisms and regulation of tubular transport. Postnatal renal development is not simply due to developmental increases glomerular filtration rate with concomitant changes in abundance of transporters, but is also characterized by developmental changes in transporter isoforms and signal transduction pathways that are involved in regulation of transport. This chapter focuses on maturational changes in kidney function that occur during postnatal renal development with a focus on the differences between the adult and neonatal kidney.
Keywords
neonatal, renal physiology, transport, GFR
Renal Blood Flow and Glomerular Filtration Rate
Renal Blood Flow
The renal blood flow in the adult human is 660 ml/min which is 20–25% of the cardiac output. In contrast, the fetal kidney receives only 2% of the cardiac output from mid-gestation to term. The developmental increase in renal blood flow is due in part to an increase in cardiac output, but predominantly to a decrease in renal vascular resistance. When corrected for a body surface area of 1.73 m 2 , the human neonate has a renal blood flow of only 15–20% of that measured in adults. Renal blood flow doubles in the first month of life, and is comparable to adults by one to two years of age when corrected for body surface area. The maturational changes in renal vascular resistance are due to anatomical changes in the renal vasculature, as well as changes in the balance between vasoconstrictors, such as catecholamines and angiotensin II, and vasodilators, such as nitric oxide. The kidney develops in a centrifugal fashion. Juxtamedullary nephrons are formed before those in the superficial cortex. The renal blood flow distribution, measured by both xenon washout and injection of microspheres, shows a paucity of blood flow to the outer cortex compared to the deep cortex in neonates. During postnatal maturation there is a redistribution of blood flow, with enhanced perfusion of the outer cortex due to a decrease in renal vascular resistance.
Autoregulation
Renal blood flow (RBF) and glomerular filtration rate (GFR) remain stable over a wide range of perfusion pressures. As perfusion pressure falls there is vasodilatation of the afferent arteriole and vasoconstriction of the efferent arteriole which maintains RBF and GFR. As blood pressure increases during development, the range in pressure where autoregulation of renal blood flow and GFR occurs shifts accordingly. While neonates can autoregulate GFR in response to changes in blood pressure, this protective mechanism is far less developed than the autoregulatory capability of adults due, at least in part, to an attenuated release and efferent arteriolar response to angiotensin II.
Glomerular Filtration Rate
Initiation of glomerular filtration, as evidenced by the flow of urine, begins between nine and twelve weeks gestation in the human. The glomerular filtration rate (GFR) is lower in neonates than adults, even when corrected for body surface area. In premature human infants creatinine clearance increases as a function of postconceptual age (the sum of gestational age and postnatal age). GFR is constant at ~0.5 ml/min in infants with a postconceptional age of 28–34 weeks, despite the increase in renal size. GFR increases to 1.0 ml/min at 34–37 weeks and to 2 ml/min at a postconceptional age of 40 weeks. In absolute terms, the GFR increases 25-fold from birth to adulthood. Corrected for a surface area of 1.73 m 2 , the GFR in the human term neonate is 30 ml/min/1.73 m 2 in the first week of life. GFR continues to increase during the first ~1–2 years of life to reach adult levels when factored for body surface area ( Figure 27.1 ).

At birth, juxtamedullary glomeruli have a larger volume and greater single nephron GFR than superficial nephrons. In the guinea pig there is a 7-fold increase in GFR in the first month of life. The increase in total kidney GFR during the first week of life, a time when superficial nephron GFR is relatively constant, is predominantly due to an increase in juxtamedullary nephron GFR. After two weeks of age, however, the rise in total kidney GFR is predominantly due to an increase in GFR in superficial nephrons ( Figure 27.2 ).

The increase in single nephron GFR with postnatal maturation is due to a number of factors. Single nephron GFR is the product of the net ultrafiltration pressure and the glomerular ultrafiltration coefficient, K f . The effective ultrafiltration pressure is the difference between the hydrostatic and oncotic pressures across the glomerular capillary bed. Studies comparing newborn rats and guinea pigs to adults have shown a maturational increase in effective ultrafiltration pressure. However, these changes contribute at most 10% to the 20-fold increase in single nephron GFR. The maturational increase in GFR is predominantly the result of the increase in K f , which is the product of the hydraulic permeability of the glomerular capillary and the glomerular capillary surface area. Studies using neutral dextrans have found that the permeability characteristics change only slightly with maturation. The increase in K f , and thus single nephron GFR, is predominately due to the 7.5-fold increase in glomerular capillary surface area during renal maturation.
Sodium Chloride Transport
The transition from fetal to neonatal life is characterized by a dramatic decrease in urinary sodium excretion, despite an increase in GFR. The early fetus excretes ~20% of the filtered sodium, while the late gestation fetus excretes only ~0.2%. Term neonates are able to maintain a positive sodium balance over a wide range of sodium intake which is essential for growth. Compared to adults, neonates have a limited capacity to excrete an acute sodium load, and will develop volume expansion and hypernatremia with a sodium load. This phenomenon is exemplified in a study where adult and neonatal dogs were given an isotonic saline infusion equal in volume to 10% of the animal’s weight. The results of the cumulative excretion of sodium with time are shown in Figure 27.3 . Adult dogs had a brisk natriuresis and diuresis, excreting 50% of the sodium within two hours of the infusion. Dogs less than one week of age excreted less than 10% of the sodium infused by two hours. The limited ability to excrete a sodium load in neonates was not explained by a low GFR, since there was a comparable change with volume expansion in neonates and adults. Premature neonates have high urinary sodium losses and fractional excretion of sodium compared to term neonates. The following section discusses the maturation of tubular transport, which maintains the positive sodium balance in growing neonates.

Glomerulotubular Balance
Glomerulotubular balance remains fairly constant under a number of conditions which alter the GFR in the adult. During postnatal development, the maturational increase in the GFR is paralleled by a concomitant increase in the rate of tubule solute absorption. If this did not occur, there would be loss of essential solutes which would jeopardize the life of the neonate as GFR increases during development.
In the fetus, however, the GFR and delivery of solutes and water to the tubules can surpass the reabsorptive capacity of the tubules. In a clearance study examining the fractional reabsorption of volume and sodium after salt-loading in fetal, young, and adult guinea pigs, the fractional reabsorption of volume and sodium were lower in the fetus and in one-day-old animals. By 2–5 days of age, the fractional reabsorption of sodium and water were at the adult level. The glomerular–tubular imbalance is not only present in the fetus, but can also be manifest in the premature neonate. This is exemplified by the fact that glucosuria is frequently present in premature human neonates born before 30 weeks of gestation. In human neonates born before 34 weeks gestation, 93% of the filtered glucose is reabsorbed, which is comparable to the fractional reabsorption in the guinea pig fetus. By 34 weeks of gestation, the human neonate can reabsorb over 99% of the filtered glucose load.
Maturation of Proximal Tubule Volume Reabsorption
The developing kidney exhibits a centrifugal pattern of nephron maturation, with juxtamedullary nephrons being formed before superficial nephrons. The glomerular and tubular morphology of juxtamedullary nephrons are more mature than those in the superficial cortex. In many species, including the mouse, rat, and rabbit, nephrogenesis continues after birth in the superficial cortex. Nephrogenesis also continues postnatally in humans born prior to 34 weeks gestation.
The reabsorptive capacity of the neonatal superficial and juxtamedullary proximal tubule is less than that of adults. The rate of volume absorption in superficial proximal convoluted tubules increases two-fold between a 22–24-day-old weanling and a 40–45-day-old adult rat. In rabbit superficial proximal tubules the rate of volume absorption increased four-fold between one week and one month of age, while the rate of volume absorption in rabbit juxtamedullary proximal convoluted tubules did not change appreciably during that time. However, there is a two-fold increase in volume absorption in juxtamedullary nephrons between 4 and 6 weeks of age in the rabbit. Proximal tubule transport for each solute is the sum of transport mediated by passive diffusion, solvent drag, and active transport, which are discussed below.
Na + /K + -ATPase Activity
The basolateral Na + /K + -ATPase provides the driving force for all sodium-dependent transport along the nephron by generating and maintaining a low intracellular sodium concentration. Inhibition of Na + /K + -ATPase with ouabain reduces the rate of volume absorption to zero in neonatal and adult isolated rabbit proximal convoluted tubules. Thus, maturation of Na + /K + -ATPase activity plays a key role in the maturation of solute transport along the nephron.
The Na + /K + -ATPase is made up of an α-subunit and a regulatory ß-subunit. There are four α isoforms and three ß isoforms. The α-subunit is the catalytic subunit, and has an ATP-binding site as well as the binding site for cardiac glycosides. The adult kidney predominantly expresses the α1 and ß1 isoforms. There is a postnatal increase in renal Na + /K + -ATPase activity. In dissected rabbit tubules, the neonatal Na + /K + -ATPase activity is lower than the adult in each nephron segment examined ( Figure 27.4 ). The V max Na + /K + -ATPase activity increases five-fold during renal maturation, with no change in the K m for sodium, potassium or ATP.

The increase in Na + /K + -ATPase activity in the rabbit juxtamedullary proximal convoluted tubule lags behind the maturational increase in bicarbonate and volume absorption by one week, consistent with the maturational increase in apical membrane transport being the driving force for the increase in basolateral Na + /K + -ATPase. Several studies have supported this hypothesis. In rat proximal tubule cells in culture, stimulation of the Na + /H + exchanger increases intracellular sodium and leads to an increase in Na + /K + -ATPase activity. An increase in intracellular sodium not only increases pump activity, but also increases α and ß Na + /K + -ATPase subunit mRNA and protein abundance. Chronic increases in Na + /H + exchanger activity in rats induced by metabolic acidosis result in an increase in Na + /K + -ATPase activity in growing but not adult rats, an effect not seen when the rats were administered amiloride, an inhibitor of the Na + /H + exchanger. Thus, the maturational increase in Na + /H + exchanger activity may be responsible for the increase in Na + /K + -ATPase activity.
Maturation of Proximal Tubule NaCl Transport
In the early proximal tubule there is preferential reabsorption of bicarbonate over chloride ions. This leaves the proximal tubule luminal fluid with a higher chloride concentration and a lower bicarbonate concentration than the peritubular fluid. Chloride transport is the sum of active transcellular reabsorption and chloride diffusion across the paracellular pathway down its concentration gradient. In the adult rabbit proximal convoluted tubule, two-thirds of NaCl transport is active and transcellular and one-third is passive and paracellular. In the adult rat proximal convoluted tubule one-third of NaCl transport is active, and two-thirds is mediated by passive diffusion.
Active chloride transport by the proximal convoluted tubule is mediated by the parallel operation of the Na + /H + and Cl − /base exchangers. Cl − /OH − , Cl − /oxalate, and Cl − /formate exchange have all been found to mediate chloride uptake into the proximal tubule ; however, the relative importance of these transporters remains controversial. In the rabbit superficial convoluted tubule there is evidence for both Cl − /OH − and Cl − /formate exchangers. However, in juxtamedullary proximal convoluted tubules only Cl − /OH − exchange is present. In the guinea pig there is a large increase in Na + /H + exchanger activity and Cl − /formate exchange in brush border membrane vesicles during the transition from fetus to newborn. In isolated perfused rabbit proximal straight tubules the rate of active NaCl transport was two-fold greater in adult proximal straight tubules compared to neonatal tubules. Both Cl − /OH − and Na + /H + exchange activities were five-fold less in neonatal tubules compared to that in adult tubules.
The relative contribution of passive chloride transport to total NaCl transport may be species-specific. In the adult rat the late proximal tubular chloride concentration is significantly higher than that in the glomerular ultrafiltrate. However, in the neonatal rat, the proximal tubule luminal chloride concentration remains the same as that in the glomerular ultrafiltrate. The constant luminal chloride concentration could be due to equal rates of chloride and bicarbonate reabsorption by the immature segment or to a higher chloride permeability in the neonate mediating higher rates of passive chloride transport than in adults. Direct measurements of chloride permeability and the relative contributions of passive chloride transport have not been examined in vivo . Thus, the reason for the lack of the chloride gradient in neonatal rat proximal tubules is unknown.
Developmental Changes in the Paracellular Transport
Direct measurements of rabbit proximal convoluted tubule chloride and bicarbonate permeabilities demonstrate that neonatal segments have a lower permeability to both solutes than in the adult. In addition, the resistance of the proximal tubule is higher in the neonatal rabbit proximal tubule than the adult, providing further evidence that there are developmental changes in the paracellular pathway. Finally, studies have demonstrated that solvent drag does not contribute significantly to volume absorption in the neonatal tubule, as the reflection coefficients for both NaCl and NaHCO 3 were not different from one. Passive chloride transport has been characterized in the rabbit using in vitro microperfusion. In both neonates and adults chloride permeability is extremely low, indicating that passive chloride transport does not contribute significantly to chloride absorption in this nephron segment. The permeability of chloride in neonatal juxtamedullary proximal convoluted tubules is more than 10-fold lower than that of the adult segment. In adult rabbit proximal straight tubules there is a substantive rate of passive chloride transport ; however, the neonatal rabbit proximal straight tubule is impermeable to chloride. Thus, there is no passive NaCl transport in the neonatal proximal straight tubule.
The maturational changes in passive paracellular transport indicate there must be a developmental change in the composition of tight junction proteins. The tight junction creates a barrier and mediates the paracellular properties of the paracellular pathway. Occludin, a protein with a ubiquitous distribution, and claudins, a family of tight junction proteins that number over 20, have four membrane spanning domains forming two extracellular loops that adjoin with their partner on the neighboring cell. The permeability properties, including the ion permeability and resistance of epithelia, are determined predominantly by the isoforms of the claudins in the tight junction. Using semiquantitative real-time PCR of individually dissected tubules, there was no difference in the mRNA expression of claudins 1, 2, 10a, 12, and occludin in neonates compared to adult proximal tubules. However, claudins 6 and 9 were present in neonatal proximal tubules, but were absent in the adult segment. Expression of claudins 6 and 9 in Madin–Darby canine kidney II (MDCK II) cells, which have a low resistance and do not express these claudin isoforms, resulted in an increase in the paracellular resistance and increased sodium-to-chloride and bicarbonate-to-chloride permeability ratios. In addition, expression of claudin 6 and claudin 9 in MDCK II cells resulted in a decrease in the transepithelial chloride permeability. Thus, the expression of claudin 6 and 9 in neonatal proximal tubule is likely the cause for the higher resistance and the lower chloride permeability than in the adult tubule.
Distal Tubule NaCl Transport
The thick ascending limb and distal convoluted tubule reabsorb NaCl, and are impermeable to water. The osmolality of the luminal fluid collected by micropuncture of rat early distal tubules is 40% lower in adults compared to neonates, and the fraction of filtered sodium remaining in the early distal tubule is higher in neonatal than adult rats, consistent with a maturational increase in the rate of sodium transport in the thick ascending limb. In vivo micropuncture of 13- to 39-day-old rats showed an increase in loop NaCl reabsorption during postnatal maturation, and in vitro microperfusion of the rabbit cortical thick ascending limb demonstrated that the rate of sodium transport in the adult segment is five-fold greater than that of the neonate. Both rat and rabbit cortical and medullary thick ascending limb Na + /K + -ATPase activity increase approximately four-fold during postnatal maturation, consistent with a large developmental increase in sodium reabsorption by both segments. A direct comparison of NaCl transport in neonatal and adult medullary thick ascending limb has not been performed; however, there is a maturational increase in Na + /K + -ATPase activity in this segment.
Compared to adults, neonates have a limited capacity to excrete a sodium load which is not due solely to a lower GFR. None of the nephron segments discussed thus far is responsible for this phenomenon, as this requires a segment where the relative transport rates are higher in neonates than in adults. The adult distal convoluted tubule reabsorbs only 5% of the filtered sodium, but sodium transport rates are higher in neonates. In an in vivo micropuncture study, distal tubule transport was assayed during hydropenia and during volume expansion in 24- and 40-day-old rats. While 24-day-old hydropenic rats had a higher fraction of filtered sodium remaining in the early distal tubule than 40-day-old animals, this difference had disappeared by the late distal tubule puncture site. With volume expansion there was a comparable fraction of filtered sodium remaining in the early distal tubule in the two age groups, but there was enhanced late distal tubule sodium reabsorption in the younger rats. Thus this segment is, at least in part, responsible for the blunted natriuresis with salt-loading in neonates.
The cortical collecting duct is the nephron segment responsible for the final modulation of sodium transport under the control of aldosterone. In the isolated perfused cortical collecting tubule, there is a three- to five-fold increase in sodium transport between one-week-old and adult rabbits. Most of the maturational increase occurs between the first and second weeks of life. Sodium entry in the neonatal and adult cortical collecting tubule is via an apical sodium channel termed ENaC. ENaC is the rate-limiting step in collecting tubule sodium transport, and neonatal cortical collecting ducts have fewer apical conducting sodium channels than adult segments ( Figure 27.5 ). ENaC is composed of α-, ß-, and γ- subunits, and the mRNA and protein abundance of each increases during postnatal life. The driving force for conductive sodium entry across the apical conductance is the basolateral Na + /K + -ATPase, which increases in activity two-fold during maturation in this segment. Interestingly, the fetal collecting duct expresses the Na + /K + -ATPase on the apical membrane; the significance of this is unknown.

Regulation of Sodium Transport
Renin–Angiotensin–Aldosterone
Plasma renin activity is higher in the neonate than the adult, and increases appropriately to a reduction in extracellular fluid volume in the fetus, premature infant, and neonate. Plasma aldosterone levels are also significantly higher in preterm and term neonates than in adults. Cord blood from term infants born to mothers who ingested a normal salt diet had significantly lower plasma aldosterone levels than those whose mothers ingested a low-salt diet or who were taking diuretics. These data imply that the human fetus can also respond to volume contraction with an increase in serum aldosterone. The plasma aldosterone level has also been shown to vary inversely with sodium intake in preterm infants with respiratory distress syndrome. Although the newborn can increase aldosterone secretion, the effect of aldosterone to increase distal tubule sodium reabsorption and potassium secretion is blunted compared to adults. For example, in adult rats, adrenalectomy produces a 40-fold increase in urinary Na/K ratio, but in neonatal rats adrenalectomy has no effect on urinary electrolyte excretion. Administration of exogenous aldosterone to adult adrenalectomized rats decreases the urinary Na/K ratio profoundly, but administration of aldosterone has no effect on urinary electrolytes in adrenalectomized neonatal rats. Isolated perfused cortical collecting tubules from adult rabbits treated with mineralocorticoid had a significant increase in sodium absorption compared to control rabbits, but neonatal cortical collecting tubules were unaffected by prior mineralocorticoid treatment. This resistance to aldosterone in neonatal rats is not due to a paucity of mineralocorticoid receptors, but is a postreceptor phenomenon. However, in the mouse the blunted effect of aldosterone may also be due to fewer mineralocorticoid receptors compared to adults.
Angiotensin II augments proximal and distal tubule sodium reabsorption by binding to both basolateral and luminal receptors. While neonatal rats have a blunted diuresis and natriuresis in response to volume expansion, this was not the case in neonates which received Losartan, an AT I receptor blocker, consistent with angiotensin II playing an important role in the regulation of sodium absorption in the neonate.
Renal Nerves and Catecholamines
Renal nerve stimulation produces an increase in renal vascular resistance and reduction in renal blood flow in the fetus and newborn, although the effect is less in immature animals compared to adults. Renal denervation of the fetal and neonatal lamb does not significantly alter renal blood flow, GFR or renal sodium handling, indicating that basal renal function is not significantly affected by renal nerves. However, in the transition from fetal to neonatal life, renal nerves play a role in the decrease in sodium excretion that occurs during this period. Sheep with denervated kidneys had a greater urine volume and sodium excretion in the first 24 hours after birth, as well as lower plasma atrial natriuretic peptide and renin levels. There is no difference in urinary sodium or volume excretion after the first day of life, indicating that other unknown variables play an important role in mediating the profound decrease in urinary sodium and volume excretion after birth.
Dopamine is a natriuretic hormone whose plasma levels are increased with volume expansion. Dopamine increases renal blood flow and GFR, and inhibits sodium transport in the proximal tubule, thick ascending limb, and collecting duct. The concentration of dopamine is higher in fetal rat plasma and amniotic fluid than in the maternal blood. Administration of exogenous dopamine to human premature neonates with respiratory distress syndrome increases GFR, and produces a natriuresis and diuresis. However, comparative studies demonstrate that the effect of dopamine on the Na + /H + exchanger and Na + /K + -ATPase is less in neonatal than adult tubules.
Renal Acidification
The serum bicarbonate concentration in human infants in the first year of life averages 22 mEq/l, a value significantly less than that of adults. Premature human neonates can have serum bicarbonate levels as low as 14.5 mEq/l. The lower serum bicarbonate concentration in neonates and infants is due to a lower threshold for bicarbonate by the immature kidney. This section will discuss the salient differences between the neonatal and adult kidney with regard to renal acidification.
Proximal Tubules
The adult proximal tubule reabsorbs 80% of the filtered bicarbonate. The lower bicarbonate threshold in neonates is, in large part, due to a lower bicarbonate reabsorptive capacity of the neonatal proximal tubule. In rabbit juxtamedullary proximal convoluted tubules perfused in vitro , the rate of bicarbonate reabsorption in proximal tubules from one-week-old rabbits is one-third that of the adult proximal tubule. The rate of bicarbonate absorption does not change significantly until the time of weaning at four weeks of age. At six weeks of age, the rate of bicarbonate absorption is comparable to the adult. The maturational pattern of glucose and total volume reabsorption is quite similar to that for bicarbonate absorption. The rate of bicarbonate absorption has not been directly measured in superficial nephrons. However, micropuncture studies in rats and in vitro microperfusion studies in rabbits have demonstrated a similar maturational increase in the rate of volume absorption in these segments. Since a substantial fraction of volume absorption reflects bicarbonate absorption, a similar maturational pattern for bicarbonate is likely.
There are several potential explanations for the lower rate of bicarbonate transport in neonatal proximal tubules. In the adult proximal tubule there is a preferential reabsorption of bicarbonate over chloride ions. This leaves the luminal fluid with a higher chloride concentration and a lower bicarbonate concentration than that in the peritubular capillaries. This bicarbonate concentration difference could potentially allow bicarbonate to diffuse from the peritubular capillaries back to the luminal fluid. The amount of bicarbonate passive backflux is dependent upon the permeability of the paracellular pathway of the neonatal proximal tubule to bicarbonate. However, bicarbonate permeability in juxtamedullary rabbit proximal convoluted tubules is less in neonatal juxtamedullary proximal tubules perfused in vitro than that of the adult segment. Thus, the lower rate of bicarbonate transport in neonatal juxtamedullary proximal tubules is not explained by enhanced back diffusion, but is entirely due to a lower rate of active transcellular bicarbonate transport.
In the adult proximal tubule, two-thirds of apical proton secretion is mediated by the Na + /H + exchanger and one-third is via an H + -ATPase. The driving force for the Na + /H + exchanger is the low intracellular sodium concentration generated by the Na + /K + -ATPase. In the adult proximal tubule bicarbonate, exit is via the Na(HCO 3 ) 3 co-transporter. A lower rate of bicarbonate reabsorption by the neonatal proximal tubule could be due to a lower activity of any of these transport processes.
Studies using a variety of different techniques have demonstrated a maturational increase in proximal tubule apical membrane Na + /H + exchanger activity. In isolated perfused rabbit juxtamedullary proximal convoluted tubules the rate of Na + /H + exchanger activity is approximately one-third that of the adult rate, and adult values are reached at six weeks of age. These results compare well to the maturational changes in bicarbonate absorption measured in isolated perfused rabbit convoluted tubules. While this study solely examined juxtamedullary proximal tubules, similar findings have been demonstrated in brush border membrane vesicles from the renal cortex. Na + /H + exchanger activity in late rabbit fetal kidney cortex is 25% that of the adult cortex. This difference is entirely due to a lower V max , and not to a difference in the K M for sodium.
The H + -ATPase, present on the apical membrane of the adult proximal tubules, does not contribute significantly to bicarbonate absorption in the neonatal proximal tubule. To study the relative contribution of the Na + /H + exchanger and the H + -ATPase to proximal tubule acidification, the rates of these two transporters were assayed by measuring proton secretory rates in adult and neonatal proximal convoluted tubules in response to an intracellular acid load. As has previously been demonstrated, the rate of neonatal Na + /H + exchanger activity is less than that in the adult. In the adult rabbit proximal tubule two-thirds of the pH recovery from an acid load is due to the Na + /H + exchanger, and one-third is due to the the H + -ATPase. In the neonate, 95% of pH recovery is due to the Na + /H + exchanger, and only 5% is due to a sodium-independent mechanism. Thus, both the Na + /H + exchanger and the H + -ATPase undergo a significant increase in activity during maturation, and presumably limit bicarbonate absorption by the neonatal proximal tubule.
Three isoforms of the Na + /H + exchanger, NHE1, NHE3, and NHE8 have been localized to the proximal tubule. NHE1 has a ubiquitous distribution in tissues, and is found on the basolateral membrane of the proximal tubule. NHE1 protein abundance does not vary significantly with postnatal maturation. NHE3 is the apical membrane Na + /H + exchanger mediating most luminal proton secretion by the adult proximal tubule. There is a substantive postnatal maturational increase in NHE3 mRNA and protein abundance. Interestingly, neonates have Na + /H + exchanger activity at a time when NHE3 protein abundance is barely detectable. The disparity between the presence of Na + /H + exchanger activity and the paucity of NHE3 in the proximal tubule of neonates is due to the presence of NHE8. NHE8 is predominantly an intracellular sodium–hydrogen exchanger that is also abundantly expressed on the apical membrane in neonates. Brush border membrane NHE8 decreases in abundance during postnatal maturation ( Figure 27.6 ). The factors responsible for this isoform switch will be discussed below.

The basolateral membrane Na(HCO 3 ) 3 co-transporter facilitates bicarbonate exit from the proximal tubule. Na(HCO 3 ) 3 symporter activity in neonates is only slightly less than that of adults. This transporter not only plays a role in bicarbonate exit, but is also the predominate mechanism for the defense against changes in intracellular pH by the proximal tubule.
Distal Tubule Acidification
The adult cortical collecting duct can absorb or secrete bicarbonate depending on the acid–base status of the animal. Proton and bicarbonate secretion are mediated by α- and β-intercalated cells, respectively, which are far fewer in number than principal cells, the predominant cell in the cortical collecting duct. The number of α- and β-intercalated cells per millimeter of tubular length increases several-fold during maturation of the rabbit cortical collecting duct. β-intercalated cells start to appear in the mouse cortical collecting tubule in the first week of life. While β-intercalated cells are present in the outer medullary collecting duct of the neonate, they disappear by apoptosis and are no longer present in this segment by 2–3 weeks of age.
Net bicarbonate transport in the cortical collecting tubule is dependent on the relative rates of bicarbonate absorption and secretion. In isolated perfused cortical collecting tubules from neonatal rabbits there is no net bicarbonate transport, whereas adult cortical collecting ducts secrete bicarbonate. Bicarbonate secretion by the β-intercalated cell is via an apical chloride-bicarbonate exchanger that functions at a reduced rate in the neonatal segment. Removal of bath chloride inhibits basolateral membrane chloride-base exchange on α-intercalated cells and inhibits luminal proton secretion. Removal of bath chloride had no effect on the rate of bicarbonate transport in cortical collecting tubules from neonatal rabbits, but increased net bicarbonate secretion in adult segments, indicating that there is a maturational increase in the cortical collecting tubule to secrete bicarbonate. The bicarbonate absorptive capacity of the α-intercalated cell in the cortical collecting duct also increases during postnatal maturation. The outer medullary collecting duct is an important segment for urinary acidification. Unlike the cortical collecting tubule, the number of intercalated cells per millimeter of tubular length does not change significantly with postnatal development. The rate of bicarbonate absorption is only slightly less in the neonatal outer medullary collecting ducts compared to the adult rabbit segment. Thus, there is a significant difference in the relative maturity of the cortical and medullary segments in neonates.
Titratable Acid and Ammonia Excretion
In addition to reclaiming the filtered load of bicarbonate, the kidney must excrete an amount of acid equivalent to the acid generated from metabolism. The growing animal must also excrete protons liberated during the formation of bone. This is in part compensated for by the gastrointestinal absorption of alkali in the neonate, but as in the adult, the neonatal kidney plays a major role in acid excretion. Thus, the kidney of the growing neonate must excrete 50–100% more acid per kg than the adult.
To excrete the quantity of acid generated from metabolism of proteins and growing bones, there must be urinary buffers to accept the secreted protons. If there were no titratable acids and ammonia in our urine, the secretion of a few protons would decrease the urine pH to levels that would inhibit further proton secretion. Net acid excretion, the sum of ammonium and titratable acid excretion per kg body weight, is significantly less in neonates than adults. However, by seven days of age cows milk formula-fed infants have comparable rates of ammonium and titratable acid excretion as that of adults when normalized per kg of body weight. Breast milk-fed infants, however, have significantly less phosphate intake and lower rates of titratable acid excretion than infants fed cows milk formula. Thus, the rate of renal maturation of net acid excretion is quite brisk. However, unlike adults, human neonates function at near maximal capacity of net acid excretion to eliminate metabolically generated acid, and have a limited ability to respond to an exogenous acid-load by increasing titratable acid and ammonia excretion. In comparison to adults, neonates given an acid-load have a smaller increase in both titratable acid and ammonium excretion, making them more prone to develop acidosis when so challenged. By one month of age the rate of net acid excretion in response to an acid-load is comparable to that of adults. However, young infants respond to an acid-load with higher rates of titratable acid excretion to compensate for the lower rates of ammonium excretion. In vitro studies have shown that the rate of renal ammonia production in kidney slices is lower in neonates compared to adults. The low rate of ammonia production is in part due to lower glutamine synthetase activity limiting glutamine availability, but predominantly due to lower glutaminase activity. As in adults, glutaminase activity increases substantively in response to an acid-load. Premature neonates have significantly lower rates of titratable acid and ammonia excretion than term infants. Preterm infants are thus less tolerant of a high protein formula than term infants, because sulfur containing amino acids generate an acid-load which cannot be eliminated due to the low rates of net acid excretion. Administration of NH 4 Cl to premature infants resulted in an increase in net acid excretion, but premature infants had lower rates of ammonium and titratable acid excretion, and a higher urine pH, than term neonates. As with term infants, there is a rapid maturation of the ability of premature infants to excrete an exogenous acid-load.
Carbonic Anhydrase Activity
Carbonic anhydrase facilitates the reversible hydration of CO 2 to H 2 CO 3 . Carbonic anhydrase is localized to all acidifying nephron segments, where it plays an important role in acidification. For example, carbonic anhydrase inhibition results in a 90% decrease in the rate of proximal tubule bicarbonate reabsorption. There are 15 carbonic acid isoforms, with both species- and nephron segment-specificity.
Carbonic anhydrase II is located in the cytosol of all acidifying renal tubules, and comprises 95% of carbonic anhydrase activity in the kidney. Carbonic anhydrase IV comprises approximately 5% of total renal carbonic anhydrase activity, and is located on the apical and basolateral membrane of the proximal tubule and on the apical membrane of acid-secreting cells in the distal nephron. Carbonic anhydrase XII is present on the basolateral membrane of acidifying segments. In rodents, carbonic anhydrase XIV is also expressed on the apical membrane of the proximal tubule and thick ascending limb. Carbonic anhydrase II protein abundance, normalized per millimeter of tubular length, increases approximately 10-fold in rat proximal convoluted tubules, cortical collecting tubules, and outer medullary collecting tubules between one and twelve weeks of age. In the rabbit, carbonic anhydrase II increases only two-fold during postnatal maturation compared to carbonic anhydrase IV, which undergoes a ten-fold increase in mRNA and protein abundance with cortical maturation. Thus, the developmental increase in carbonic anhydrase may be a factor in the postnatal increase in renal acidification.
Induction of Nephron Maturation
As noted above, there are a number of quantitative and qualitative changes that occur in all nephron segments during postnatal development. Most of the studies examining the factors responsible for the postnatal changes during development come from studies of the proximal tubule, which will be the focus here. There are a number of potential factors which may be responsible for the postnatal maturational changes in proximal tubule transport. The maturational increase in GFR may induce the maturation of transporters on the apical membrane by increasing solute delivery. As previously discussed, an increase in apical membrane sodium transport could increase intracellular sodium and play a potential role in the maturational increase in Na + /K + -ATPase activity. There are also significant postnatal maturational changes in several hormones which affect proximal tubular transport, including thyroid hormone and glucocorticoids, which increase 3-fold and 20-fold, respectively, in the postnatal period.
Glucocorticoids increase renal cortical Na + /H + exchanger activity. This effect can, in part, be mediated by an increase in GFR which will increase sodium delivery to the proximal tubule. However, glucocorticoids and thyroid hormone have a direct epithelial action on the proximal tubule to increase the rate of bicarbonate absorption and Na + /H + exchanger activity. This effect of glucocorticoids and thyroid hormone on NHE3 is due to an increase in NHE3 transcription. However, glucocorticoids also increase apical membrane NHE3 by increasing the rate of exocytosis. Administration of glucocorticoids to pregnant rabbits two days prior to the delivery increases the V max of the Na + /H + exchanger in neonates to levels the same as those seen in adults. The K m for sodium is comparable in neonates and adults. Similarly, proximal convoluted tubules from neonatal rabbits whose mothers received dexamethasone before delivery had infants with an almost two-fold increase in the rate of bicarbonate absorption to levels comparable to that measured in adult animals. In addition, the rate of Na + /H + exchanger and Na(HCO 3 ) 3 activity in proximal tubules increased two-fold in dexamethasone treated neonates. The effect of dexamethasone is specific for the NHE3 isoform, the isoform present on the apical membrane of the proximal tubule and responsible for the majority of Na + /H + exchanger activity in adults. Prevention of the maturational increase of either glucocorticoids or thyroid hormone prevents the maturational increase in Na + /H + exchanger activity and NHE3 protein abundance. While glucocorticoids are the most important factor causing the maturational increase in NHE3, adrenalectomy in the neonatal period does not totally prevent an increase in both Na + /H + exchanger activity and NHE3 protein and mRNA abundance, suggesting that thyroid hormone can compensate, to some extent, in the absence of glucocorticoids. This was definitively demonstrated in studies using hypothyroid-glucocorticoid-deficient rats, where the maturational increase in both hormones was prevented, and where Na + /H + exchanger activity, NHE3 protein, and mRNA abundance remained at neonatal levels into adulthood. As noted, there is an isoform switch from NHE8 to NHE3 during postnatal development. The maturational decrease in NHE8 on the apical membrane of the proximal tubule is due the postnatal increase in thyroid hormone. Administration of thyroid hormone to neonates resulted in a premature decrease in NHE8 and increase in NHE3 on brush border membranes, while prevention of developmental increase in thyroid hormone prevented the decrease in brush border membrane NHE8 abundance. The regulation of NHE8 by thyroid hormone is due to a direct epithelial effect to decrease NHE8 surface expression, likely by post-transcriptional regulation.
As will be discussed, there is a maturational decrease in the rate of phosphate transport during postnatal maturation. This is observed at a time when there is an increase in serum glucocorticoid levels. Administration of glucocorticoids to neonatal rats produced a significant inhibition in the rate of sodium-dependent phosphate uptake. This is due to a reduction in the V max with no change in the K M for phosphate. This glucocorticoid-induced decrease in phosphate uptake was not accompanied by a change in NaPi-2a mRNA abundance; however, there was a three-fold decrease in NaP i transporter protein abundance. Thus, glucocorticoids play a role in the maturational decrease in phosphate transport.
Thyroid hormone appears to play an important role in postnatal development of mitochondrial enzymes in the rat proximal convoluted tubule. The normal maturational increase in the proximal tubule mitochondrial enzymes 3-keto acid-CoA transferase, an enzyme involved in ketone body oxidation, and citrate synthase and carnitine acetyltransferase were impaired in hypothyroid rats. The enzyme activities were restored with thyroid replacement. Acetoacetyl-CoA thiolase, however, is not decreased in 21-day-old hypothyroid rats. Finally, thyroid hormone plays a role in the maturational changes that occur in the permeability properties of the paracellular pathway. As noted above, the adult rabbit proximal straight tubule has a high chloride permeability which results in high rates of passive chloride transport. The neonatal rabbit proximal straight tubule is impermeable to chloride. Neonatal proximal straight tubules have higher P Na /P Cl (sodium to chloride permeability ratio) and P HCO3 /P Cl ratios than adult segments. Many, but not all, of the maturational changes in paracellular permeability properties of the proximal tubule can be accelerated by administration of thyroid hormone to neonates or prevented if the neonates are made hypothyroid.
Phosphate Transport
Phosphate is essential for growth, and unlike adults growing animals are in positive phosphate balance. Human neonates and infants have a higher serum phosphate concentration than that of adults. While the high serum phosphate in neonates could be due to the lower GFR compared to adults, this is not the case. Studies have demonstrated that increasing the GFR by arginine infusion in young rats does not increase phosphate excretion, and lowering the GFR in adult rats by constricting the abdominal aorta does not increase the rate of phosphate absorption to that seen in the neonate. The tubular reabsorptive capacity for phosphate is higher in neonates and infants than in adults. Approximately 90–95% of the filtered phosphate is reabsorbed in human neonates during the first week of life, and growing children continue to maintain a higher maximal tubular reabsorption of phosphate than adults.
A number of variables, such as intrinsic properties of phosphate transport in the proximal tubule, dietary phosphate content, parathyroid hormone, and growth factors can modulate phosphate transport, and could potentially explain the higher rate of phosphate transport by the neonatal kidney. The role of these factors has been extensively studied and will be reviewed in this section. A higher intrinsic rate of renal phosphate transport has been demonstrated in the isolated perfused guinea pig kidney where other in vivo factors were eliminated. The maximal tubular reabsorption of phosphate per volume of glomerular filtrate in 3–7-day-old neonatal guinea pig kidneys is 40% greater than that measured in adult animals. A higher intrinsic rate of proximal tubular phosphate reabsorption was also directly demonstrated in micropuncture studies, where 5–14 day neonatal guinea pigs reabsorbed a higher fraction of filtered phosphate than adults. The maximal rate of phosphate uptake in brush border membrane vesicles (V max ) is five-fold higher in neonates than in adults, in the absence of a maturational change in the K M for phosphate in growing animals.
In addition to the difference in the rate of sodium-dependent phosphate co-transport with maturation, other intrinsic proximal tubular factors may play a role in the higher rates of phosphate transport in growing animals. The intracellular phosphate concentration (P i ) measured in isolated perfused kidneys using NMR was 40% lower in growing animals than in adults. This provides a greater driving force for phosphate transport in growing animals. The lower intracellular phosphate concentration in the presence of a higher rate of apical phosphate transport implies that the rate of basolateral phosphate exit is also higher in growing animals, although this has not been directly examined.
Membrane fluidity also has been shown to affect phosphate transport. The brush border membrane content of cholesterol, sphingomyelin, and phosphatidylinositol increases with age. This change in lipid composition decreases membrane fluidity, which directly decreases the rate of phosphate transport. Thus, the lipid composition and high membrane fluidity of the neonate and growing animal provides an environment which increases the V max of the NaPi co-transporter. Glucocorticoids, which increase during the time of weaning, decrease membrane fluidity, and likely play a role in the postnatal decrease in phosphate transport.
There are two sodium-dependent phosphate co-transporters, designated as NaPi-2a and NaPi-2c, present on the apical membrane of the proximal tubule that are primarily responsible for phosphate reabsorption. In mice, NaPi-2a has been identified as the most important phosphate transporter in the kidney, responsible for ~70% of phosphate reabsorption. NaPi-2a mRNA is first detected in early proximal convolutions in the post S-shaped body segment of the developing nephron. However, NaPi-2a protein is not detected until the proximal tubules have a distinct brush border membrane. Brush border membrane NaPi-2a expression is two-fold higher in 4-week-old juvenile rats than in adult rats, consistent with the higher rate of phosphate transport in growing animals. A growth-specific NaPi co-transporter was postulated to be responsible for the increased phosphate transport in the weaning animals, which was later identified as NaPi-2c. NaPi-2c mRNA and protein expression is highest in the brush border of the convoluted proximal tubules of weanling rodents, whereas it plays a negligible role in renal phosphate absorption in adults. In contrast to rodents, NaPi-2c plays an important role in phosphate transport in humans. Mutations in NaPi-2c result in an autosomal recessive disorder termed hereditary hypophosphatemic rickets with hypercalciuria that is characterized by hypophosphatemia, due to renal phosphate-wasting, rickets, hypervitaminosis D, hypercalcemia, and hypercalciuria.
Dietary phosphate content regulates renal phosphate transport in adult and in growing animals. Thyroparathyroidectomized growing rats had a greater increase in their maximal tubular capacity for phosphate reabsorption in response to a low-phosphate diet and a blunted decrease in phosphate reabsorption on a high-phosphate diet compared to adult thyroparathyroidectomized rats. In addition, the adaptive increase in maximal tubular capacity of phosphate reabsorption in response to phosphate deprivation took longer to occur in adult animals than in growing animals. A low phosphate diet increases the rate of sodium-dependent phosphate uptake by brush border membrane vesicles in growing rats. In addition, ingestion of a low-phosphate diet increases the abundance of Na-Pi transporters on the apical membrane of the proximal tubule, whereas a high-phosphate diet produces the opposite effect. The dietary changes in phosphorus affect both brush border membrane NaPi-2a and NaPi-2c expression.
The plasma concentration of PTH is lower in human neonates than in adults. Fetal and neonatal rats can respond to changes in serum calcium with appropriate changes in PTH levels, although there is a blunted response to PTH secretion. Infusion of PTH in one-day-old human neonates produces no increase in urinary phosphate excretion. By three days of age, infusion of parathyroid hormone results in an increase in phosphate excretion and increase in urinary cAMP. However, the phosphaturic response to PTH is markedly lower in neonates than adults. Furthermore, in micropuncture studies, the proximal tubule response to infusion of pharmacologic doses of PTH is attenuated in young rats compared to adults. Despite the fact that PTH did not significantly increase phosphate excretion in young animals, the urinary cAMP corrected for GFR in response to PTH infusion was similar in all ages. Thus, there is clearly a disassociation between the PTH-induced increase in cAMP production in neonates and the effect on phosphate excretion. The blunted response to PTH in the neonate compared to the adult is due to lower levels of phospholipase A2 activity in response to PTH and cAMP.
Growth hormone administration can result in an increase in serum phosphate. Brush border membrane vesicle phosphate transport is increased in dogs that received growth hormone compared to controls. The effect of growth hormone on phosphate transport is mediated by IGF-1. While growth hormone is not a significant regulator of phosphate transport in the adult, this may not be the case in the growing animal. Administration of growth hormone-releasing factor antagonist, which suppresses growth hormone secretion, has no effect on the maximal rate of phosphate absorption in adult rats, but significantly reduces phosphate absorption in growing rats.
In addition to PTH, there are a number of hormones, known as phosphatonins, that regulate phosphate transport, including fibroblast growth factor-23 (FGF23), secreted frizzled-related protein 4 (sFRP-4), matrix extracellular phosphoglycoprotein, fibroblast growth factor 7 (FGF7), and dentin matrix protein-1(DMP1). These hormones are dysregulated in a number of diseases with aberrant phosphate regulation, incuding X-linked hypophosphatemia and tumor-induced osteomalacia. The role of these phosphatonins in the developmental changes in phosphate transport remains to be elucidated.
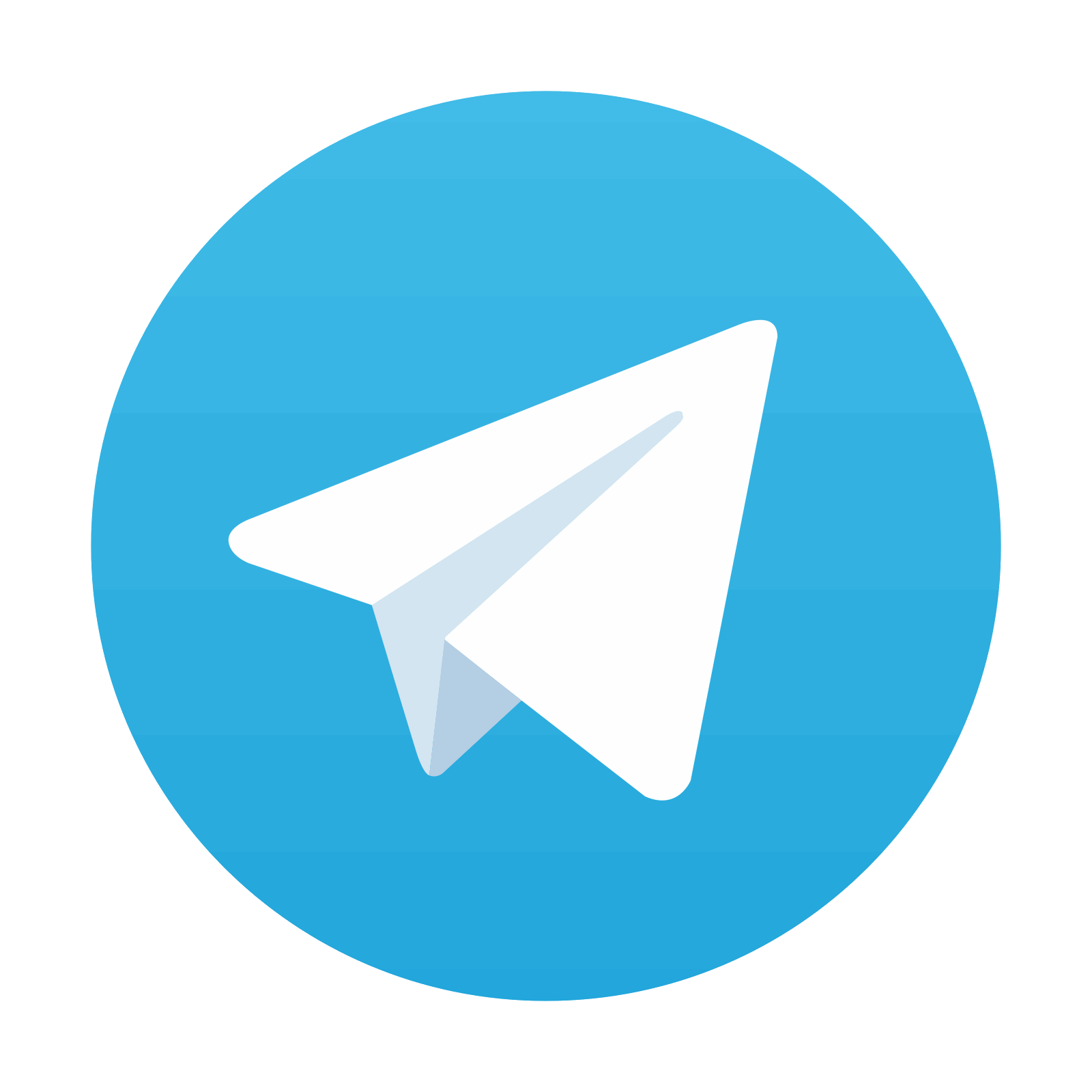
Stay updated, free articles. Join our Telegram channel

Full access? Get Clinical Tree
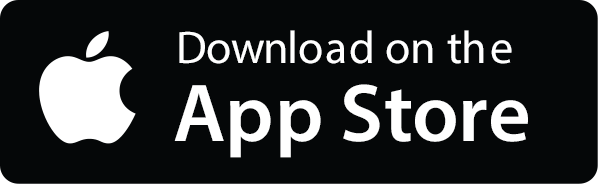
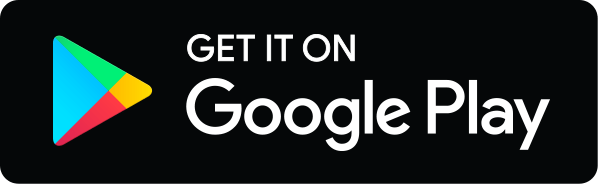