Parenteral nutrition (PN) is the intravenous administration of nutrients necessary for the maintenance of life. The nutrient components of PN include dextrose, amino acids, fat, electrolytes, multivitamins, and trace elements. Clinicians who are caring for infants and children should pay close attention to the changing nutrient requirements with age; specialized needs of children; vascular access; and the sometimes-limited ability of infants, children, and the critically ill to handle large amounts of fluid, protein, fat, and carbohydrates. In the 1960s, Dudrick et al. showed that beagle puppies and subsequently an infant could be successfully nourished with the use of PN solutions and central venous access. Since that time, research has promoted substantial advancement in the fields of intravenous access and PN solution components, as well as an improved understanding of the needs of patients with various illnesses to provide individualized care across the spectrum of life. Normal growth and development have been shown in patients exclusively fed by PN.
Indications
PN may be used as primary, adjunctive, or supportive therapy. The enteral route is the preferred choice when the clinician is deciding how to provide nourishment for any patient. It is only when a patient cannot receive adequate nutrition enterally for an extended period that the parenteral route should be used. When the patient’s tolerance for enteral feeds improves, every effort should be made to start and advance the delivery of enteral nutrition. PN is therefore used in patients who cannot be fed enterally for five or more days and should be used to support the patient until recovery from the underlying condition has occurred. In the very low-birth-weight (VLBW) or malnourished infant with limited nutritional reserves, PN should begin on the first day of life with a protein- and glucose-containing solution. Appropriate indications for initiating PN include compromised gut integrity (resection, high-output fistulas, complete obstruction, paralytic ileus, or ischemia), malabsorption, severe short bowel syndrome, intractable vomiting or diarrhea, or inability to obtain enteral access ( Table 88-1 ). PN is especially important in the patient with preexisting malnutrition or with chronic disease. It may be used to supplement enteral intake in patients who have increased needs (e.g., patients with chronic diarrhea, malabsorption, short bowel syndrome, or cystic fibrosis) or those who are unable to tolerate adequate enteral feeds to support themselves nutritionally. Most patients with 25 cm of small bowel and an ileocecal valve can ultimately tolerate enteral feeds and discontinue PN. A 2008 retrospective study demonstrated that residual intestinal length greater than 40 cm was significantly associated with higher survival, whereas presence of an ileocecal valve was not associated with survival. Data from the International Serial Transverse Enteroplasty (STEP) Data Registry demonstrated that longer pre-step intestinal length was associated with increased likelihood of achieving enteral autonomy and decreased risk of progression to transplantation nor death. The use of supplemental PN should also be considered when slow advancement of enteral feeds is anticipated. In addition to a supportive role, PN may be used to treat an underlying condition (e.g., chylothorax).
Primary | Gut failure, necrotizing enterocolitis, severe motility disorders, inability to obtain enteral access |
Supportive | Postoperative patients, burns, liver failure, renal failure, severe viral gastroenteritis, oncology and bone marrow transplant recipients, inflammatory bowel disease, trauma |
Supplemental | Nutritional failure, feeding intolerance |
Route of Administration
PN can be administered via a central or peripheral venous route depending on the available access and the composition of the PN solution. Peripheral PN is generally used for patients whose anticipated period of inadequate enteral feedings is less than 1 week and who have normal fluid requirements. It is often difficult to maintain peripheral access for longer than 1 to 2 weeks or to deliver adequate calories with solutions containing 10% or 12.5% dextrose. When hyperosmolar solutions with dextrose concentrations higher than 12.5% are administered through a peripheral intravenous line, there is a risk of phlebitis and thrombosis. Although some variance exists across institutions, limiting the infusion of a peripheral solution to a maximum osmolarity of 850 to 1000 mmol is a typical standard of practice. Solutions with high dextrose (more than 12.5% dextrose) and calcium concentrations may be associated with increased risk for phlebitis. The concomitant administration of intravenous fat may help decrease phlebitis in a peripheral vein. Depending on the size of the vein, it may not be possible to infuse large volumes or administer PN at a high rate.
The central venous route is used for the administration of large fluid volumes at high infusion rates, for hypertonic solutions, and for prolonged administration of PN solutions (more than 2 weeks). PN may be administered via a peripherally inserted central catheter (PICC), a tunneled central venous catheter (CVC, e.g., Broviac), or an implantable port (e.g., portacath). It is recommended that the tip of the catheter be placed at the junction of the superior vena cava and right atrium. The best place for a line associated with the least amount of complications is the right internal jugular vein, with the tip of the catheter high in the superior vena cava. This position, corresponding to the level of T6, is at the level of the right mainstem bronchus and the junction of the right atrium and superior vena cava. When femoral lines or umbilical venous catheters are used, the tip of the catheter should be placed superior the level of the diaphragm in the inferior vena cava. Although these two specific catheters are adequate for central infusion, both are associated with increased risk of infection because of contamination at the exit site. Malpositioned catheters, such as those at the level of the renal vessels and those in the liver, should not be used for PN administration because of the risk of thrombosis. It is not recommended that PN be administered via umbilical arterial catheters because of the risk of sepsis. The incidence of peripheral venous access and thrombosis has been well described. Measures to prevent thrombosis of vessels include making sure the vessel is not traumatized during insertion of the catheter, using correct tip placement, avoiding the subclavian and femoral veins, always using the smallest catheter possible, using the smallest vein possible, and using an appropriately sized catheter for the vein. Other measures for preventing thrombosis include administering PN into a larger vessel, using ultrasound guidance at the time of insertion, removing the CVC as soon as possible, treating CVC blockages early, preventing and treating all infections and venous occlusions, reusing previous access sites for CVC placement, and not placing the CVC into a fibrin sheath. In a patient with poor access, the following sites for placing a CVC may need to be considered: translumbar inferior vena cava, recannulation of the central vein, transhepatic intravenous catheter, use of collateral veins, azygous and hemi-azygous veins, intercostal veins, and placement of the line directly into the right atrium. Long lines or PICC lines are threaded into the heart through a large peripheral vein such as the basilic vein or cephalic vein. The success of placement is dependent on the patency of the vein selected, the presence of valves, and the experience of the person placing the line. PICC lines have been used for more than 20 years and are popular because there are few limitations regarding their use related to age, gender, or diagnosis. There is a low incidence of complications with PICC lines—less than 1% for infection, central vein thrombosis, and catheter malposition—as long as their use is limited to 6 to 8 weeks. In addition, significant cost savings are associated with the use of this type of catheter. Contraindications to the placement of PICC lines include dermatitis, cellulitis, burns at or near the insertion site, and previous ipsilateral venous thrombosis.
Patients receiving long-term PN (i.e. greater than 6 weeks) will benefit from the placement of a permanent CVC or tunneled silicone elastomer (Silastic) catheter (e.g., Broviac, Hickman, or Groshong) and subcutaneous portacaths. A surgeon or an interventional radiologist generally places these catheters using general anesthesia or conscious sedation. Tunneled catheters, which can be placed either via a cutdown or percutaneously, have a Dacron cuff located on the midportion of the catheter. This cuff stimulates the formation of dense fibrous adhesions, which anchor the catheter subcutaneously to prevent dislodgment. The cuff also acts as a barrier to bacteria migrating subcutaneously along the catheter surface. Sutures are needed to anchor the catheter at the exit site for several weeks after insertion to allow time for the formation of fibrous adhesions to the Dacron cuff.
Implantable ports are made of plastic or titanium with a compressed silicon disk designed for 1000 to 2000 insertions with a noncoring needle. They are inserted percutaneously into the jugular, subclavian, or cephalic vein and placed in a subcutaneous pocket over the upper chest wall. There are smaller ports available that are primarily used for arm placement and for children. These ports are generally used in situations in which the catheter is only periodically accessed. Patients at high risk for catheter-related thrombosis include those with cancer, those with infections, and those receiving chemotherapeutic agents or PN.
PN can also be delivered via peritoneal or hemodialysis catheters. Intradialytic PN (IDPN) is the administration of PN during dialysis and has been shown to be useful in patients with end-stage renal disease who do not respond to oral nutritional intervention. IDPN has been shown to be safe and beneficial in smaller studies of patients with protein energy malnutrition. PN can also be administered to patients who are receiving extracorporeal membrane oxygenation (ECMO). Neonates on ECMO are particularly susceptible to the effects of catabolic stress and require careful nutritional support. The dextrose/amino acid solution is administered through the ECMO circuit and intravenous fat is administered through a peripheral line to avoid occlusion of the ECMO circuit.
Parenteral Nutrition Components and Requirements
Energy
A patient’s enteral requirements are 5% to 10% higher than their parenteral requirements to account for the thermic effect of food and for the loss of some nutrients in the stool during the process of digestion and absorption. There are several ways to estimate energy requirements: the World Health Organization (WHO) equation, Dietary Reference Intakes (DRIs), and prediction equations such as the Schofield height-weight equation and those developed by Duro et al. and Pierro et al. Demonstration of adequate weight gain in the absence of edema and normalization of certain nutritional markers (e.g., prealbumin) is the best way to determine if a patient’s energy requirements have been accurately assessed. This response is sometimes difficult to assess in critically ill patients, in patients with edema or renal failure, or in patients receiving corticosteroids. It is also unclear whether one should expect normal rates of growth in the critically ill patient or just provide enough energy to prevent catabolism. There is concern about energy requirements in critically ill patients on PN. A study by Mehta et al. documents the occurrence of under- or overfeeding in the critically ill population as the result of using predictive equations to determine energy needs. The dynamic changes in energy needs of critically ill children are not well captured in estimation equations, and these children are particularly susceptible to poor nutrition. Indirect calorimetry (IC) measures oxygen consumption and carbon dioxide production during respiratory gas exchange, providing the most accurate measurement of resting energy expenditure (REE). IC is increasingly available and is often helpful for determining the caloric requirements of patients who are nutritionally at risk such as infants and children with failure to thrive, those who are dependent on PN or enteral tube feeds, obese patients, and critically ill patients. It was previously thought that measurement of mechanically ventilated patients was not accurately calculated by IC; however, current literature supports the use of IC with this population to prevent energy imbalance.
Infants and children have higher per-kilogram caloric requirements compared with adults, because of growth and development requirements. For infants up to 1 year of age, a per-kilogram caloric requirement that decreases with age is used. This is based on their energy requirements and the need for catch-up growth. We use the WHO equation for determining the needs of children older than l year of age. This equation, which provides an estimate of REE, is based on data from several thousand children and has been found to be accurate in children older than 1 year of age. Total energy needs may then be determined by multiplying the REE by a factor determined by the severity of underlying disease, the activity level of the patient, and the need for catch-up growth ( Tables 88-2 and 88-3 ).
Age (Years) | Male | Female |
---|---|---|
1-3 | 60.9 wt − 54 | 61.0 wt − 51 |
3-10 | 22.7 wt + 495 | 22.5 wt + 499 |
10-18 | 17.5 wt + 651 | 12.2 wt + 746 |
18-30 | 15.3 wt + 679 | 14.7 wt + 496 |
* Estimated daily energy requirements = REE × disease activity/stress factor.
Stress Factor | Description |
---|---|
1.1-1.3 | Well-nourished child at rest with mild to moderate stress or after minor surgery |
1.3-1.5 | Normal active child with mild-to-moderate stress, inactive child with severe stress (trauma, cancer, extensive surgery), or malnourished child requiring catch-up growth or with severe stress |
1.5-1.7 | Active child requiring catch-up growth or with severe stress |
* Estimated daily energy requirements = REE × disease activity/stress factor.
The energy needs of obese patients are best determined by the Schofield height-weight equation. Caloric needs and energy intake may be increased in patients with head injury or sepsis. Paralyzed patients on a ventilator will have no physical activity and thus have lower energy needs. Often a “stressed” patient will be given additional calories, but it is not clear whether this practice should be endorsed. Various studies have shown that the increased demands of illness are often counterbalanced by decreased physical activity, which keeps total energy expenditure the same whether the patient is well or sick. Although a major concern is to provide adequate energy to prevent catabolism, it is equally important to not overfeed the patient. Providing energy in excess increases the risk of complications including hyperglycemia, azotemia, immunosuppression, and hepatic steatosis. Some research in adults suggests the approach of permissive underfeeding to prevent complications from overfeeding in the obese population. A large body of evidence supports the use of a reduced energy PN formulation to provide nitrogen balance similar to that of a eucaloric regimen, without the negative effects just noted. A retrospective study of children with burn injuries demonstrated the ability of hypocaloric, high-protein PN to facilitate wound healing while minimizing complications. Longitudinal studies in the pediatric population will be important to determine if hypocaloric-feeding regimens can maintain adequate nitrogen balance while promoting growth.
It is necessary to provide not only calories but also protein in adequate amounts so that the patient is in positive nitrogen balance; otherwise, the patient will utilize protein for energy.
Protein
The protein source in PN is provided by crystalline amino acids, which provides approximately 4 kcal/g of protein. Protein usually provides 10% to 20% of total PN calorie needs. Since the advent of these purer forms of amino acids, the incidence of hyperammonemia and metabolic acidosis has been rare. Also demonstrated is that nitrogen retention is better with amino acid formulations when compared with protein hydrolysates. A variety of protein solutions are available for use in children and adults and in patients with hepatic disease, renal disease, and metabolic disease. An example of a protein solution used in a metabolic disorder is the parenteral protein solution designed for use in methylmalonic acidemia. In this instance, the amounts of certain amino acids can be dosed according to daily blood amino acid levels. The compositions of protein solutions for infants, including preterm, are different from those used in children and adults. Infant amino acid formulations, such as TrophAmine (B. Braun), provide essential amino acids such as cysteine, histidine, and tyrosine, which are not found in adult formulations. TrophAmine also contains taurine, which is important for brain and retinal growth; deficiency of this amino acid is associated with cholestasis. The plasma amino acid pattern seen in infants receiving TrophAmine resembles normal 2-hour postprandial levels seen in 1-month-old healthy full-term breast-fed infants. The low pH of TrophAmine allows for large amounts of calcium and phosphorus to be added to the PN solution without precipitation. TrophAmine also contains a higher concentration of branched-chain amino acids. Branched-chain amino acids have been shown to improve nitrogen balance, protein synthesis, and immunocompetence in septic or trauma patients. TrophAmine is used in patients younger than 6 months of age, and an adult amino acid preparation is used in patients 6 months of age or older. TrophAmine can be used in patients with end-stage liver disease and cholestasis because of its branched-chain amino acid profile. Specialized amino acid formulations are available for certain disease states (renal and hepatic failure). These solutions are expensive, and studies have not demonstrated a clear beneficial survival effect when these solutions have been used in patients with renal and hepatic failure. The amino acid formulation designed for use in patients with severe liver failure and hepatic encephalopathy contains increased amounts of branched-chain amino acids and reduced amounts of methionine and aromatic amino acids. However, study results using this formulation are mixed and have not demonstrated a clear beneficial survival effect in these patients. Specialized formulations for renal failure have also been developed, but their use is not widespread due to a lack of clear benefit ( Table 88-4 ).
TrophAmine | Aminosyn | HepatAmine | Aminosyn RF | |
---|---|---|---|---|
Essential Amino Acids * | ||||
l -Isoleucine | 82 | 72 | 113 | 89 |
l -Leucine | 140 | 94 | 138 | 140 |
l -Lysine | 82 | 72 | 76 | 103 |
l -Methionine | 34 | 40 | 13 | 140 |
L-Phenylalanine | 48 | 44 | 13 | 140 |
L-Threonine | 42 | 52 | 56 | 63 |
L-Tryptophan | 20 | 16 | 8 | 32 |
L-Valine | 78 | 80 | 105 | 102 |
Nonessential Amino Acids * | ||||
L-Alanine | 54 | 128 | 96 | — |
L-Arginine | 120 | 98 | 75 | 115 |
L-Aspartic acid | 32 | — | — | — |
L-Glutamic acid | 50 | — | — | — |
L-Glycine | 36 | 128 | 113 | — |
L-Histidine | 48 | 30 | 30 | 83 |
L-Proline | 68 | 86 | 100 | — |
L-Serine | 38 | 42 | 63 | — |
L-Taurine | 2.5 | — | — | — |
L-Tyrosine | 24 | 49 | — | — |
% Branched chain amino acids | 30 | — | 36 | 33 |
% Essential amino acids | 53 | 47 | 52 | 80 |
% Nonessential amino acids | 47 | 53 | 48 | 20 |
There is question as to the benefit of adding albumin to the PN solution in patients with hypoproteinemia. This practice is generally not recommended, because albumin has a short half-life and no nutritional value. Endogenous albumin has a half-life of 21 days, but exogenous albumin has a shorter duration in circulation, and its purpose is to increase oncotic pressure. There is a significant amount of aluminum and sodium present in albumin solutions, which can be detrimental to patients with sensitive fluid status. Although albumin in the setting of acute resuscitation may be associated with a higher survival benefit, there is also a concern that albumin in PN may increase sepsis.
In addition to providing calories to patients, it is also important to include protein in adequate amounts. The most commonly used method for determining the protein needs of full-term infants younger than 1 year of age and older children is the Recommended Dietary Allowances (RDAs) or DRIs for age and gender. The protein recommendations are derived from the minimum amount of protein intake required to maintain nitrogen balance. The protein needs for premature infants or children with chronic disease may be higher than the RDA.
Protein needs decline progressively with age. The protein needs of infants and children are higher than those of adults because of different growth rates. The amount of protein required to enhance protein accretion is higher in sick patients. Increased protein needs may be found in conditions such as protein-losing enteropathy, protein-calorie malnutrition, sepsis, burns, patients on ECMO, or inflammatory gastrointestinal conditions. Infants need a 25% increase in protein intake during the postsurgical period and a 100% increase if they have sepsis or are on ECMO. Protein can be safely administered on the first or second day of life in preterm infants. In most preterm infants, protein is typically started at 2.0 g/kg of amino acids per day the first day PN is used and then increased to 3.0 g/kg on day 2. Due to the rapid growth of preterm infants and increased needs for appropriate growth and accretion of substrate, these patients may require up to 4 g/kg per day of protein. Exceptions to this rule may be in VLBW infants, critically ill patients with hepatic or renal insufficiency (not on dialysis), and patients with disorders of protein metabolism ( Table 88-5 ). Older children and adolescents require protein intakes of 1 to 2 g/kg per day. At our institution, we use a maximum of 150 g protein per day. Unless the patient has hepatic or renal failure, or a disorder of protein metabolism is suspected, there is no evidence to suggest that it is beneficial to start protein at the low amount and then advance it to goal at a slow rate. This practice only results in a delay of adequate nutrition. Protein needs in infants, children, and adolescents vary from 0.75 g/kg 2.5 g/kg per day. Blood urea nitrogen (BUN) is a useful measure of adequate or excessive protein intake if nutritional status, hydration, and renal function are within normal limits.
Infants 0-5 kg | 2-3.5 |
Children 5-20 kg | 1-2.5 |
Children 20-40 kg | 1-2 |
Children/adults >40 kg | 0.8-2 * |
Cysteine is a conditionally essential amino acid in neonates and infants. Improved nitrogen retention has been seen with the administration of cysteine to PN solutions. However, it is not present in significant amounts in amino acid solutions due to instability. Cysteine can be added to TrophAmine to reduce the pH of the PN, which allows for increased phosphorous and calcium solubility. Cysteine should not be added to the PN solution if the infant is acidotic, because cysteine may exacerbate metabolic acidosis. The recommended dose for cysteine is 40 mg/g of PN protein. Taurine can also be found in infant amino acid formulations and plays an integral role in brain and retinal membrane development as well as bile acid conjugation. Some evidence suggests that parenteral supplementation of taurine can prevent cholestatic liver disease. Parenteral supplementation has improved plasma levels and transaminase concentrations in adults with short bowel syndrome, but has not been studied in children. Glutamine was originally classified as a nonessential amino acid but is currently classified as a conditionally essential amino acid. Studies have shown that supplementation with glutamine has improved nitrogen balance and immunocompetence, decreased sepsis, and maintained protein synthesis in postoperative patients. Other studies have shown a beneficial effect of PN supplemented with glutamine in patients with short bowel syndrome or trauma, patients who have undergone bone marrow transplantation, and critically ill burn patients. The current standard of practice in Canada and Europe is to provide parenteral glutamine in adult trauma and burn patients. Beneficial effects from supplementation include decreased incidence of bacteremia, improved nitrogen balance, decreased duration of hospital stay, reduced incidence of severe mucositis and veno-occlusive disease, and a decrease in drug toxicity. Glutamine has also been found to be safe for use with preterm infants, but there has been no significant effect on morbidity or mortality. Intravenous glutamine is not readily available in the United States at this time, and specific recommendations on pediatric use have not yet been put forth due to the limited available data.
Carnitine is synthesized in the body from two essential amino acids, lysine and methionine, and is required for the transport of long-chain fatty acids into the mitochondria. Neonates and infants are unable to produce carnitine endogenously and need an exogenous supply. Serum and tissue carnitine levels may be depleted in patients who are receiving PN exclusively for an extended period. Inadequate carnitine may reduce fatty acid transport, thereby limiting oxidation and energy production. Carnitine supplementation in PN solutions in premature infants has been suggested to correct low free and acylcarnitine levels. Although hereditary carnitine deficiency responds to carnitine supplementation, it is not clear whether the low levels seen in premature infants and patients with short bowel syndrome represent a true deficiency and need to be supplemented. Indeed, although some studies have shown improved levels, fat tolerance, and some increase in growth and nitrogen retention, other studies have failed to show any significant effect or have shown a negative effect with high doses. An intravenous form of l -carnitine is available and may be added to PN formulations. The recommended dose for l -carnitine in pediatrics is 10 to 20 mg/kg per day. It has been suggested that intravenous carnitine be added to the PN of VLBW infants to assist with triglyceride beta-oxidation if triglyceride levels increase to greater than 200 g/dL. However, this practice is not widespread and has not been found to be beneficial in the short term. Although no overwhelming evidence supports supplementation, a typical standard of practice is to provide carnitine parenterally if PN is the only source of nutrition for more than 2 weeks, if the patient has hypertriglyceridemia, or if low serum carnitine levels are present.
Choline is a precursor of acetylcholine and phosphatidylcholine, and is required for the synthesis of very low-density lipoproteins (VLDLs) and for triacylglycerol (TAG) export. Choline deficiency has been suggested as a contributing factor for hepatic steatosis due to the impairment of hepatic TAG secretion. Premature infants and patients on long-term PN without sufficient enteral feeds are at risk for choline deficiency. Buchman et al. showed a reduction in hepatic steatosis and increased plasma free choline levels after administration of a choline supplemented PN solution for 6 weeks. Buchman et al. also showed lower than normal plasma free choline levels in patients receiving home total PN (TPN). This observation was made in about 80% of home PN patients. The provision of oral lecithin caused increased plasma free choline levels as well as decreased hepatic steatosis. Buchman et al. were able to show that low choline status was associated with fatty liver and elevated transaminases. In a trial, 15 patients were randomized to receive standard PN or PN supplemented with 2 g of choline. An improvement in liver function tests was noted in the choline-supplemented group. After the choline supplementation was stopped, there was a recurrence of steatosis.
Carbohydrates
The majority of calories in PN are provided by the intravenous monohydrate form of dextrose, which provides 3.4 kcal/g, different from the 4 kcal/g provided by the enteral form of carbohydrates. Carbohydrates usually provide 50% to 60% of total calorie intake. PN given through a peripheral vein should have a maximum concentration of 10% dextrose because more concentrated dextrose solutions can result in osmolalities greater than 900 mmol/L and an increased risk of phlebitis. In special circumstances, a 12.5% dextrose solution may be used peripherally with caution, but it should not be used in neonates and infants because of the increased risk of extravasation and phlebitis. PN given through a centrally placed line allows for the infusion of solutions with higher dextrose concentrations and osmolalities greater than 900 mmol/L and is typically appropriate for patients requiring PN for more than 7 to 10 days.
It is important to calculate glucose delivery or glucose infusion rate (GIR) in patients who receive PN. The GIR allows practitioners to determine whether glucose delivery will exceed glucose utilization rates. The GIR is expressed in mg/kg of body weight/minute. The recommended GIR for infants is between 5 and 12 mg/kg per minute. Glucose utilization is known to decrease when the GIR exceeds 14 mg/kg per minute. If the GIR is higher than recommended, greater amounts of insulin are released. Insulin hypersecretion stimulates hepatic lipogenesis, producing aglycerol from glucose, and inhibits mitochondrial fatty acid oxidation. These concurrent processes promote a buildup of TAG within hepatocytes. The initial rate of dextrose infusion should be approximately 5 mg/kg per minute in infants. The GIR can then be increased by 2 to 5 mg/kg per minute daily or 5% to 10% per day. In older children, the recommended GIR is between 2 and 5 mg/kg per minute. Urine should be monitored for glucose when PN is started and after changes in GIR. Serum glucose levels should be checked if a patient has glucosuria, and the GIR should be reduced as appropriate. LBW infants, malnourished infants, and children with small glycogen stores are at increased risk of hypoglycemia when parenteral glucose is abruptly stopped. It is commonly recommended that the rate of PN solution be tapered when cycling PN with a dextrose concentration greater than 10% to decrease the risk of rebound hypoglycemia, but a small study in pediatric patients found that abrupt discontinuation on PN was not a concern in children older than 2 years of age. Cycling PN in stable patients over the course of 16 hours may prevent or delay the onset of hepatic steatosis.
Hyperglycemia in critically ill patients who are receiving PN has been associated with increased mortality and morbidity. Increases in energy expenditure and respiratory quotient have been seen with regimens with high glucose infusion rates. Serum glucose levels should be maintained in the normal range for age. Patients receiving PN who have significant hyperglycemia may require an insulin infusion to help achieve and maintain euglycemia. Hyperglycemia is common in stressed LBW and VLBW infants soon after birth. Insulin can be used to maintain serum glucose levels in the normal range and may be considered as an alternative to the use of a hypocaloric regimen. It is preferable to run the insulin as a separate infusion as opposed to adding it to the PN solution, because the insulin may adhere to the tubing, resulting in lower amounts being delivered to the patient. It is also difficult to adjust the rate of the insulin infusion without affecting calorie intake and glucose homeostasis when insulin is mixed with the PN solution.
Lipids
Fat provides a concentrated form of both calories and essential fatty acids, which are required for prostaglandin and membrane lipid synthesis, brain and somatic growth, immune function, skin integrity, and wound healing. Intravenous fat emulsions available in the United States are composed entirely of long-chain triglycerides, usually from soybean and safflower oils. There are two product types available: a 100% soy-based emulsion (Intralipid, Liposyn III) or a 50% soy and 50% safflower oil–based emulsion (Liposyn II). Intralipid is a commonly used intravenous fat and is available in 10%, 20%, and 30% emulsions ( Table 88-6 ). The 20% emulsion is preferred because of its lower phospholipid content and improved triglyceride clearance. The 10% solution may result in hyperlipidemia because of its high phospholipid-to-triglyceride ratio and is infrequently used. Phospholipids are thought to inhibit lipoprotein lipase, the main enzyme responsible for intravenous fat clearance. The 30% emulsion can only be used in a total nutrient admixture and cannot be infused alone into a peripheral vein.
Intralipid 20% * | |
---|---|
Caloric value, kcal/ml | 2 |
Linoleic acid, % | 44-62 |
Linolenic acid, % | 4-11 |
Oleic acid, % | 19-30 |
Palmitic acid, % | 7-14 |
Stearic acid, % | 1.4-5.5 |
Vitamin E activity or α-tocopherol equivalent, mg/1000 mL | 35 |
Osmolarity, mOsm/L | 260 |
Osmolality, mOsm/kg | 350 |
* Intralipid 20% formulation preferred over 10% emulsion due to lower phospholipid content and improved triglyceride clearance.
A greater variety of lipid solutions is commonly used in Europe. Structured lipids (long-chain triglycerides [LCTs] and medium-chain triglycerides [MCTs] attached to a glycerol backbone); MCT emulsions; mixtures of MCT and LCT emulsions; olive oil-containing emulsions; mixtures of soybean oil, MCT, olive oil, and fish oil; and mixtures of MCT, LCT, and fish oil have been developed to prevent complications seen with conventional fat emulsions. These emulsions have been studied in hospitalized patients as well as in home PN patients. The advantages of MCT-containing lipid emulsions are that they are more soluble, are rapidly hydrolyzed by lipases, are quickly eliminated from the circulation, and are taken up by the peripheral tissue. They are not stored by the body, are ketogenic, and are oxidized more rapidly than LCTs. There is less elevation of liver enzymes with these emulsions. Emulsions containing MCTs and LCTs are more efficient and have less of a negative effect on the liver, immune system, and reticuloendothelial system compared with LCT-containing intravenous fat emulsions. They produce a similar amount of prostaglandins and have been shown to be useful in patients with systemic inflammatory response syndrome. Structured lipids have been studied in the pediatric age group. Studies in infants have shown improved lipid levels in those receiving a mixture of MCTs and LCTs. However, these new formulations are not readily available in the United States. Currently Omegaven, a fish oil-containing lipid emulsion, is available in Europe, but in the United States requires submission of an application to the U.S. Food and Drug Administration (FDA) for an investigational new drug (IND). Improvement in PN-associated cholestasis has been shown with use of this emulsion, especially in premature infants.
Improvement of PN-associated cholestasis has been demonstrated in a prospective study evaluating dose reduction of a soybean oil–based intravenous fat emulsion, but a recent retrospective review comparing soybean oil–based lipid emulsion at 1 g/kg per day compared with 2-3 g/kg per day demonstrated no difference in time to cholestasis. The total daily dose of intravenous fat is usually delivered over 24 hours, except when the PN is cycled. However, continuous 24-hour infusions are better tolerated than intermittent infusions. There is a concern about the growth of bacteria in intravenous fat solutions when these solutions have been hung for more than 12 hours. It has been suggested that the hang time be 12 hours or less and that unit doses be used so the bottle is entered only once in order to decrease the incidence of sepsis. Intravenous fat is usually started at an initial dosage that is increased over 1 to 3 days if triglyceride clearance is within normal limits ( Table 88-7 ). There are no clearly defined acceptable values for serum triglycerides during PN administration for hypertriglyceridemia. Although some authors have suggested that in neonates, intravenous lipids should be decreased if triglyceride levels are above 200 mg/dL, we accept triglyceride levels up to 300 mg/dL in infants and young children. A rising triglyceride value in the face of sepsis would be a reason to decrease intravenous fat administration. In adults, triglyceride levels greater than 400 mg/dL have been suggested as a cutoff value above which the intravenous fat dose should be decreased. Triglyceride levels depend on the rate of clearance of the infused fat. This in turn is affected by nutritional status, degree of malnutrition, concurrent administration of medications, and the clinical situation (stress, organ dysfunction, and infection). The impact of intravenous fat administration on lung function has been well discussed in two review articles.
0-5 kg Infants | 5-20 kg Children | 20-40 kg Children | >40 kg Children/Adults | |
---|---|---|---|---|
Initial dose | 0.5-1 | 1-2 | 1-1.5 | 0.5-1 |
Dose increase | 0.5-1 | 1 | 0.5-1 | 0.5 |
Standard dose * | 3 | 3 | 1.5-2 | 0.5-1 |
* Percentage of calories from fat should not exceed 60% of total caloric intake.
In general, during formulation of a PN regimen, the fat percentage should provide 25% to 35% of total calories. There are some exceptions to this rule where fat percentage may be higher, but it should never exceed 60% of total calories. The maximum dose of intravenous fat in infants is 3 g/kg per day. The dosage of intravenous fat decreases with age. Patients who require 100% of their caloric needs from PN need to receive intravenous fat to prevent essential fatty acid deficiency (EFAD). In the extremely LBW infant, EFAD can develop within 48 to 72 hours if no intravenous fat is provided. Preterm infants on PN without intravenous fat and enteral feeds can develop EFAD biochemically within 7 days. Clinical signs of EFAD include growth failure, flaky dry skin, alopecia, thrombocytopenia, increased infections, and impaired wound healing. Historically, EFAD has been diagnosed using triene-to-tetraene ratios above 0.2 and 0.4, respectively. The Mayo Medical Laboratory defines a normal range for triene-to-tetraene ratio for ages 32 days to 17 years to be 0.013 to 0.050. In our practice, in addition to using this ratio we take into consideration the levels of linoleic acid, alpha-linolenic acid, and mead acid, Some literature suggests that only 0.3% to 0.56% of total energy intake from linoleic acid is required to prevent EFAD, as opposed to previous information stating the need of 2% to 4% (0.5 to 1.0 g/kg per day) of total calories needed from fat.
Electrolytes and Minerals
Electrolyte requirements vary with age and are added to the PN solution in maintenance concentrations. These requirements are derived from the RDAs, with allowances made for the efficiency of absorption ( Table 88-8 ). These include sodium, potassium, calcium, magnesium, and phosphorus. Chloride and acetate are used to balance the PN solutions. It is recommended that baseline serum electrolytes are obtained before ordering the PN solution and then electrolytes are added accordingly. Periodic monitoring of serum electrolytes is required, especially in critically ill and malnourished patients. In neonates and children with high calcium and phosphorous needs, the amounts added to a PN solution may be limited because of solubility issues. Increasing the amount of protein and adding cysteine (30 to 40 mg cysteine per g amino acid), thereby lowering the pH of the solution, can allow the addition of higher amounts of calcium and phosphorus to the PN solution without causing precipitation. In instances where the patient’s calcium and phosphorous requirements cannot be met via the PN solution, a separate infusion of calcium or phosphorus may need to be provided. Patients with high calcium needs and those who cannot get all the calcium in their PN may benefit from enteral calcium administration if tolerated. It should be noted that in patients with hypocalcemia and hypomagnesemia, magnesium status needs to be normalized before calcium levels will respond to calcium supplementation.
Infants 0-5 kg | Children | Adolescents/Adults >40 kg | ||
---|---|---|---|---|
5-20 kg | 20-40 kg | |||
Sodium | 2-5 mEq/kg | 2-6 mEq/kg | 2-3 mEq/kg | 80-150 mEq/day |
Potassium | 2-4 mEq/kg | 2-3 mEq/kg | 1.5-2.5 mEq/kg | 40-60 mEq/day |
Phosphorus | 2-4 mEq/kg | 1-2 mEq/kg | 1-1.5 mEq/kg | 30-60 mEq/day |
Chloride | 2-5 mEq/kg | 2-5 mEq/kg | 2-3 mEq/kg | 80-150 mEq/day |
Acetate | Balance | Balance | Balance | Balance |
Calcium | 1-4 mEq/kg * | 0.5-1 mEq/kg | 10-25 mEq/day | 10-20 mEq/day |
Magnesium | 0.3-0.5 mEq/kg | 0.3-0.5 mEq/kg | 0.3-0.5 mEq/kg | 10-30 mEq/day |
Vitamins
Although specific parenteral vitamin dosages for pediatrics in the United States have not been updated since 1988, the ESPEN/ESPGHAN/ESPR recommendations from 2005 do not suggest any changes. Furthermore, a 2012 ASPEN position paper on parenteral multivitamins and trace elements did not recommend changes in dosing of current multivitamins. There are currently two types of multivitamin preparations (Infuvite Adult, Infuvite Pediatric, M.V.I. Pediatric, M.V.I.-12, and M.V.I. Adult) used in the United States: pediatric and adult formulations. The pediatric MVI was designed to meet the needs of preterm and term infants and children up to 10 years of age. It is dosed on a per-kilogram basis, and preterm infants receive 40% of a vial per kg of body weight. The adult and pediatric MVI preparations differ in the concentration of the various vitamins per milliliter. In general, the adult MVI has a greater concentration of vitamins, except it contains less vitamin D compared with the pediatric formulation. Some adult MVI has no vitamin K. In children 10 years of age or older, the adult MVI is used with the addition of vitamin K. The recommendation is for adult multivitamin preparations to contain vitamin K ( Table 88-9 ). The dosing recommendations for vitamins take into account the losses associated with administration. Some vitamins may adhere to the intravenous tubing, whereas others may be affected by light exposure while the PN solution is being administered. It is recommended that the adult multivitamin preparation not be used for children because it contains propylene glycol and polysorbate additives that may be toxic to the premature infant.
Preterm infants/kg | Term Infants and Children >1 Year | MVI Pediatric (5 mL) | MVI-12 * (5 mL) | |
---|---|---|---|---|
Biotin (µg) | 5-8 | 20 | 20 | 60 |
Folate (µg) | 56 | 140 | 140 | 400 |
Niacin (mg) | 4-6.8 | 17 | 17 | 40 |
Pantothenic acid (mg) | 1-2 | 5 | 5 | 15 |
Riboflavin (mg) | 0.15-0.2 | 1.4 | 1.4 | 3.6 |
Thiamine (mg) | 0.2-0.35 | 1.2 | 1.2 | 3 |
Vitamin A (retinol) (IU) | 700-1500 | 2300 | 2300 | 3300 |
Vitamin B (mg) | 0.15-0.2 | 1 | 1 | 4 |
Vitamin B (µg) | 0.3 | 1 | 1 | 5 |
Vitamin C (mg) | 15-25 | 80 | 80 | 100 |
Vitamin D (IU) | 40-160 | 400 | 400 | 200 |
Vitamin E (tocopherol) (IU) | 2.8-3.5 | 7 | 7 | 10 |
Vitamin K (mg) | 0.01 (10 mcg) | 0.2 | 0.2 | * |
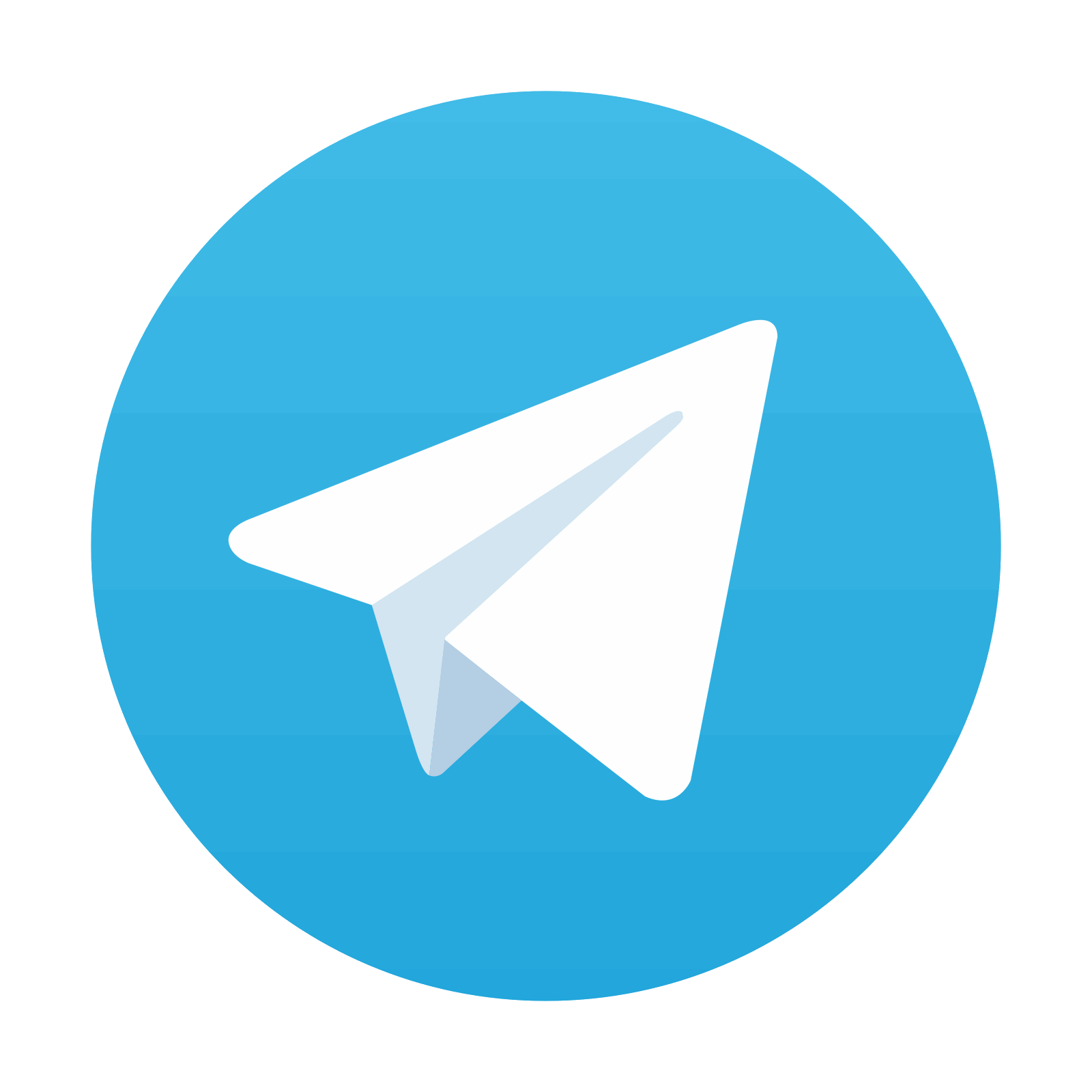
Stay updated, free articles. Join our Telegram channel

Full access? Get Clinical Tree
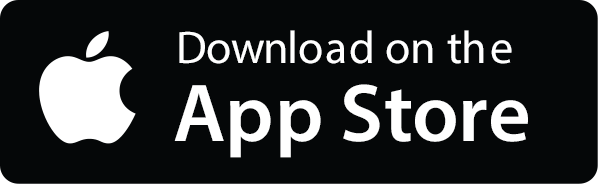
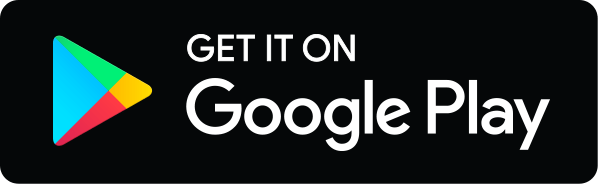
