Many of the common digestive signs and symptoms of pediatrics, including diarrhea and weight loss, are the result of disruption of either nutrient digestion or absorption. As such, maldigestion and malabsorption are not specific diagnoses but rather mechanistic categories that result from one or more pathophysiologic events that impair nutrient processing and result in a deficiency state of compromised nutriture, growth, or other abnormality. Digestive or absorptive compromise can present with only laboratory findings, subtle nondigestive symptoms, or blatant gastrointestinal or systemic complaints. Appropriate growth in the setting of an adequate calorie intake remains the best indicator of proper digestive function in the pediatric population.
This chapter focuses on the pathophysiology of maldigestion and malabsorption for the basic nutrient groups: fats, proteins, carbohydrates, vitamins, and specific micronutrients. Signs and symptoms, diagnostic testing, and imaging studies are also discussed. Many of the specific gastrointestinal disorders of pediatric gastroenterology that result in maldigestion or malabsorption are discussed in other chapters throughout this text.
The assimilation of nutrients by the gastrointestinal tract is a multistep process that can be compromised at several levels, including digestion within the lumen, absorption and processing by enterocytes, and transport into the systemic circulation. Please see Chapter 2 for discussion of the basic aspects of digestion and absorption. Liberation or solubilization is an early prerequisite for the processing of many nutrients that occurs within the gastrointestinal chyme of the digestive lumen. Fat and fat-soluble vitamins are solubilized with the aid of pancreatic and hepatic secretions to form micelles in which luminal triglyceride disassembly occurs. Luminal digestion by pancreatic enzymes is critical for protein and carbohydrate processing as well. Additional steps of digestion occur on the enterocyte membrane, particularly for polypeptides, oligosaccharides, and disaccharides. The absorption of the basic digestive components occurs at the level of the brush border of enterocytes by either active or passive processes, with postabsorptive transport via the portal vein and lymphatic channels. Coordination of the digestive process is complex and is regulated through both nerve and hormonal function, which coordinate digestive secretion, gastrointestinal motility and blood flow, and mucosal absorption.
Lipids
Dietary fat absorption is normally very efficient, with more than 94% of ingested fat assimilated into the body (see Chapter 2 for full details). The major portion of dietary fat comprises water-insoluble triacylglycerol that must interact with water to be absorbed and processed by the intestine. The digestion of dietary triacylglycerol begins with hydrolysis by gastric lipase, which in humans originates in the gastric fundus, favoring short- and medium-chain triglycerides over the longer chains. There is no absorption of fat in the stomach, other than for short-chain fatty acids; nevertheless, the stomach is the major site of fat emulsification, as it churns and breaks apart the lipids into progressively smaller droplets. Free fatty acid (FFA) release by gastric lipolysis further stimulates secretion of pancreatic lipase and colipase, which are responsible for the majority of lipid hydrolyses within the duodenum, yielding monoglyceride and FFAs. Bile salts further enhance fat solubilization; because of their amphipathic nature, they impose a detergent-like action on particles of dietary fat, which leads to emulsification into much smaller droplets. This process increases the available surface area of the fat, making it accessible for digestion by lipase. The bile salts, monoglycerides, fatty acids, and phospholipids form micelles to serve as a transfer vehicle to the enterocyte membrane. In this form, the monoglyceride and fatty acids enter the unstirred water layer that lies adjacent to the enterocyte and are then absorbed across the apical membrane of the cell. This process was once believed to occur by passive diffusion, but evidence that is more recent has implicated active transport processes involving proteins such as FATP4 and CD36. Within the enterocyte, the fatty acids are directed to the endoplasmic reticulum (ER), where they are converted back to triglycerides to be packaged into chylomicrons, the intestine’s unique lipoprotein. Apolipoprotein B (apoB) is the requisite surface structural protein that facilitates the formation of chylomicrons within the ER, whereas microsomal triglyceride transfer protein (MTTP) promotes lipoprotein biogenesis by shuttling neutral lipid from within the bilayer of the ER to an acceptor apoB molecule. The triglyceride is transported from the ER to the Golgi apparatus in a specialized vesicle, the pre-chylomicron transport vesicle (PCTV), which requires a protein complex, a component of which is Sar1b. From the Golgi, chylomicrons are packaged for vesicular transport to the basolateral membrane where they are exocytosed into the lamina propria, and enter the mesenteric lymph duct to be carried in the lymph system to the circulation via the left subclavian vein.
Disturbances in any of the aforementioned steps in fat processing can lead to fat malabsorption and steatorrhea. Defects that impede gastric mixing, such as gastric resections or motility disorders that hasten gastric emptying, can lead to fat malabsorption as a result of decreased interaction time with digestive secretions. Within the duodenum, decreased solubilization of fats occurs if the luminal concentration of conjugated bile acids falls below the critical concentration required for micelle formation. Liver disease or biliary obstruction may lead to decreased synthesis and/or secretion of conjugated bile acids, whereas intestinal loss of bile acids may occur as a result of ileal resection or from severe mucosal disease (as may be seen in Crohn’s disease). Genetic defects in bile acid transport (e.g., progressive familial intrahepatic cholestasis-1 [PFIC1]) and inborn errors of bile acid synthesis (IBABAS) can cause subsequent digestive abnormalities, as can deconjugation of the bile acids, which may occur as a consequence of bacterial overgrowth.
If exocrine pancreatic function is severely reduced, impairment of pancreatic lipase and colipase secretion results in decreased luminal hydrolysis of dietary fat. This in turn leads to increased quantities of neutral triglyceride reaching the colon, where the bacterial flora hydrolyzes them, releasing FFAs and glycerol. Steatorrhea is usually severe, with fat absorption being reduced to 70% to 40% of ingested fat; however, these stools are soft and pasty rather than liquid. Potential causes may include chronic pancreatitis, pancreatic duct obstruction, or pancreatic resection. In children, however, the most frequent cause, by far, is cystic fibrosis and a sweat-chloride test confirms the diagnosis (see Chapter 81 ). Exocrine pancreatic insufficiency from hypoplasia of the pancreas may be seen in multiple syndromes, the most frequent of which is Shwachman-Diamond syndrome (others include Johanson-Blizzard syndrome and Pearson syndrome; see Chapter 81 ). Fecal elastase appears to be a relatively simple yet sensitive and specific screening test for exocrine pancreatic function.
Congenital lipase or colipase deficiency is a rare cause of pancreatic fat malabsorption. Lipase deficiency presents with severe steatorrhea in infancy or early childhood, with the fat absorption coefficient being less than 50%, but despite the maldigestion of dietary fat, failure to thrive is not a classic feature. Colipase deficiency leads to less severe steatorrhea, again without failure to thrive, but there is an association with biliary atresia, and so these patients may present with jaundice and hepatomegaly. Combined lipase–colipase deficiency has also been described. These diagnoses are confirmed by the direct assay of lipase activity in the duodenal juice, and the children respond well to exogenous pancreatic enzyme supplementation. Even with normal pancreatic enzyme concentrations, low luminal pH or excessive calcium ingestion can reduce pancreatic lipase activity and cause steatorrhea.
Generalized intestinal mucosal disease, which can occur in celiac disease or tropical sprue and with infections such as giardia, may be associated with fat malabsorption. These disorders reduce surface area and along with decreased enterocyte function and mucosal inflammation, impede absorption of FFAs and monoglycerides.
Decreased Chylomicron Formation
Intestinal fat absorption is also impaired in diseases that decrease intracellular formation of chylomicrons and lead to accumulation of lipids within the enterocytes. Abetalipoproteinemia (ABL), hypobetalipoproteinemia (HHBL), and chylomicron retention disease (CRD) represent diseases of hypocholesterolemia that can present in the first few years of life with steatorrhea from fat malabsorption and failure to thrive. This is often accompanied by other gastrointestinal symptoms such as vomiting and abdominal distension. Deficiencies in essential fatty acids and lipid-soluble vitamins lead to extraintestinal manifestations. Most notably, neurologic degeneration may present with ataxia, dysmetria, and loss of proprioception and deep tendon reflexes. Early treatment with vitamin E has been shown to delay or prevent the development of neurologic dysfunction. Hepatic involvement in the form of steatosis and elevated serum transaminase levels is frequently noted; however, this does not seem to progress to more serious liver disease. Ophthalmologic findings are variable, but loss of night vision is often reported as the first symptom, and without intervention, blindness may ensue.
The differential may be shortened following review of the patient’s lipid profile. With extremely low levels of triglycerides, apoB, and low-density lipoproteins (LDLs), the diagnosis can be narrowed to either ABL or HHBL. If the parents of the patient are screened, ABL and HHBL can be further differentiated: parents of patients with HHBL typically have half-normal levels of apoB and LDL, whereas parents of patients with ABL usually have normal lipid profiles consistent with autosomal codominant and recessive inheritance patterns, respectively. On endoscopy, a white duodenal mucosa is frequently visualized secondary to lipid accumulation, and histology shows multi-vacuolated enterocytes in intestinal villi of normal architecture. Molecular sequencing of the Microsomal Trigyceride Transfer Protein (MTP) or apolipoprotein B (APOB) genes provides a definitive diagnostic test.
Chylomicron retention disease (CRD) presents with similar symptoms and has outcomes similar to those of other familial hypocholesterolemia diseases. The mutation occurs in the SAR1B gene that encodes the Sar1b protein. When mutated, this defect impedes migration of the PCTV from the ER to the Golgi, and these vesicles accumulate in the cytoplasm of enterocytes. Unlike the other two diseases, the presence of normal triglycerides is almost pathognomonic for CRD. These three diseases are treated primarily with careful diet control with fat restriction and supplementation with fat-soluble vitamins; in particular, vitamin E is critical for the prevention or reversal of complications.
Finally, congenital diseases (primary intestinal lymphangiectasia) or obstruction of lymphatic vessels (metastatic solid tumors, lymphoma, retroperitoneal fibrosis, or trauma) may impair chylomicron flow and cause malabsorption of dietary fat. Lymphatic vessels in the mucosa typically become dilated and chylomicrons are lost into the intestinal lumen, causing mild to moderate steatorrhea.
Proteins and Amino Acids
Protein structure is determined by amino acid number and sequence as well as through chain folding, which provides secondary and tertiary structures. Protein digestion begins with intraluminal denaturation and chain cleavage followed by brush border hydrolysis and subsequent transport across the mucosa as tripeptides and dipeptides and amino acids (see Chapter 2 ). The maldigestion/malabsorption of dietary proteins with increased nitrogen loss in the stool must be differentiated from leakage of serum proteins into the lumen that results from compromised intestinal mucosal barrier function. This latter disorder, known as protein-losing enteropathy, is discussed in Chapter 33 .
Compromised Intraluminal Digestion
Gastric protein digestion is initiated with the secretion of acid and digestive enzymes such as pepsin by the stomach lining. Substantial gastric resection with decreased acid secretion and impaired mixing can compromise intraluminal protein digestion. Exocrine pancreatic insufficiency (decreased enzyme and bicarbonate secretion) or impaired intestinal enteropeptidase activity (reduced activation of luminal and mucosal pro-enzymes) will compromise intraluminal protein digestion as well. Intraluminal protein digestion will also be influenced by loss of intestinal length (short bowel syndrome), bypass forms of bariatric surgery, or in the setting of jejunal feedings where the initial phases of gastric and duodenal luminal digestion are bypassed.
Compromised Mucosal Digestion and Absorption of Oligopeptides and Amino Acids
Further digestion of short oligopeptides to tripeptides and dipeptides and amino acids requires digestion by multiple specific peptidases located on the enterocyte brush border. Any process that compromises enzyme activation, typically mediated by trypsin in a neutral pH, will affect this final step in protein assimilation. Mucosal disorders such as celiac disease can impair protein mucosal hydrolysis and transport. Loss of absorptive surface area is also a factor in short bowel syndrome or jejunal ileal bypass, as these will also affect mucosal digestion and peptide/amino acid transport.
Congenital Disorders of Proteolytic Enzyme Secretion and Amino Acid Transport ( Table 31-1 )
Although extremely rare, congenital deficiencies of proteolytic enzyme secretion have been described for trypsinogen (OMIM #614044) caused by mutation of Protease, serine, 1 ( PRSS1 ) and the brush border enterokinase/enteropeptidase deficiency (omim #226200) caused by mutation of PRSS7 . Affected infants can have overlapping presentations with chronic diarrhea, failure to thrive, hypoproteinemia, and skin rash. Congenital trypsinogen deficiency can also be associated with imperforate anus.
Disorder | Gene Inheritance | Malabsorbed Substrates | Suggested Mechanism of Malabsorption | Clinical Features | Ref(s) |
---|---|---|---|---|---|
|
| Neutral amino acids (tryptophan, leucine, methionine, phenylalanine, tyrosine, valine, histidine, lysine, and others) | Decreased intestinal absorption of neutral amino acids | Patients can be asymptomatic or have pellagra-like photosensitive skin rash, cerebellar ataxia, emotional instability, mental retardation, diarrhea, amino aciduria | |
|
| Cystine and/or dibasic amino acids (lysine, ornithine, arginine) |
|
| |
|
| Dibasic amino acids (lysine, ornithine, arginine) | Defect of the basolateral transporter (y+LAT-1) for dibasic amino acids (also malabsorption of dipeptides and oligopeptides) in liver and kidney | Lysine deficiency symptoms with sparse hair, hyperammonemia, nausea, vomiting, diarrhea, protein malnutrition, failure to thrive, hepatomegaly/cirrhosis, aversion to protein-rich food, intellectual impairment, leukopenia, osteopenia/osteoporosis | |
|
| Lysine | Decreased intestinal absorption of lysine | Mental retardation, malnutrition, failure to thrive | |
|
| L-Proline, hydroxyproline | Impaired intestinal absorption of L-proline | No significant symptoms, aminoaciduria | |
|
| Tryptophan | Intestinal tryptophan transport defect | Blue discoloration of diapers, failure to thrive, hypercalcemia, nephrocalcinosis | |
|
| Methionine | Intestinal methionine transport defect | Mental retardation, seizures, diarrhea, white hair, blue eyes, hyperpnea; urine has characteristic sweet smell of dried celery | |
|
| Lysine, arginine | Impaired intestinal lysine and arginine absorption | Congenital cataract, aminoaciduria, mental retardation, rickets, constipation choreoathetosis, renal disease |
Small intestinal amino acid transport occurs by way of type-specific brush border cotransport proteins, which are also present in the renal tubule and are responsible for the reabsorption of amino acids from glomerular filtrate. Most congenital defects of amino acid transport affect both brush border enterocyte absorption as well as renal reabsorption for each subgroup of amino acids. These proteins differ from the transporters in the basolateral surface of the enterocyte, which are involved in transport disorders such as lysinuric protein intolerance. Congenital amino acid transport defects are often of minimal nutritional consequence because the essential amino acids can be absorbed in sufficient quantity in the form of tripeptides and dipeptides through different brush border transport mechanisms. The predominant diagnostic and pathophysiologic feature of most of these disorders is the excessive excretion of specific classes of amino acids in the urine with associated kidney disease. Nutritional symptoms can be encountered in settings with poor nutritional protein intake as in underdeveloped countries. In Hartnup’s disease, a disorder of neutral amino acid transport, dietary-associated essential serum amino acid deficiencies can lead to niacin deficiency through deficient synthesis with pellagra-like features of diarrhea, dermatitis, and neurologic findings of cerebellar ataxia and psychosis. The more common and characterized congenital amino acid transport deficiencies and associated features are described in Table 31-1 .
Significant progress has been made in the study and identification of proton-coupled oligopeptide transporters that are present in a variety of organs, including intestine, kidney, and brain. These proteins mediate transport of dipeptides and tripeptides as well as peptide-like drugs across the intestinal brush border and include the specifically identified proteins PepT1 (SLC15A1), PepT2 (SLC15A2), PhT1 (SLC15A4), and PhT2 (SLC15A3). Gene mutations affecting protein function with resultant malabsorption have yet to be identified for the oligopeptide transporters.
Carbohydrates
In infants, lactose (glucose + galactose) serves as the primary carbohydrate, as it is found in breast milk and most cow’s milk–based infant formulas. As infants wean and advance their diets, however, starch (glucose polymers) becomes the predominant carbohydrate. The two chief constituents of starch are amylose, which is non-branching in structure, and amylopectin, which consists of highly branched chains. Adults in the Western world consume 100 to 800 g of carbohydrates daily, with starches accounting for about 50%, and the disaccharides sucrose, lactose, and maltose making up the remainder.
For full details on carbohydrate metabolism, please see Chapter 2 . Briefly, the breakdown of starch begins in the oral cavity by salivary α-amylase, but the bulk of starch digestion occurs in the duodenum by pancreatic amylase, giving the resultant maltose (two glucose molecules) and maltotriose (three glucose molecules) as well as alpha-limit dextrins. Because only monosaccharides can be absorbed across the enterocyte membrane, further digestion of disaccharides and starch breakdown products occurs via enzymes located in the brush border membrane. At this stage, monosaccharides cross the enterocyte apical membrane via carrier-mediated transport. Glucose and galactose are cotransported with sodium through the sodium-glucose transporter 1 (SGLT1) utilizing the sodium gradient generated by the Na+/K+-ATPase pump located in the basolateral membrane. Fructose is transported by facilitated diffusion handled by glucose transporter 5 (GLUT5). All monosaccharides subsequently exit the enterocyte by facilitated diffusion through the GLUT2 channel at the basolateral membrane to enter the portal circulation. With defects in the process, undigested carbohydrates reach the colon where they undergo bacterial degradation, culminating in fermentation, which results in the formation of short-chain fatty acids, carbon dioxide, hydrogen, and methane. These products lead to symptoms typical of carbohydrate malabsorption including acidic watery stools, abdominal distension, and flatulence.
Defective Intraluminal Hydrolysis of Carbohydrates
Pancreatic α-amylase is normally secreted in excess, and for this reason, carbohydrate hydrolysis is impaired in only severe forms of pancreatic insufficiency ( Table 31-2 and Chapter 82 ). Pancreatic amylase deficiency has been described in a small number of patients. These children showed poor weight gain on a diet containing starch, despite an adequate caloric intake. Weight gain and growth improved when starch was eliminated from the diet and replaced by disaccharides.
Disorder | Gene Inheritance | Malabsorbed Substrates | Suggested Mechanism of Malabsorption | Clinical Features | Ref(s) |
---|---|---|---|---|---|
Pancreatic Disease | |||||
|
| Lipids, carbohydrates, protein | Abnormal transport of Cl − and Na + leading to thickened secretions | Recurrent respiratory infections, pancreatic insufficiency, failure to thrive | |
|
| Lipids, carbohydrates, protein | Exocrine pancreatic insufficiency | Chronic or cyclic neutropenia, thrombocytopenia, and anemia. Eczema. Metaphyseal abnormalities, growth retardation | |
|
| Lipids, carbohydrates, protein | Exocrine pancreatic insufficiency | Congenital aplasia of the alae nasi, ectodermal scalp defects, and imperforate anus | |
| Mitochondrial | Lipids, carbohydrates, protein | Exocrine pancreatic insufficiency secondary to mitochondrial DNA deletions | Refractory sideroblastic anemia and vacuolization of marrow precursors | |
Enterocyte Disorders | |||||
|
| Protein, fat | Defective activation of pancreatic proenzymes owing to lack of intestinal enterokinase | Diarrhea, failure to thrive, hypoproteinemia, edema, anemia | |
|
| Carbohydrates, fat, cobalamin, electrolytes, water | Villus atrophy with microvillus inclusions in enterocytes, absent or shortened brush border microvilli | Severe watery diarrhea and steatorrhea requiring total parenteral nutrition | |
|
| Generalized malabsorption | Enteropathy with villus atrophy and inflammation | Hyperinsulinism, profound congenital sensorineural deafness, enteropathy, renal tubular dysfunction | |
|
| Generalized malabsorption | Villus atrophy | Polyendocrinopathies, severe diarrhea, hemolytic anemia | |
|
| Generalized malabsorption | Lack of enteroendocrine cells | Severe diarrhea, failure to thrive, type I diabetes mellitus | |
|
| Generalized malabsorption | Intestinal epithelial cell dysplasia and villus atrophy | Severe diarrhea, failure to thrive |
Disaccharidase Deficiencies ( Table 31-3 )
Sucrase-Isomaltase Deficiency
Congenital sucrase-isomaltase deficiency (CSID) was first described by Weijers and colleagues in 1960. It is generally considered to be a rare condition, with the homozygous condition approximating 0.2% in individuals of European descent; however, much higher incidence is found among Alaskan and Canadian natives, and incidence is as high as 5% to 10% in Greenlanders. CSID infants receiving human milk and lactose-containing formulas do not develop symptoms, but once exposed to sucrose and starch (baby juices, baby food fruits, crackers, and other starches) they often progress to severe diarrhea, diaper rash, failure to thrive, and abdominal distension. In older children, intermittent diarrhea, cramps, and gassiness are more prevalent. Symptoms, and especially starch tolerance, often improve with age, and the correct diagnosis may be missed for years, frequently attributed to toddler’s diarrhea or irritable bowel syndrome. In older subjects, studies have identified those who present with dyspepsia, vomiting, and flatulence rather than the classic watery diarrhea and poor growth. In several studies that examined disaccharidase levels from intestinal biopsies, sucrase deficiency was found in 11% to 13% of biopsy specimens, suggesting that CSID may be much more common than previously thought. Treatment includes avoidance of sucrose or maltose. More recently, sacrosidase, marketed as Sucraid, has been approved for the treatment of CSID, which normalizes symptoms and stool frequency. Typically, doses are split, with half given at the onset of a meal and half at the midpoint. The current recommendations are also to perform skin tests on patients with asthma before initiating sacrosidase administration.
Disorder | Gene Inheritance | Malabsorbed Substrates | Suggested Mechanism of Malabsorption | Clinical Features | Ref(s) |
---|---|---|---|---|---|
Malabsorption of Carbohydrates | |||||
| SI |
| Impaired digestion of disaccharide and starch secondary to enzyme deficiency on the brush border membrane | Acidic diarrhea proportional to ingested carbohydrate | |
|
| Lactose | Impaired digestion of disaccharide secondary to enzyme deficiency on the brush border membrane | Acidic diarrhea proportional to ingested carbohydrate | |
|
|
| Selective defect in glucose and galactose sodium cotransport system | Severe diarrhea and metabolic acidosis, especially during neonatal period | |
|
| Fructose | Impaired transport of fructose across the brush border membrane | Diarrhea, bloating | |
|
| Trehalase | Impaired digestion of disaccharide secondary to enzyme deficiency | Acidic diarrhea and bloating may follow mushroom ingestion | |
|
|
| Transport defect of monosaccharides in the basolateral membrane of enterocytes as well as in liver and kidney | Carbohydrate malabsorption, tubular nephropathy, hepatomegaly, failure to thrive, and fasting hypoglycemia | |
Malabsorption of Lipids | |||||
| PNLIP | Lipids | Impaired luminal lipid hydrolysis in the duodenum | Severe steatorrhea without failure to thrive | |
|
| Lipids | Dysfunction in MTTP causes impaired apolipoprotein B processing and secretion | Steatorrhea, failure to thrive, low fat-soluble vitamins. Low LDL, apoB, and triglycerides | |
|
| Lipids | Impaired production of apolipoprotein B | Steatorrhea, failure to thrive, low fat-soluble vitamins. Low LDL, apoB, and triglycerides | |
|
| Lipids | Defect in chylomicron transport from the ER to the Golgi in enterocytes | Steatorrhea, failure to thrive, low fat-soluble vitamins. Normal triglycerides |
Lactase Deficiency: The most common type of carbohydrate maldigestion and malabsorption is caused by intestinal lactase deficiency, which can be subdivided into congenital, adult-onset, and secondary lactase deficiency. Congenital lactase deficiency (alactasia) is a rare disorder inherited in an autosomal recessive manner. The typical symptoms start several days after birth, with the onset of breast-feeding or ingestion of lactose-containing formula. Typical symptoms include acidic liquid diarrhea, abdominal distension, and severe malnutrition. The infants appear hungry and vomiting is rarely noted. Treatment consists of strict adherence to a lactose-free diet. Primary, adult type hypolactasia, affects the majority of the world’s human population, with more than 75% of the human adult population showing a decline of lactase level to about 5% to 10% of their level at birth. Incidence varies between different populations and age of onset because the expression of lactase is genetically determined. Up to 80% of individuals of African, Arab, and Latino descent, and nearly 100% of Native Americans and Asians, reveal adult type hypolactasia; the lowest prevalence (<5%) is detected in populations in northwestern Europe. Typically, symptoms develop in late childhood and into adolescence. Abdominal discomfort begins soon after consuming milk or milk products and may include pain, diarrhea, and flatulence. The symptoms are proportional to the amount of lactose ingested, but there is a wide spectrum of tolerances with regard to source and volume. Lactose avoidance/limitation as well as supplemental lactase ingestion serves to reduce symptoms. Secondary lactase deficiency occurs following infectious gastroenteritis or injury to the small intestinal mucosa. Lactase is the last of the disaccharidases to recover, and patients should be warned that recovery could take weeks to months.
Trehalase Deficiency: Trehalose (alpha- d -glucopyranoside) is a nonreducing disaccharide composed of two glucose molecules that occurs in mushrooms, some microorganisms (such as algae and ascaris lumbricoides), and in many insects. It may also be used as a food additive. Trehalase deficiency can cause symptoms similar to those of other disaccharidase deficiencies including vomiting, abdominal pain, bloating, and diarrhea.
Maltase Deficiency: Several maltases (glucoamylases) have been identified and are responsible for the digestion of maltotriose. The clinical significance of maltase deficiency appears to be minimal, but patients may present with chronic diarrhea and abdominal distension. Symptoms start with the introduction of starch and formula containing short polymers of starch. Diagnosis is by duodenal biopsy and evidence of normal pancreatic amylase, which also breaks down starch.
Carbohydrate Transport Defects ( Table 31-3 )
Glucose-galactose malabsorption was first described in 1962 by Lindquist and Meeuwisee, and was subsequently shown to be caused by the lack of the sodium-coupled transporter SGLT1. Symptoms of malabsorption may be elicited by ingestion of as little as 6 g of glucose and include severe, life-threatening diarrhea, particularly during the neonatal period, with subsequent severe metabolic acidosis and hyperosmolar dehydration. Diarrhea ceases immediately on withdrawal of the offending carbohydrates (glucose, galactose, and lactose) from the diet.
Fructose Malabsorption: Fructose is transported across the brush border membrane by GLUT5, and both fructose and glucose are exported through the basolateral membrane via the GLUT2 transporter. Expression of GLUT5 in the gut of rodents does not occur until the time of weaning, and in young children, a similar expression pattern may be responsible for fructose intolerance that leads to toddler’s diarrhea, for example, with ingestion of excessive fruit juice. Malabsorption of fruit juices is dose dependent, with diarrhea developing when the daily consumption of juice exceeds 15 mL/kg body weight.
Surprisingly, GLUT2 is not required for intestinal sugar absorption, and only 16% of those with mutations have intestinal symptoms such as diarrhea. However, GLUT2 is essential for reabsorption in the proximal tubule, so defects may result in massive glucosuria. In 1997, Santer and colleagues first identified mutations in GLUT2 as causative of Fanconi-Bickel syndrome, a rare autosomal recessive disorder characterized by carbohydrate malabsorption, tubular nephropathy, hepatomegaly and abnormal glycogen accumulation, failure to thrive, and fasting hypoglycemia. These patients most frequently present with failure to thrive, rickets, and osteopenia.
Carbohydrates
In infants, lactose (glucose + galactose) serves as the primary carbohydrate, as it is found in breast milk and most cow’s milk–based infant formulas. As infants wean and advance their diets, however, starch (glucose polymers) becomes the predominant carbohydrate. The two chief constituents of starch are amylose, which is non-branching in structure, and amylopectin, which consists of highly branched chains. Adults in the Western world consume 100 to 800 g of carbohydrates daily, with starches accounting for about 50%, and the disaccharides sucrose, lactose, and maltose making up the remainder.
For full details on carbohydrate metabolism, please see Chapter 2 . Briefly, the breakdown of starch begins in the oral cavity by salivary α-amylase, but the bulk of starch digestion occurs in the duodenum by pancreatic amylase, giving the resultant maltose (two glucose molecules) and maltotriose (three glucose molecules) as well as alpha-limit dextrins. Because only monosaccharides can be absorbed across the enterocyte membrane, further digestion of disaccharides and starch breakdown products occurs via enzymes located in the brush border membrane. At this stage, monosaccharides cross the enterocyte apical membrane via carrier-mediated transport. Glucose and galactose are cotransported with sodium through the sodium-glucose transporter 1 (SGLT1) utilizing the sodium gradient generated by the Na+/K+-ATPase pump located in the basolateral membrane. Fructose is transported by facilitated diffusion handled by glucose transporter 5 (GLUT5). All monosaccharides subsequently exit the enterocyte by facilitated diffusion through the GLUT2 channel at the basolateral membrane to enter the portal circulation. With defects in the process, undigested carbohydrates reach the colon where they undergo bacterial degradation, culminating in fermentation, which results in the formation of short-chain fatty acids, carbon dioxide, hydrogen, and methane. These products lead to symptoms typical of carbohydrate malabsorption including acidic watery stools, abdominal distension, and flatulence.
Defective Intraluminal Hydrolysis of Carbohydrates
Pancreatic α-amylase is normally secreted in excess, and for this reason, carbohydrate hydrolysis is impaired in only severe forms of pancreatic insufficiency ( Table 31-2 and Chapter 82 ). Pancreatic amylase deficiency has been described in a small number of patients. These children showed poor weight gain on a diet containing starch, despite an adequate caloric intake. Weight gain and growth improved when starch was eliminated from the diet and replaced by disaccharides.
Disorder | Gene Inheritance | Malabsorbed Substrates | Suggested Mechanism of Malabsorption | Clinical Features | Ref(s) |
---|---|---|---|---|---|
Pancreatic Disease | |||||
|
| Lipids, carbohydrates, protein | Abnormal transport of Cl − and Na + leading to thickened secretions | Recurrent respiratory infections, pancreatic insufficiency, failure to thrive | |
|
| Lipids, carbohydrates, protein | Exocrine pancreatic insufficiency | Chronic or cyclic neutropenia, thrombocytopenia, and anemia. Eczema. Metaphyseal abnormalities, growth retardation | |
|
| Lipids, carbohydrates, protein | Exocrine pancreatic insufficiency | Congenital aplasia of the alae nasi, ectodermal scalp defects, and imperforate anus | |
| Mitochondrial | Lipids, carbohydrates, protein | Exocrine pancreatic insufficiency secondary to mitochondrial DNA deletions | Refractory sideroblastic anemia and vacuolization of marrow precursors | |
Enterocyte Disorders | |||||
|
| Protein, fat | Defective activation of pancreatic proenzymes owing to lack of intestinal enterokinase | Diarrhea, failure to thrive, hypoproteinemia, edema, anemia | |
|
| Carbohydrates, fat, cobalamin, electrolytes, water | Villus atrophy with microvillus inclusions in enterocytes, absent or shortened brush border microvilli | Severe watery diarrhea and steatorrhea requiring total parenteral nutrition | |
|
| Generalized malabsorption | Enteropathy with villus atrophy and inflammation | Hyperinsulinism, profound congenital sensorineural deafness, enteropathy, renal tubular dysfunction | |
|
| Generalized malabsorption | Villus atrophy | Polyendocrinopathies, severe diarrhea, hemolytic anemia | |
|
| Generalized malabsorption | Lack of enteroendocrine cells | Severe diarrhea, failure to thrive, type I diabetes mellitus | |
|
| Generalized malabsorption | Intestinal epithelial cell dysplasia and villus atrophy | Severe diarrhea, failure to thrive |
Disaccharidase Deficiencies ( Table 31-3 )
Sucrase-Isomaltase Deficiency
Congenital sucrase-isomaltase deficiency (CSID) was first described by Weijers and colleagues in 1960. It is generally considered to be a rare condition, with the homozygous condition approximating 0.2% in individuals of European descent; however, much higher incidence is found among Alaskan and Canadian natives, and incidence is as high as 5% to 10% in Greenlanders. CSID infants receiving human milk and lactose-containing formulas do not develop symptoms, but once exposed to sucrose and starch (baby juices, baby food fruits, crackers, and other starches) they often progress to severe diarrhea, diaper rash, failure to thrive, and abdominal distension. In older children, intermittent diarrhea, cramps, and gassiness are more prevalent. Symptoms, and especially starch tolerance, often improve with age, and the correct diagnosis may be missed for years, frequently attributed to toddler’s diarrhea or irritable bowel syndrome. In older subjects, studies have identified those who present with dyspepsia, vomiting, and flatulence rather than the classic watery diarrhea and poor growth. In several studies that examined disaccharidase levels from intestinal biopsies, sucrase deficiency was found in 11% to 13% of biopsy specimens, suggesting that CSID may be much more common than previously thought. Treatment includes avoidance of sucrose or maltose. More recently, sacrosidase, marketed as Sucraid, has been approved for the treatment of CSID, which normalizes symptoms and stool frequency. Typically, doses are split, with half given at the onset of a meal and half at the midpoint. The current recommendations are also to perform skin tests on patients with asthma before initiating sacrosidase administration.
Disorder | Gene Inheritance | Malabsorbed Substrates | Suggested Mechanism of Malabsorption | Clinical Features | Ref(s) |
---|---|---|---|---|---|
Malabsorption of Carbohydrates | |||||
| SI |
| Impaired digestion of disaccharide and starch secondary to enzyme deficiency on the brush border membrane | Acidic diarrhea proportional to ingested carbohydrate | |
|
| Lactose | Impaired digestion of disaccharide secondary to enzyme deficiency on the brush border membrane | Acidic diarrhea proportional to ingested carbohydrate | |
|
|
| Selective defect in glucose and galactose sodium cotransport system | Severe diarrhea and metabolic acidosis, especially during neonatal period | |
|
| Fructose | Impaired transport of fructose across the brush border membrane | Diarrhea, bloating | |
|
| Trehalase | Impaired digestion of disaccharide secondary to enzyme deficiency | Acidic diarrhea and bloating may follow mushroom ingestion | |
|
|
| Transport defect of monosaccharides in the basolateral membrane of enterocytes as well as in liver and kidney | Carbohydrate malabsorption, tubular nephropathy, hepatomegaly, failure to thrive, and fasting hypoglycemia | |
Malabsorption of Lipids | |||||
| PNLIP | Lipids | Impaired luminal lipid hydrolysis in the duodenum | Severe steatorrhea without failure to thrive | |
|
| Lipids | Dysfunction in MTTP causes impaired apolipoprotein B processing and secretion | Steatorrhea, failure to thrive, low fat-soluble vitamins. Low LDL, apoB, and triglycerides | |
|
| Lipids | Impaired production of apolipoprotein B | Steatorrhea, failure to thrive, low fat-soluble vitamins. Low LDL, apoB, and triglycerides | |
|
| Lipids | Defect in chylomicron transport from the ER to the Golgi in enterocytes | Steatorrhea, failure to thrive, low fat-soluble vitamins. Normal triglycerides |
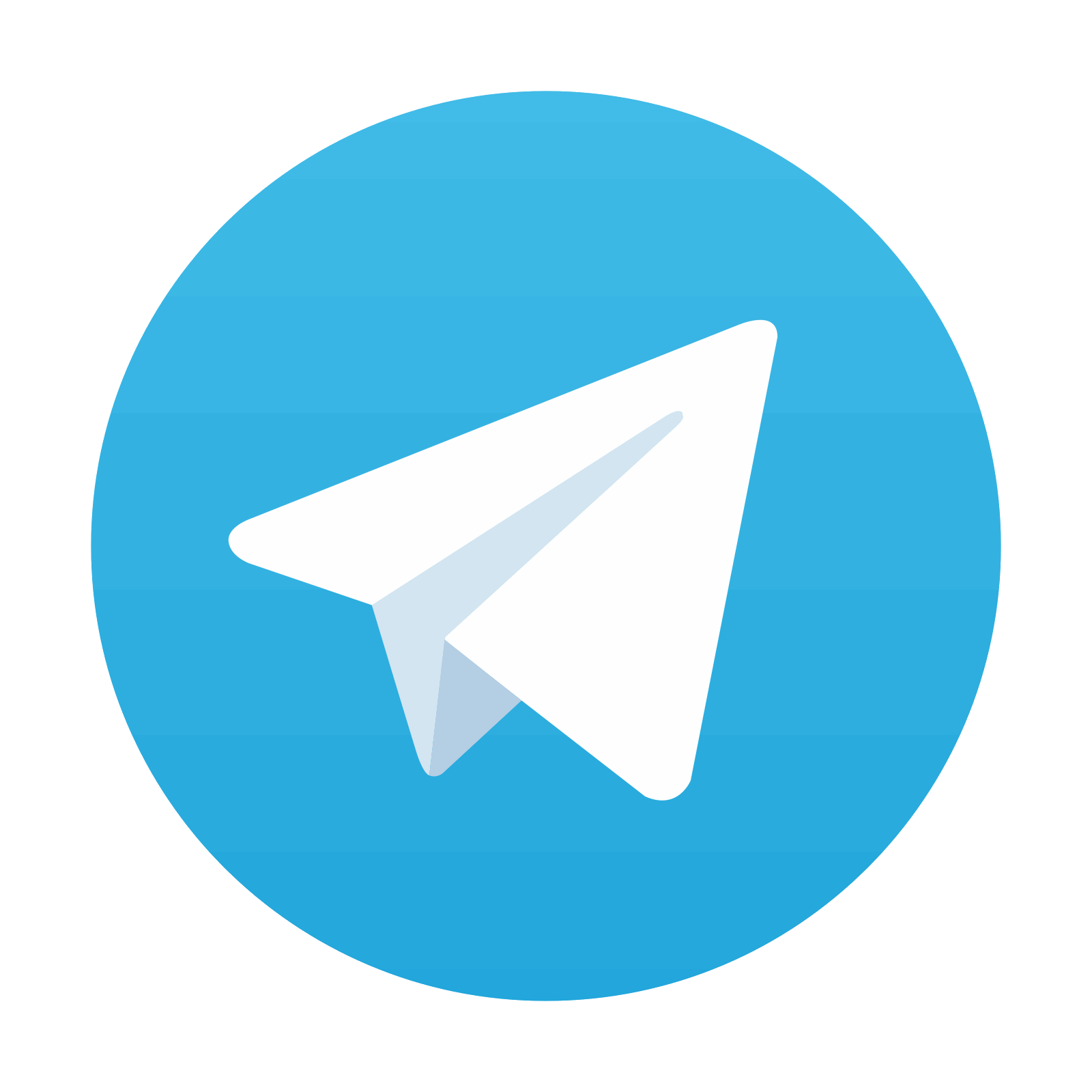
Stay updated, free articles. Join our Telegram channel

Full access? Get Clinical Tree
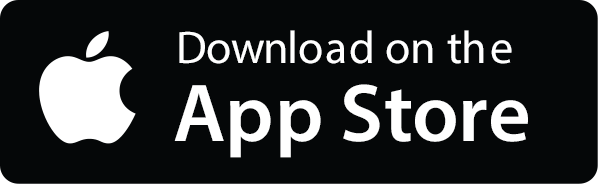
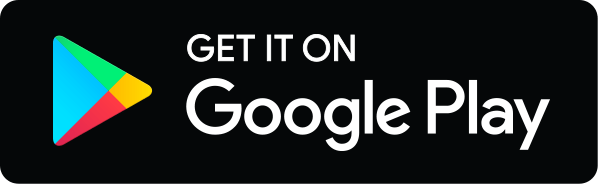
