Imaging modality
Objects visualized, scale and resolution
Detail of objects visualized
Energy source
CT and MRI
Whole organs
Bone, organs, fat, air (gross level)
X-ray (for CT) or radiofrequency EM waves (for MRI)
Ultrasound
Whole organs
Soft tissue, fat, fluid (macroscopic level)
Sound waves (2–30 MHz)
Penetration (cm)
Resolution (150 μm)
OCT
Tissues
Tissue layers, architecture and structural organization (mesoscopic level)
Near-infrared light (1,300 nm)
Penetration (2 mm)
Resolution (10–15 μm)
Given its ability to provide real-time, high-resolution images, OCT has emerged as a promising imaging modality because of its ability to function as a type of “optical biopsy,” yielding information on tissue pathology in situ and in real time, without the need for excision of specimens and processing as in conventional biopsy and histopathology [4, 5]. In addition, OCT can easily be integrated into existing surgical instruments, such as laparoscopes, endoscopes, catheters, hand-held probes, or needles [6–8]. As a result, OCT can potentially guide surgical intervention or help image tissue microstructure in situations where conventional excisional biopsy would be hazardous or impossible [5]. While initially used in the field of ophthalmology to image the retina and macula in vivo, OCT has been investigated for its roles in imaging the gastrointestinal, cardiovascular, gynecologic, skin, and nervous systems [9–14].
OCT imaging in the genitourinary system was first demonstrated in 1997, and since then, multiple studies have emerged examining its use as a tool to guide clinical decisions. Early ex vivo studies noted significant levels of correlation between OCT images and histologic architecture of urologic tissues sampled. They noted that OCT was also able to differentiate early changes in mucosal surfaces of urologic tissues such as hyperplasia, dysplasia, and frank carcinoma and thus could serve as a near surrogate for histology [15, 16]. OCT has been best studied in the realm of bladder cancer, which will be covered in a separate chapter. In this chapter, we review and discuss preclinical and clinical studies of OCT in other urologic organs such as the prostate, kidney, and testis.
Prostate OCT
Prostate cancer has remained the leading soft tissue cancer diagnosed in men in the USA. The use of OCT has been explored as a modality to help image the architecture of the prostate gland and periprostatic tissues in animal and human studies.
Regarding the imaging of periprostatic tissues, the cavernous nerve (CN) was an obvious choice of tissue to try to image using OCT owing to its close proximity to the prostate surface and importance in preservation during prostatectomy to improve postoperative sexual function. Radical prostatectomy inherently carries significant quality-of-life risks including incontinence and erectile dysfunction due to injuries to the cavernous nerves (CN). Although nerve-sparing radical prostatectomy can positively impact postoperative outcomes, there is currently no established visual modality available to conclusively identify the microscopic fibers within the neurovascular bundle (NVB) during radical prostatectomy. Improvements in real-time imaging and identification of the CNs intraoperatively via OCT could potentially aid in preventing injuries to the nerves and thus improve potentially postoperative potency and continence.
Initial studies demonstrated significant correlation of OCT findings with the histologic findings in differentiating cavernous nerves from the underlying prostate glandular architecture in small animal models (Fig. 4.1). CNs and ganglions could be differentiated using OCT from periprostatic structures such as the bladder, prostate gland, seminal vesicles, and periprostatic fat, with a unique imaging signature for each of these structures (Fig. 4.2) [3, 17, 18] (Figs. 4.1 and 4.2). In a study by Fried et al., simultaneous cavernous nerve stimulation was performed following identification of the neurovascular structures in rats to provide confirmation of the actual neurovascular bundle (NVB) imaged by OCT with promising results [3].
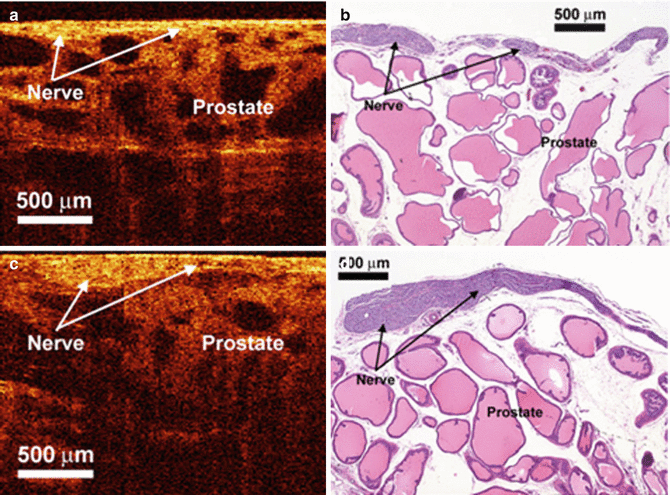
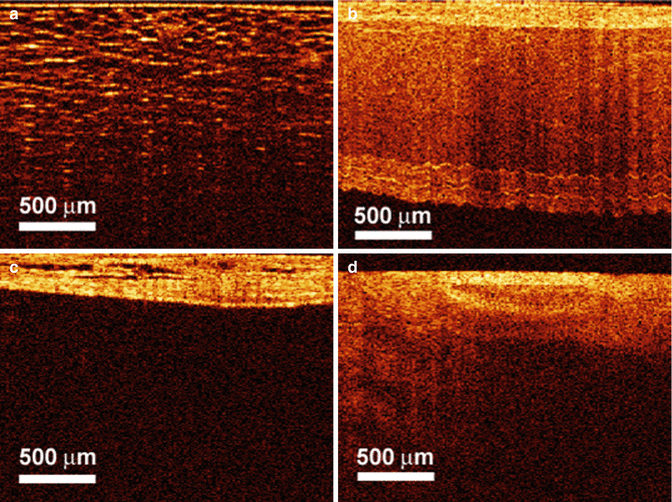
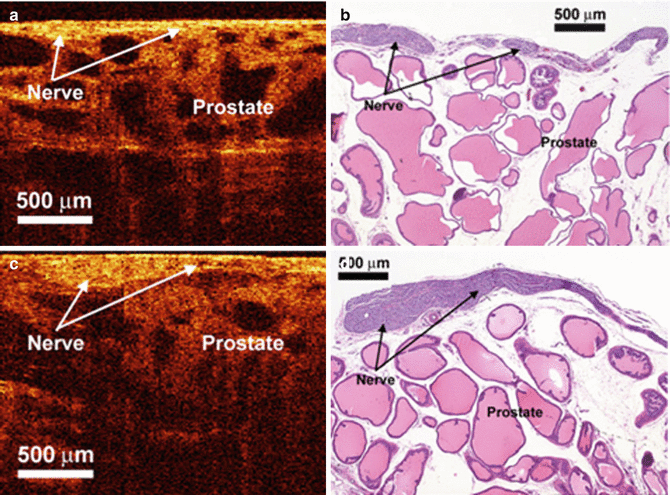
Fig. 4.1
OCT and histologic images of the rat cavernous nerve: (a, b) longitudinal section; (c, d) in each section, the cavernous nerve lies superficial to the prostatic stroma and glands and has a relatively hyperintense signal as compared to the underlying hypointense prostate glands (From Fried et al. [3], with permission)
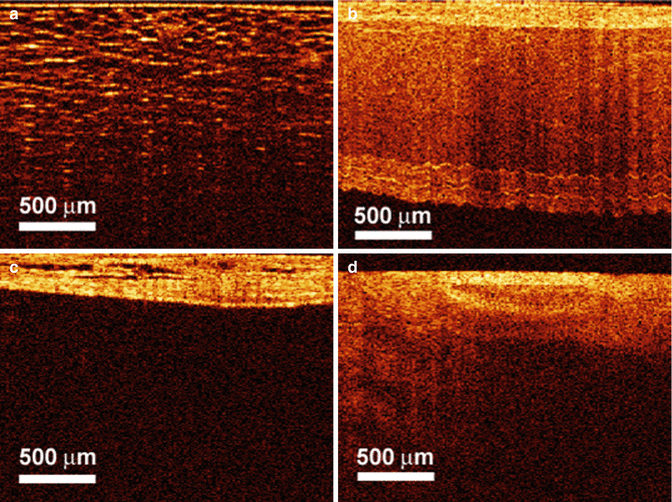
Fig. 4.2
OCT images of rat (a) periprostatic fat, (b) seminal vesicles, (c) bladder wall, and (d) periprostatic blood vessels (From Fried et al. [3] with permission)
OCT has also been studied in vivo for intraoperative use in identifying neurovascular bundles in patients undergoing nerve-sparing radical prostatectomy. In a preliminary experience by Aron et al. on 24 patients undergoing either laparoscopic or robot-assisted radical prostatectomy, OCT was applied intraoperatively in an attempt to preserve NVBs using real-time data acquisition [1]. In an attempt to evaluate the feasibility of nerve-sparing prostatectomy, specimens were examined both in vivo and in vitro for the presence or absence of the neurovascular bundles. The OCT images were found to correlate independently of the surgeon’s subjective impression of the tissue being imaged. Moreover, in four patients who underwent a wide excision of the neurovascular bundle, bench examination of the specimen proved a 100 % correlation between the OCT image and the histological examination of the prostate (Fig. 4.3). Aron and colleagues also found that identification of the NVBs required an experienced operator to distinguish them from adipose tissue, small vessels, and lymphatics [1].
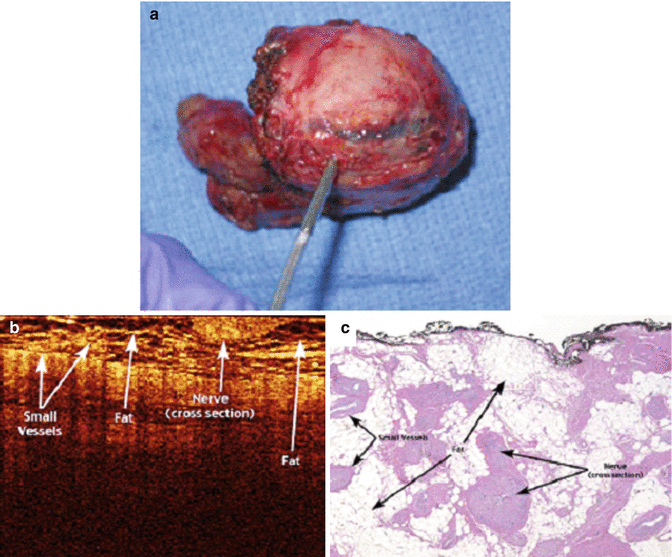
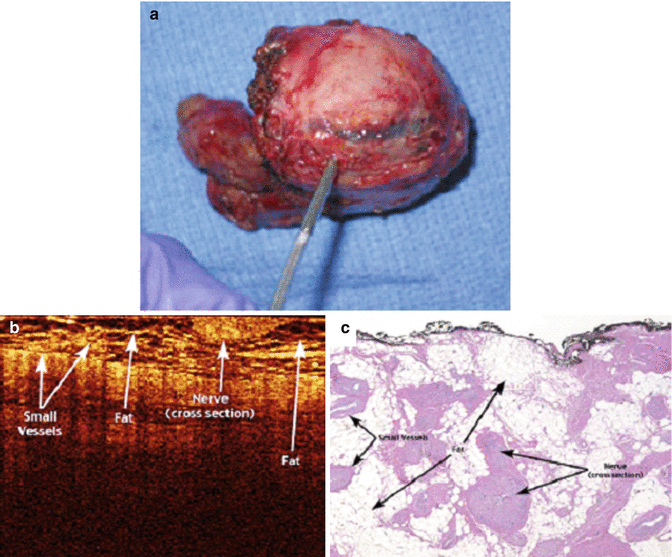
Fig. 4.3
Images of (a) human robotic-assisted radical prostatectomy specimen with NVB resected, (b) OCT imaging, and (c) histologic (hematoxylin-eosin) correlation of ex vivo human prostatectomy specimens revealing intact overlying neurovascular bundle (From Rais-Bahrami et al. [18], with permission)
Other studies noted that identification of the NVB proved to be difficult because of the presence of more periprostatic blood vessels and fat resulting in a degradation of the OCT resolution [18]. Furthermore, the limitations of OCT’s field of view make intraoperative mapping of the NVB for nerve-sparing radical prostatectomy challenging if not impractical. Thus, the ability to apply OCT technology for the purposes of directing cavernous nerve preservation remains to be determined.
The use of OCT has also been applied to prostate cancer treatment. Historically, approximately 85 % of patients with American Joint Commission on Cancer (AJCC) staging pathologic Stage T2 disease remain without clinical evidence of disease 15 years after radical prostatectomy [19–21]. The reported estimate of 10-year prostate-specific antigen (PSA) failure-free survival, however, declines to 82, 42–54, 43, and 0 % for those surgically treated patients found to have focal or established extracapsular extension, established extracapsular extension, seminal vesicle invasion, and positive pelvic lymph nodes, respectively [22]. Oncological outcomes following prostatectomies have also been linked to positive surgical margins. Despite advances in diagnostic imaging, however, it is still not possible to visualize the extent of microscopic disease reliably before or during definitive local therapy [21].
OCT has consequently been evaluated for potential use in evaluating positive surgical margins and extracapsular extension. Dangle et al. correlated OCT images of post-prostatectomy ex vivo specimens to detect positive margins and found a sensitivity, specificity, and negative predictive value of 70, 84, and 96 %, respectively [23]. They noted the low positive predictive value for detecting positive margins could be due to the heterogeneous appearance of the tumor and the low depth of penetration (2–3 mm) with OCT. Based on the high negative predictive value, however, they concluded that OCT could be useful to rule out positive surgical margin, extraprostatic extension, and seminal vesicle invasion.
OCT has also been applied to help differentiate benign from malignant microscopic structures in the prostate gland. In their study, D’Amico and colleagues obtained three to six samples from radical prostatectomy specimens of seven men with localized prostate cancer [21]. These specimens were first imaged with OCT and then sent for histopathologic examination. The investigators found that structural architecture on the order of 50–150 μm within benign glandular epithelium, fibroadipose tissue, and malignant glandular epithelium could be resolved to a depth of 0.5 mm using OCT. Thus, as in other urologic organ systems, OCT functioned as a type of “optical biopsy” to provide ultra-high-resolution images of tissue architectural morphology without the need to excise and process a specimen as in conventional biopsy and histopathologic analysis. Regions within the prostate identified as suspicious for prostate cancer based on OCT could then be preferentially sampled. The major technical limitation noted in this study, however, was that the 10-μm order of resolution that was achieved was limited to a depth of just 0.5 mm.
Kidney OCT
OCT has been applied in evaluating kidney morphology and renal masses incidentally discovered by CT or MRI. OCT was initially investigated in kidneys of rats both ex vivo and in vivo. Initial studies demonstrated that OCT was able to correctly visualize structures including the renal capsule, the renal parenchyma, and surrounding tissues (Fig. 4.4).
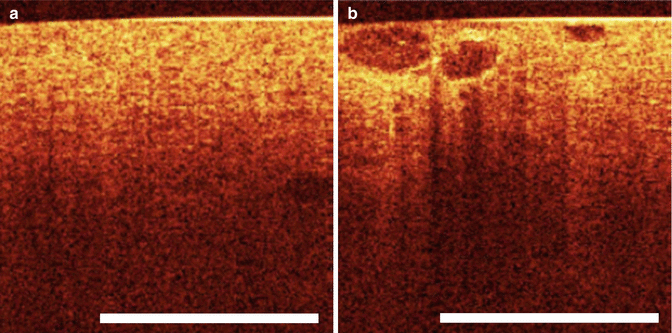
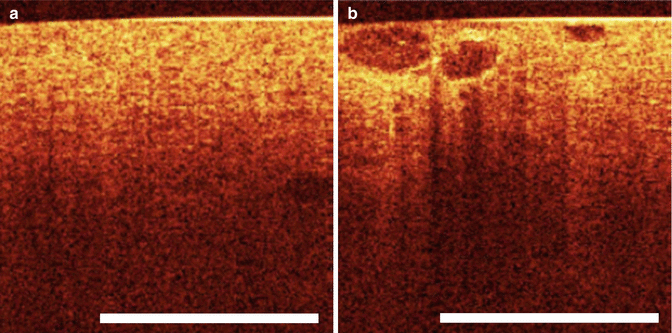
Fig. 4.4
(a) OCT of normal renal parenchyma. (b) Optical coherence tomography image of heterogeneous well-defined structures of renal parenchyma histologically proven to be renal cell carcinoma (From Goel and Kaouk [40], with permission)
In addition, OCT was able to show histopathologic alterations to renal morphology in samples that had been subjected to ischemic or nephrotoxic insults [24–26]. When renal ischemia was induced, for example, OCT revealed dramatic shrinkage of tubular lumens, diminished flow of filtrate through nephrons, and deterioration of the microvillus brush-border lining the proximal tubules [25]. As a result, groups like Li et al. examined how OCT could be applied in areas such as transplant surgery to image donor kidney structures and to evaluate organ viability following physiologic insult and acute kidney injuries [27].
< div class='tao-gold-member'>
Only gold members can continue reading. Log In or Register a > to continue
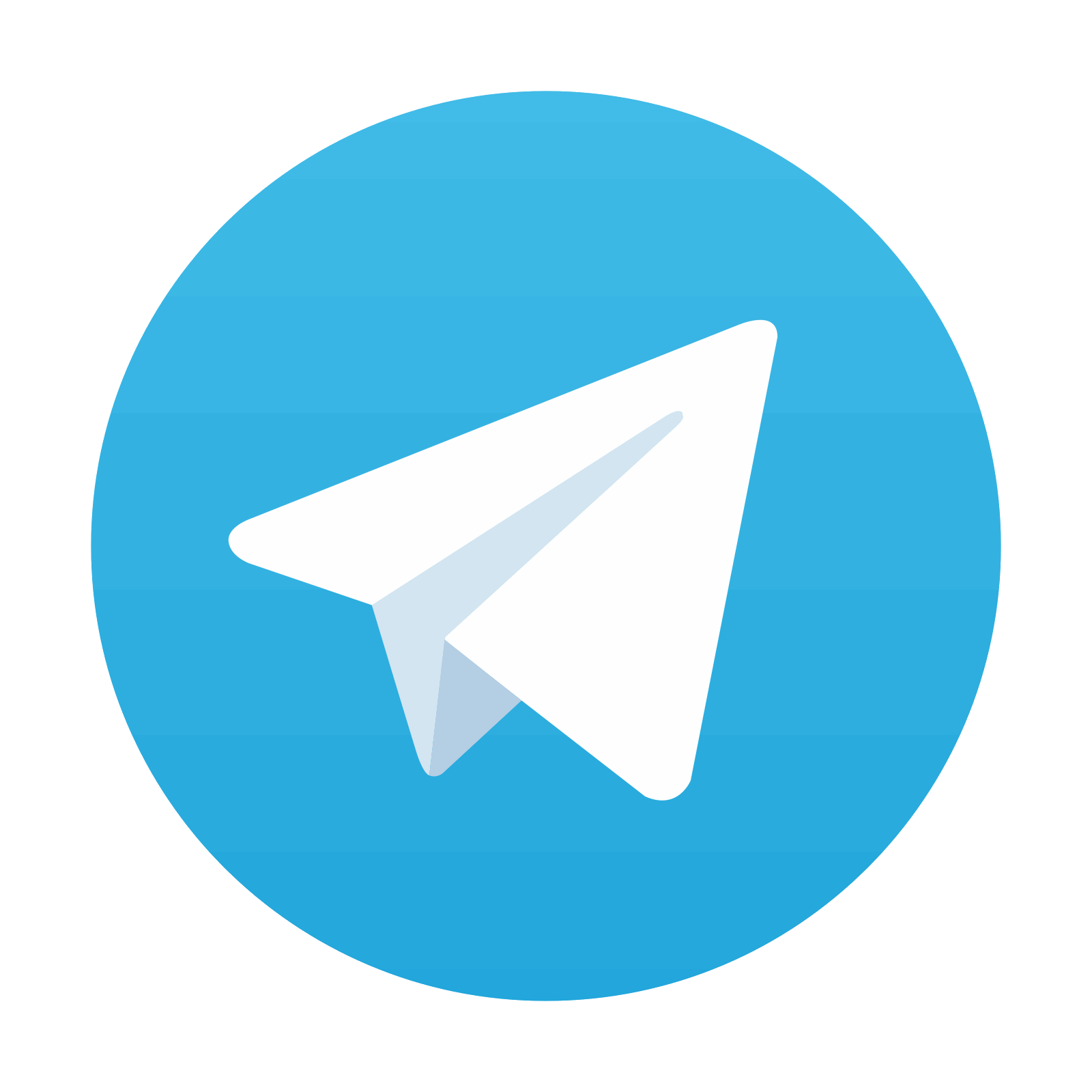
Stay updated, free articles. Join our Telegram channel

Full access? Get Clinical Tree
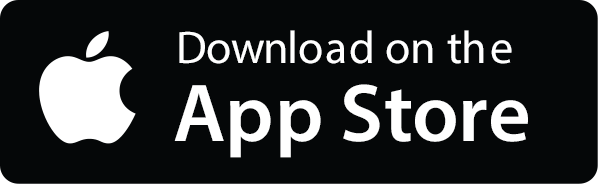
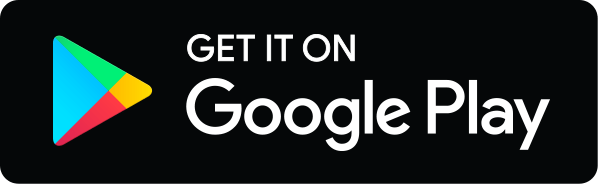