Fig. 3.1
Schematic overview displaying time-domain, low-coherence interferometry which can measure echo time delays of light. (a) A Michelson-type interferometer is equipped with a sample and reference arm. Light which is back-reflected from mirror 1 interferes with light travelling a known reference path length (mirror 2). The reference arm is mechanically translated in order to produce a time-varying path length difference between both arms. Using a low-coherence light source, interference will only be observed when light from the tissue arrives nearly at the same time as light from the reference arm. Axial (depth) scan information is obtained by detecting the interference signal. An image is formed by colour-mapping the amplitude of adjacent axial scans in a 2D or 3D representation. (b) Clinical usable OCT systems are equipped with fibre optics which are, in the case of bladder OCT, interfaced with a fibre optical scanning probe (c) that fits through the working channel of a cystoscope (black inset, Courtesy of Dr. Jörg Schmidbauer)
Endoscopic OCT
The development of optical fibres for light transport in telecommunication made fibre-based OCT systems possible. This has led to the development of ultrathin fibre-based sample arm devices that allowed endoscopic integration. Small OCT probes were first and mainly developed for cardiovascular applications, allowing visualisation of cardiovascular plaque, thrombi or stent placement. More recently, this resulted in the commercial development of disposable in vivo OCT probes with a diameter less than 1 mm which are already used in several medical settings, i.e. to be used in endoscopes or in combination with 16G/18G needles to access internal tissue. However, these probes are mainly developed for circumferential (lighthouse-like) scanning and are therefore excellent for imaging tubular tissue. This makes them less usable for imaging the bladder where forward scanning, covering the same field of view as a regular cystoscope, is more preferable. Forward scanning has been developed using small moving mirrors on the tip of a fibre. A clinical applicable OCT system employing forward-looking OCT technology has been developed by Imalux known as the Niris® Imaging System. This system employs a 2.7 mm-diameter imaging probe (Niris® Optical Imaging Probe). The Imalux Niris system has been used in most bladder cancer studies. However, novel designs are researched to reduce the size, which is currently the limiting factor for scope integration.
Functional OCT
Clinical usability of OCT images depends on obvious factors such as high resolution, high imaging speed and adequate contrast to discriminate between benign and malignant tissues. Contrast in OCT is caused by spatial differences in refractive index of different tissue constituents, e.g. contrast originates from reflection of different structures. It is known that the refractive index is proportional to the density of the cells and cell structure. Because malignant cells display an increased number, larger and more irregularly shaped nuclei with a higher refractive index and more active mitochondria, OCT images are expected to be different in malignant tissue compared to normal and benign tissue [1]. These differences can be elucidated from the light scattering properties [2] that are measured from the signal decrease with depth from OCT images, which is quantified by the attenuation coefficient μ oct. Studies have shown that quantitative measurement of μ oct allows in vivo differentiation between different tissue types. An animal study by Xie et al. [3] showed differences in scattering properties between normal and cancerous urothelium. Similar studies on lesions in the kidney [4], vulva [5], oral tissue [6] and lymph node metastasis [7] confirmed the ability of OCT to distinguish tissue types based on μoct. However, initial studies on ex vivo human bladder urothelial biopsies showed that factors typical for ex vivo settings (e.g. cauterisation of bladder tissue specimens) on μoct-based grading of human bladder cancer were inconclusive but indicative of the need for in vivo evaluation [8].
OCT in Bladder Cancer: State of the Art
Literature Survey on Animal Studies
The feasibility of OCT on urothelium has been investigated in a variety of animal models. The potential of OCT as a diagnostic tool was demonstrated in rabbit [3] and porcine bladders [9]. OCT in the porcine bladder, a close homolog to the human bladder, showed the ability to image the anatomical layers of the porcine bladder including the urothelium, lamina propria and muscularis propria. The first systematic study of OCT in urological tumours was performed on bladders of Fischer rats that were exposed to methyl-nitroso-urea, a standard rat model for bladder cancer studies. In this rat bladder cancer model, edema, inflammatory infiltrates, submucosal blood congestions and abnormal growth of urothelium were seen [9].
Although the first animal studies of OCT in urology showed the high potential of OCT as a diagnostic tool in urothelial carcinoma, they also showed the major technical limitations of the OCT systems that had to be overcome before introducing the technique in a clinical setting. An in vivo setting in the rabbit bladder using transverse OCT imaging probe indicated two problems using this probe. First, since the bladder is a relatively large and irregularly shaped organ and the catheter used was designed to have a fixed working distance, the bladder wall was predominantly displaced from the focal plane during imaging, which resulted in relatively low transverse resolutions, as the distance from the imaging probe to the wall did not remain constant. Second, since the number of transverse pixels is constant, over a larger circumference, the lateral imaging resolution decreased as the size of the pixel increased. These two results emphasised that redesign of the imaging probe was mandatory [3].
Literature Survey on Humans Ex Vivo
After the feasibility of OCT was demonstrated in cardiology and ophthalmology, OCT was applied for the first time on human urological tissue in 1997 [10]. OCT measurements were performed on normal bladder tissue obtained from autopsy. Ex vivo was seen that OCT is able to delineate the microstructure of the normal human bladder wall. Anatomical layers in the human bladder, including the urothelium, lamina propria and muscularis propria, can be identified (Figs. 3.2 and 3.3) [3, 10, 11].
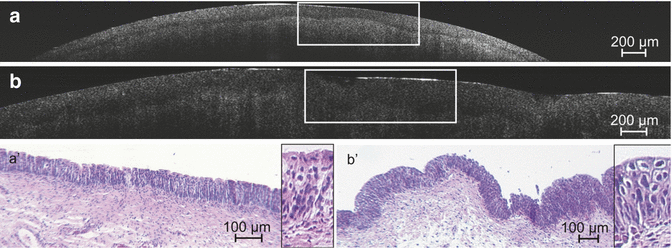
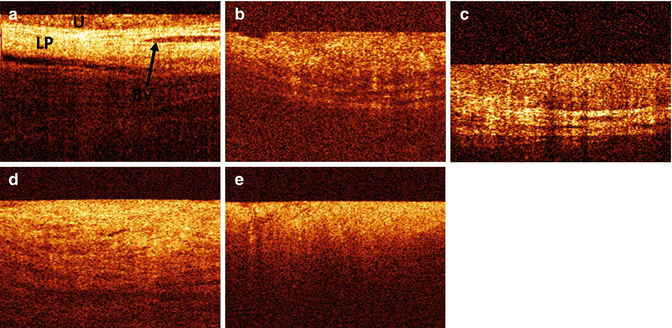
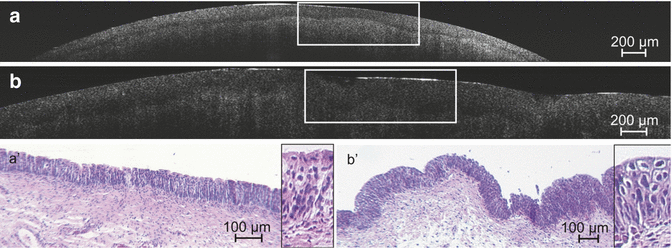
Fig. 3.2
OCT bladder pathology correlated with pathohistology (Courtesy of Prof. R. Knüchel). Correlation of ultrahigh-resolution OCT B-scans of healthy tissue (a) and carcinoma in situ (CIS) (b) with histological findings (a′ and b′). Histological sections (haematoxylin-eosin staining) represent the marked areas within the corresponding OCT images. The insets in (a′ and b′) represent magnified views of the urothelial layer of the tissue. OCT representation of healthy tissue (a) and CIS (b) both demonstrate a continuous dark layer in between the higher scattering urothelium and stroma representing the basement membrane zone. The urothelium illustrated is very homogeneous for healthy tissue (a), which is not the case for CIS (b) in which the tissue appears coarse grained and inhomogeneous
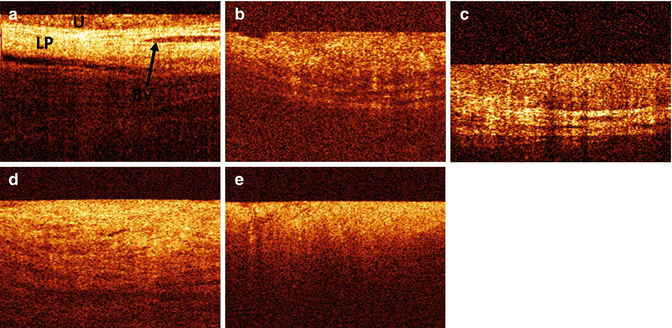
Fig. 3.3
OCT bladder pathology. (a) Normal bladder lining which is characterised by high contrast and a stratified structure. The urothelial (U) layer is visible as an upper dark layer, while the second bright layer is the lamina propria (LP). Blood vessels (BV) are visible as dark structures in the lamina propria. (b) Carcinoma in situ (CIS) is recognised by its low contrast compared to normal-appearing OCT bladder images. The urothelial and lamina propria layer appears to be more bright. The horizontal structures of the anatomical layers are still recognisable. (c) Ta carcinoma of the bladder is recognised on OCT image as thickened upper urothelial layer, clearly distinguishable from the second bright lamina propria layer, showing no signs of invasion. (d) T1 carcinoma of the bladder. Horizontal structures are losing contrast resulting in indistinguishable urothelial and lamina propria layer. The muscularis is still visible. (e) T2 carcinoma of the bladder. OCT image of a T2 tumour showed tumour extending into the muscle indicated by the total loss of horizontal structures (Courtesy of Dr. Jörg Schmidbauer)
The capability of OCT in distinguishing between different anatomical layers is based on the different back-reflection characteristics of each layer [11]. The urothelium is slightly lower scattering, and therefore higher and more regular back-reflection intensity is seen. This gives the urothelium a darker appearance on OCT images compared to the underlying lamina propria. Secondly, OCT is capable to identify capillaries within the submucosa in normal human bladder tissue and vessels in the bladder wall [3]. Unlike animal carcinogenesis models, the first preliminary human studies showed that bladder cancers in humans are more complicated in terms of urothelial backscattering. Tumorigenesis induces complex surface condition changes resulting in enhanced backscattering in some lesions and reduced backscattering in others. Nevertheless, promising results can be provided when incorporating other diagnostic parameters such as urothelial heterogeneity, visibility of anatomical layers and vascularisation changes [12]. The anatomical microstructure of the bladder could no longer be visualised in ex vivo bladders of patients suffering muscle-invasive bladder cancer (Fig. 3.3) [3]. However, due to the limited axial resolutions to about 10 μm of the standard OCT systems, a valid decision of invasion and the assessment of urothelial dysplasia are not possible.
The standard OCT systems are very well able to visualise the urothelium, lamina propria and muscularis propria, and the integrity of the basement membrane cannot be reproduced because of restricted resolution power of such systems [11]. Ultrahigh resolution OCT, however, is able to visualise the basement membrane zone in healthy and diseased bladder wall tissue by providing high-resolution OCT images [11]. The basement membrane zone is visualised as a thin layer showing a minimum of signal intensity between the moderately scattering urothelium and the higher scattering lamina propria. In tissue samples of invasive bladder tumours, the basement membrane appears no longer as a continuous signal-free layer but shows interruption in ultrahigh resolution OCT images [11]. This latest advantage gives OCT the potential to give real-time information on tumour stage.
An initial study on μoct-based grading of bladder cancer in bladder tissue specimens collected during transurethral tumour resection (TURT) was inconclusive due to typical factors for ex vivo settings [8]. Cauterisation of bladder tissue specimens after transurethral resection, orientation of the biopsies and the effect of tissue relaxation of the biopsies are all factors that reflect limitations due to ex vivo circumstances. This outcome indicates the need for in vivo evaluation of OCT as a diagnostic tool for both grading and staging in bladder cancer.
Literature Survey on Humans In Vivo
Since the bladder is a relatively large and irregularly shaped hollow organ, OCT measurements are hampered as was seen in vivo animal studies. The first step in implementation of OCT in hollow organs was endoscopic implementation to provide a reliable and convenient access of probing OCT to the surface of the urinary bladder. However, this first step included several optical, engineering and biomedical aspects such as creation of an OCT interferometer with a flexible arm, development of miniaturised lateral scanning probe with a remote control and acquisition of OCT data in parallel with common endoscopic imaging. In 1997 the first OCT imaging probe was successfully developed with endoscopes by integrating the sampling arm of an all-optical-fibre interferometer into standard endoscopy using their biopsy channel to transmit low-coherence radiation to the tissue, providing direct access of OCT to the bladder wall possible in vivo [13]. To probe the surface of the urinary bladder, a miniaturised electromechanical unit was developed, controlling the lateral scanning process by terminating the sampling arm of the fibre interferometer [13]. Polarisation fading due to polarisation distortions occurring by bending the endoscopes arm was avoided by using polarisation maintaining fibres to transport the light. This first endoscopic integration resulted in an endoscopic OCT system with a central wavelength of 830 nm, an image depth of 3 mm and a scanning rate of 30 cm/s and finally in an OCT picture of 200 × 200 pixel size in approximately 1 s.
Results on application of this new OCT device were compatible with ex vivo results; due to the different backscattering intensity of each different anatomical layer, they could be identified. Like in ex vivo settings, the urothelial layer was seen as a weakly scattering layer, while the lamina propria was seen as a bright layer with the highest scattering intensity. In the lamina propria, vessels could be identified. The muscularis propria was identified as a less scattering layer beneath the lamina propria [1, 13, 14]. Benign conditions of the bladder such as cystitis were seen as edematous thickened urothelial layer and thickness of the lamina propria. In case of prolonged chronic cystitis, sclerosis and fibrosis was seen as increased backscattering from these structures, combined with increased vascularisation of the bladder wall [1]. In chronic cystitis in children, typical accumulation of fluid under the urothelial layer was seen on tomograms [1, 14]. Also bladder wall inflammation and von Brunn’s nests were correctly diagnosed using OCT [15].
Secondly, a higher homogeneity and typically higher urothelium backscattering characterised the OCT images. The latter resulted in a decreased depth of optical imaging, because of the strong scattering in malignant cells. Finally, a higher vascularisation degree was found in tomograms of cancerous tissue [13].
Studies on sensitivity and specificity of OCT on real time classifying bladder lesions as benign or malignant show high overall sensitivity of 100 %, overall specificity of 89 %, positive predictive value of 75 %, negative predictive value of 100 % and an accuracy of 92 % [15]. Positive predictive value for invasion into the lamina propria was 90 % [15]. Other in vivo studies showed similar results on sensitivity, specificity, positive predictive value, negative predictive value and accuracy in staging urothelial cancer of the bladder [11, 15–18] (Table 3.1) but also suggest that disruptions of the bladder wall from erosion, scarring or granuloma could result in false-positive results [15, 16]. In addition, for large tumours with extensive broadened urothelium, tumour depth will transcend OCT imaging depth and compromise the staging ability of OCT. This problem may be overcome by applying OCT to the tumour base [14].
Table 3.1
Diagnostic accuracy of OCT on the bladder urothelium
Author | Stage of Research | Sensitivity | Specificity |
---|---|---|---|
Hermes et al. [11] | 142 ex vivo human bladder biopsy specimens | 83.8 % | 78.1 % |
Manyak et al. [15] | OCT cystoscopically | 100 % | 89 % |
Goh et al. [16] | OCT cystoscopically | Ta 90 %, T1 75 %, T2 100 % | Ta 89 %, T1 97 %, T2 90 % |
Sengottayan et al. [18] | OCT cystoscopically | Ta 90 %, T1 75 %, T2 100 % | Ta 89 %, T1 97 %, T2 90 % |
Ren et al. [17] | OCT cystoscopically | 94.40 % | 81.3 % |
Karl et al. [19] | OCT cystoscopically | 100 % | 65 % |
One study showed a limited specificity of 65 %, probably due to a learning curve [19]. However, these results are very promising; a small study population limits them. Despite these limitations, emerging OCT with WLC increases the positive predictive value and negative predictive value of WLC dramatically [17], and a combination of these two techniques could possibly be helpful in the discrimination between cancer and benign lesions of the bladder wall.
< div class='tao-gold-member'>
Only gold members can continue reading. Log In or Register a > to continue
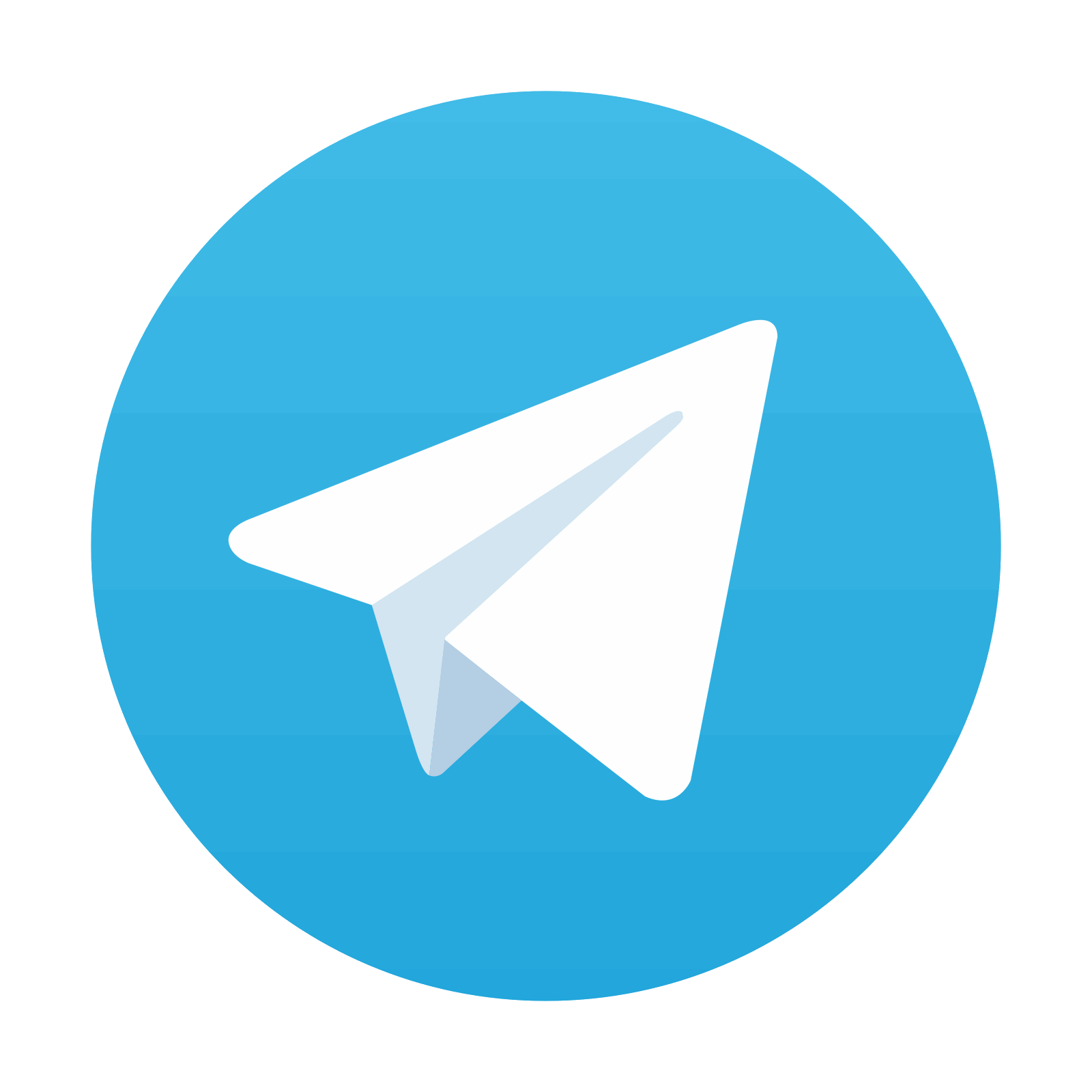
Stay updated, free articles. Join our Telegram channel

Full access? Get Clinical Tree
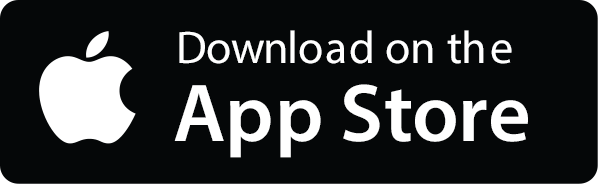
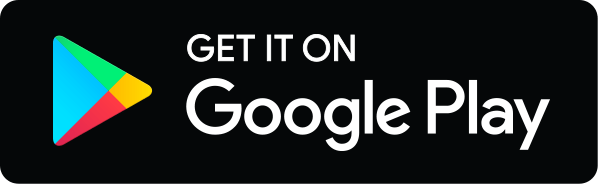