Fig. 16.1
Energy balance and body composition. FFM fat-free mass, FM fat mass, VAT visceral adipose tissue, SAT subcutaneous adipose tissue
Total energy expenditure (TEE) is divided into resting and non–resting energy expenditure. Resting energy expenditure includes the basal metabolic rate (BMR), also termed basal energy expenditure (BEE), and the thermic effect of food (TEF) (Figs. 16.1 and 16.2). Non-resting energy expenditure, or activity energy expenditure (AEE), embraces programmed physical activity (PPA) and non–exercise activity thermogenesis or NEAT (i.e., the sum of spontaneous physical activity plus occupational/leisure activities) [4, 5]. BMR is the rate of energy expenditure by human body at rest while in the postabsorptive state and is a measure of energy spent for the ongoing function of the vital organs. As such, it is operative continuously and through temporal integration plays an important part in determining one’s overall energy expenditure. Equally, the BMR determines the minimum energy requirement for maintaining ongoing vital organ function. It is therefore important to know one’s BMR value before planning a diet therapy.


Fig. 16.2
TEE (total energy expenditure) in an average healthy sedentary man. BEE basal energy expenditure (or BMR basal metabolic rate), TEF thermic effect of food, AEE activity energy expenditure (PPA and NEAT)
Since energy requirements depend on the absolute and relative size of the body components (mainly skeletal muscles, organs, and skin and to a much lesser extent adipose tissue), body composition has been recognized as a more suitable basis than body weight for estimating the basal metabolic rate.
Body composition, energy balance, and biochemical laboratory data represent the main biological variables through which it is possible to investigate the nutritional status (Fig. 16.3). In undernourished people, a nutritional status evaluation helps to quantify caloric and nutrient deficits that must be addressed in an appropriate diet therapy plan. The assessment of nutritional status similarly allows us to make the diagnosis and define the severity and clinical risks of associated overweight and obesity conditions, as well as to detect the existence of a reduced muscle mass (a condition named sarcopenia), investigate the causes of weight gain, prescribe the most appropriate treatment, and assess the results of a dietetic, medical, or surgical intervention over time. Nutritional status assessment in this context goes beyond appreciating the extent of energy imbalance to quantify the excess adipose tissue or fatness (body fat percentage) and to specify its regional distribution. The amount of excess white adipose tissue and its central or peripheral location (respectively, visceral adipose tissue or subcutaneous adipose tissue) are important issues related to cardio-metabolic risk in obese-overweight people. While generalized and abdominal obesities are both associated with greater risk of morbidity and mortality, abdominal obesity in particular has been associated with an increased risk of myocardial infarction, stroke, and premature death. Increased visceral adipose tissue is associated with a range of metabolic abnormalities related to the secretion of proinflammatory cytokines produced by adipose tissue. These adipokines, and in particular IL-6 and TNF, promote a chronic low-grade inflammatory state linked to the obesity-associated metabolic diseases [6]. These alterations cause decreased glucose tolerance, reduced insulin sensitivity, and adverse lipid profiles, which are risk factors for the development of metabolic syndrome, type 2 diabetes, cardiovascular diseases (CVD), stroke, and cancer.


Fig. 16.3
Nutritional status, lifestyle, and health status relationship. Body composition, energy balance, and biochemical laboratory data represent the main biological variables through which it is possible to investigate nutritional status
The nutritional status should be always monitored in a patient with an increased cardio-metabolic risk, regardless of the body mass index (BMI) value that may be normal or increased. The same principle should also be applied in subjects with increased BMI although apparently healthy.
16.1 Body Composition
16.1.1 Body Composition Components: FFM and FM
FFM and FM are the two generic components used in models of body composition. FFM can be divided into a more metabolically active part, mainly formed by visceral organs, skeletal muscle, and skin and a part formed by the supporting tissues, bone, and body fluids whose metabolic demands are very low. Further stratification can be made as the visceral organs consume much more energy per unit of weight than skeletal muscle. The skeletal muscle component of FFM is considered to have a much lower resting metabolic rate than the visceral organs; nevertheless, it is more abundant and its mass can be modified by our lifestyle, mainly by physical activity. The FM is not metabolically inert but it certainly represents a minor component of the BMR. These considerations have led to the development of a number of models of body composition that differ in their complexity depending on how finely the subcomponents are divided and how they account for the influence of the varying proportions of the different components of FFM and/or tissue-specific metabolic rates. Validation and use of these models in the diagnosis and prognosis of obesity and its associated comorbidities require knowledge of the percentage of FM, the relative proportion of FFM and FM, and the body composition of specific segments of the body like the trunk and limbs. Various in vivo field and laboratory methodologies have been developed to estimate or measure the compartments that represent the different constituents of the human body [7, 8]. Both these aspects of nutritional status evaluation are discussed below.
16.1.2 Body Composition Models
The information obtained from complete dissections and analyses of the composition of human cadavers has led to the establishment of reference values; the development of the concept of the Reference Man [9] provides the bases for developing various models of human body composition.
In the basic two–compartment model, the body is divided into the fat mass that comprises body fat and the fat-free mass, including all the remaining tissues. Dividing the FFM into a lean tissue mass (LTM) and bone mineral content (BMC), in combination with the FM, yields a three–compartment model that is particularly interesting as it can be assessed by dual X-ray absorptiometry (DXA).
The elemental, the molecular, the cellular, the functional (or tissue model), and the whole-body models of body composition represent different multi–compartment models of progressively higher complexity. In the molecular model, the FFM is subdivided in its molecular constituents, water, proteins, minerals, and glycogen, while fat is the molecular component of the FM. The body cell mass in the cellular model is considered the metabolically active district responsible for energy consumption at rest, in the postabsorptive state, or BMR; the other components of the cellular model are extracellular fluids, extracellular solids, and fat.
The clinician is generally interested in measuring body composition in terms of FM and FFM as the bi-compartmental model of body composition is particularly well established for the diagnosis, treatment, and prognosis of diseases related to alterations of nutritional status.
16.1.3 Field Methods and Laboratory Measurements of FFM and FM
A variety of reference laboratory methods have entered use for body composition analysis, including densitometry, hydrometry, in vivo neutron activation analysis (IVNAA) [10], and DXA. Estimation equations have been compiled using values obtained by different field methods as anthropometry (weight, height, skinfold thickness, and circumference measurement) or conductance-based techniques like body impedance assessment (BIA); these estimate equations have been validated by comparison with data obtained by gold standard laboratory methods.
The different densities of the FM and FFM can be used to reexpress their mass fractions in the bi-compartmental model in terms of their relative volumes. The proportions of FM and FFM obtained from cadaver analyses have led to the creation of algorithms that indirectly estimates the percentage of body FM from body density (see Siri equation in Table 16.1). Body density in turn can be obtained by dividing body mass by body volume. While body mass is related to body weight, body volume cannot be so easily assessed. Water displacement is one possibility, but in practice, densitometry tends to rely on either underwater weighing or air-displacement plethysmography to evaluate body volume. Underwater weighing is a laboratory method that compares the weight of the subject in air to that obtained while they are entirely submerged in water. In air–displacement plethysmography on the other hand, the subject is placed in a closed air-filled chamber that is then subjected to a pressure change in order to calculate the volume of the body. Densitometry is probably the best laboratory approach to obtaining data for the two-component model for adults.
Table 16.1
Skinfold body density (BD) predictive equations
Skinfold equations | ||
---|---|---|
Durnin and Womersley [11] | n = 481 (209 M, 272 F), 16–72 years | M: BD = 1.1765 − 0.0744 × (Log SKN) |
SKN = biceps + triceps + subscapular + suprailiac | ||
F: BD = 1.1567 − 0.0717 × (Log SKN) | ||
SKN = biceps + triceps + subscapular + suprailiac | ||
n = 308 M, 18–61 years, n = 249 F, 18–55 years | M: BD = 1.112 − (0.00043499 × SKN) + (0.00000055 × √SKN) − (0.00028826 × age) | |
SKN = chest + axilla + triceps + subscapular + abdominal + suprailiac + thigh | ||
F: BD = 1.097 − (0.00046971 × SKN) + (0.00000056 × √SKN) − (0.00012828 × age) | ||
SKN = chest + axilla + triceps + subscapular + abdominal + suprailiac + thigh | ||
n = 308 M, 18–61 years, n = 249 F, 18–55 years | M: BD = 1.10938 − (0.0008267 × SKN) + (0.0000016 × √SKN) − (0.0002574 × age) | |
SKN = chest + abdominal + thigh | ||
F: BD = 1.0994921 − (0.0009929 × SKN) + (0.0000023 × √SKN − (0.0001392 × age) | ||
SKN = triceps + suprailiac + thigh | ||
Forsyth and Sinning [14] | M, 19–22 years, male athletes | M: BD = 1.103 − 0.00168 × SKNS − 0.00127 × SKNA |
SKNS = subscapular | ||
SKNA = abdominal |
Body density can be also estimated from the measurement of skinfold thickness with the use of population-specific equations (Table 16.1).
Dilution methods are based on the principle that the volume of a body compartment can be calculated as the ratio of a tracer dose dispensed orally or intravenously to its final concentration in that compartment after a certain period of time. Since on average in healthy adults the total body water (TBW) constitutes 73 % of the FFM, the latter can be easily calculated if TBW is measured. The measurement of TBW, or hydrometry, is a dilution method based on deuterium [16], a stable nonradioactive isotopic tracer of hydrogen. The value of FM can then be obtained by the difference of body mass and FFM. Similarly, extracellular water can be calculated with basic dilution techniques and a nonradioactive Br tracer administered orally.
Anthropometry–based methods are good field methods. Weight, BMI, skinfold thickness, and body circumference measurements are simple, quick, inexpensive, and portable techniques.
Body weight is more a dimension of body size than a measure of body composition. Nevertheless, weight as a function of height has an essential role in the evaluation of nutritional status in children and adults. Weight is the sum in kg of the FM and FFM. In the diagnosis of overweight and obesity, it is important to know the percentage of body FM rather than the absolute amount of fat in kg, and specialists encourage a definition of obesity based on percentage of body fat or fatness. Changes in body weight over time are more often due to changes in the amount of FM, as FFM is the most stable of the two components. Daily weight fluctuations are usually caused by variations in water content, such as in edema.
Body mass index or BMI is calculated by dividing weight (in kilograms) by height (in meters) squared. BMI is widely used as an index of the degree of obesity or undernutrition. It is better seen as a measure of fat content (FM in kg) than of fatness (% fat) however. BMI is a compositional index that has relevant clinical and prognostic value [17]. BMI values correlate with mortality rate [18] and have developed into an indicator of risk of diseases related to adiposity like premature death, cardiovascular diseases, high blood pressure, osteoarthritis, some cancers, and diabetes. It should be remembered however that although BMI has traditionally been used as a measure of body size and composition, abdominal fat mass can vary significantly within a narrow range of total body fat and BMI.
The most widely accepted classifications for overweight and obese people are those from the World Health Organization (WHO), based on BMI (Table 16.2).
Classification of overweight and obesity and associated disease risk | |||
---|---|---|---|
Class | BMI | WC | Disease risk |
Underweight | <18.5 | M: <102 cm | – |
F: <88 cm | |||
M: >102 cm | – | ||
F: >88 cm | |||
Normala | 18.5–24.9 | M: <102 cm | – |
F: <88 cm | |||
M: >102 cm | – | ||
F: >88 cm | |||
Overweight | 25–29.9 | M: <102 cm | Increased |
F: <88 cm | |||
M: >102 cm | High | ||
F: >88 cm | |||
Obesity class I | 30–34.9 | M: <102 cm | High |
F: <88 cm | |||
M: >102 cm | Very high | ||
F: >88 cm | |||
Obesity class II | 35–39.9 | M: <102 cm | Very high |
F: <88 cm | |||
M: >102 cm | Very high | ||
F: >88 cm | |||
Obesity class III | >40 | M: <102 cm | Extremely high |
F: <88 cm | |||
M: >102 cm | Extremely high | ||
F: >88 cm |
Skinfold thickness and body circumferences. Skinfold thickness is a measure of subcutaneous adipose tissue. Skinfolds themselves may be compared with reference data or used in estimation equations to derive whole-body fatness (Table 16.1).
Skinfold measurements are taken at specific sites of the body. The subscapular, triceps, biceps, and suprailiac skinfold measurements are among the most commonly used sites. After pinching the skin and subcutaneous fat tissue away from the underlying muscle, the tester uses special skinfold calipers to measure the skinfold thickness in millimeters.
Skinfold thickness has the characteristics of a good field method. It is simple and quick, and the calipers are inexpensive and portable. Since estimation equations are always specific to the population on which they have been validated (specificity concerns age, sex, ethnicity, technical origins), one must consider which of the available equations most closely fits the individual being measured. The seven- and three-skinfold equations of Jackson and Pollock [12, 13], the equations of Durnin and Womersley [11], and the equation of Forsyth and Sinning [14] are among the most used (Table 16.1).
Circumference measurement. Circumference measurement is another simple and useful anthropometric measure. The waist, hip, and mid-arm are the most frequent sites where circumferences are measured.
Waist circumference (WC) is measured at the approximate midpoint between the lower margin of the last palpable rib and the top of the iliac crest [19]. In other protocols, waist circumference has been measured at the top of the iliac crest [22], or at the level of the umbilicus or at the point of the minimal waist [23].
Hip circumference is taken around the widest portion of the buttocks.
Waist–hip ratio (WHR) is the waist circumference divided by the hip circumference.
Since waist circumference and waist–hip ratio reflect abdominal adiposity, high values for WC or WHR indicate abdominal fat accumulation [21]. They represent an additional measure of body fat distribution [24] and are a good predictor of cardio-metabolic risk [20] (Table 16.2). Unfortunately, universal cutoff points for WC, as for BMI, are not applicable as disease risk may differ between ethnic groups [19].
Mid–upper arm circumference (MUAC) is an index of nutritional status that, in conjunction with BMI, is particularly useful as a measure of chronic energy deficiency in children and hospital patients. It is highly correlated with BMI, but it does not need weight and height measuring apparatus. Used together with a triceps skinfold measurement, it is possible to obtain cross-sectional areas of adipose tissue and muscle at mid-upper arm level.
Body impedance assessment (BIA). The principle of body impedance assessment is that the resistance or impedance of the body to the flow of a weak alternating electric current (800 mA 50 kHz) is inversely proportional to the total body water (TBW). TBW composes 60 % of an adult body weight and about 73 % of the FFM of nonobese subjects [7, 25]. At low frequencies, current flows mainly through extracellular water, while at higher frequencies, it also penetrates cell membranes into intracellular water. Thus, multifrequency instruments can evaluate the extracellular and intracellular component of TBW.
The procedure of BIA involves the careful placement of the electrodes (signal and detecting electrodes) on the wrist and ankle. Simplified devices require merely that the subject stands barefooted on metal plates connected to the electrodes.
From appropriate well-validated estimation equations that include empirical impedance values and anthropometric variables such as height and weight, it is possible to calculate TBW and FFM, and hence FM. More involved BIA procedures give the possibility to estimate the composition of body segments, like upper and lower limbs.
BIA is a field body composition technique, it is quick and simple to use, and it has a good reproducibility. It is important to standardize the measurement conditions [26] and use appropriate well-validated estimation equations.
Dual X–ray absorptiometry (DXA). DXA is a method for whole–body and regional body composition analysis. DXA produces a bidimensional image of the entire human body. The principle behind DXA is in the differential degree of attenuation of X-ray beams of different energies by the soft tissues and bone. Subsequently, the procedure has been developed for non-osseous fat and lean tissue. This makes DXA well suited to describing the body in terms of bone mineral content, lean tissue mass, and fat mass, which can be used in the two-compartment model with the FFM divided into BMC and LTM. DXA technique may be used to describe the composition of the entire body or of its segments like the trunk and the limbs. Thus, DXA may be used to assess peripheral or central adipose tissue accumulation [3] or the amount of LTM in the limbs where it is mostly represented by skeletal muscle. An index obtained from the DXA has been used to diagnose sarcopenia [27].
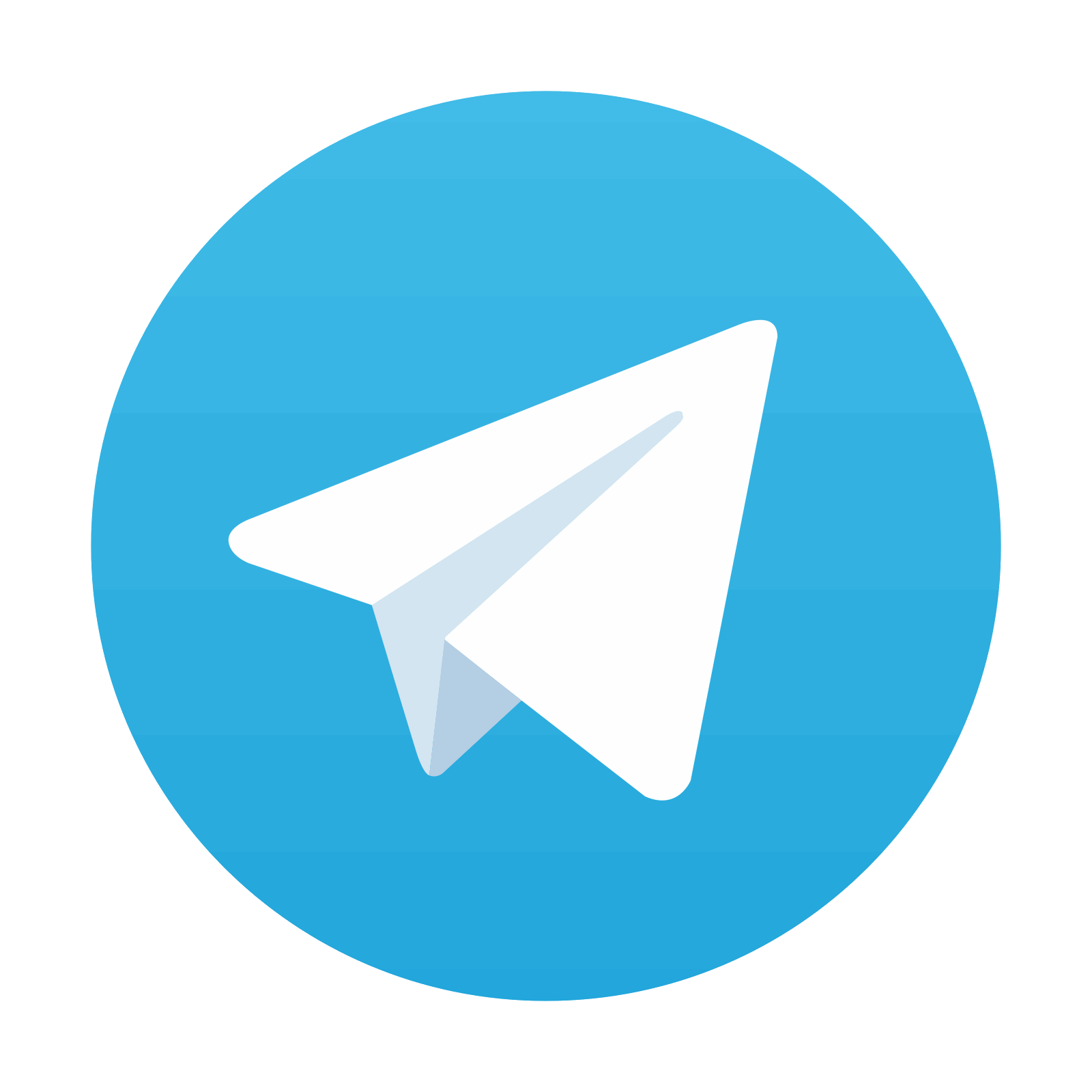
Stay updated, free articles. Join our Telegram channel

Full access? Get Clinical Tree
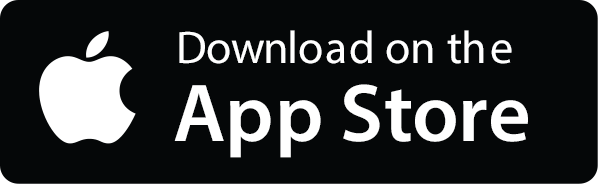
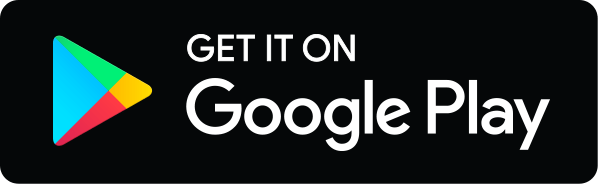