Fig. 6.1
Basic principles of multiphoton microscopy (MPM). (a) The Jablonski diagram showing how MPM fluorescence emission is caused by the simultaneous absorption of two or three low-energy (near-infrared) photons, which cause a nonlinear excitation equivalent to that created by a single photon of bluer light. (b) Two types of signals are generated by MPM: fluorescence and second harmonic generation (SHG). While fluorescence signal is broad and isotropic, SHG signal is narrow and occurs at precisely half the exciting wavelength
Most importantly, by utilizing two-photon excitation in the 700–800 nm range, MPM enables both in vivo and ex vivo imaging of fresh, unprocessed, and unstained tissue via intrinsic tissue emissions (ITEs). The ITE signal is composed of two components: (1) tissue autofluorescence, in part from reduced nicotinamide adenine dinucleotide (NADH) and flavin adenine dinucleotide (FAD) in cells (both benign and malignant), elastin in the connective tissue, and lipofuscin in fat and other cells, and (2) second harmonic generation (SHG), a nonlinear scattering signal which arises from non-centrosymmetric structures such as tissue collagen (see Fig. 6.1b) [7–9]. It has been shown that MPM/ITE imaging is capable of generating distinctive optical signals that enable imaging of animal [8, 10–14] and human [15–22] tissues at submicron resolution in three dimensions to a depth of up to 0.5 mm below the specimen surface, at acquisition rates of ~1 image/s. These imaging parameters enable detailed visualization of cellular and subcellular structures.
Potential Applications of MPM in Urologic Practice
Below, we have summarized some recent applications of MPM in the identification and diagnosis of several urological tissues. We present MPM imaging results in two types of specimens. For detailed diagnostics, we present results on ex vivo human surgical samples – either taken during cystoscopic procedures or taken after partial or radical organ resections. All these specimens were imaged without further processing, i.e., there was no fixation, sectioning, or staining of the tissue. Fresh tissue was placed on a slide or a tissue culture dish, hydrated with buffered saline, and placed directly on the microscope stage under the objective of an upright microscope.
Olympus FluoView FV1000MPE imaging system (Olympus America, Center Valley, PA) in an upright configuration was used for all MPM imaging reported here. Briefly, fresh (unprocessed and unstained) specimens were excited using 780 nm light from a tunable Ti-sapphire laser (Mai Tai DeepSee, Spectra-Physics, Newport Corporation, Irvine, CA). Three distinct ITE signals were collected using photomultiplier tubes and then color coded by using MetaMorph v7.0r4 (Molecular Devices, Sunnyvale, CA) as follows: (1) SHG (360–400 nm, color-coded red), a nonlinear scattering signal originating from tissue collagen; (2) short-wavelength autofluorescence (420–490 nm, color-coded green), originating in part from reduced nicotinamide adenine dinucleotide (NADH) and flavin adenine dinucleotide (FAD) and from elastin; and (3) long-wavelength autofluorescence (550–650 nm, color-coded blue), originating in part from lipofuscin.
Images were acquired at both low (4×/0.28NA dry objective) and high magnification (25×/1.05 NA water immersion objective) in order to assess architectural and cytological details of the tissue, respectively. The 4× objective allowed imaging up to ~0.5 mm into the tissue (imaged area in a single frame: 3.2 mm2). The 25× objective allowed imaging up to ~0.25 mm depth (imaged area in a single frame: 0.51 mm2). Scanner zooms and automated tiling functions were used to achieve a higher magnification and larger area, respectively, if needed. Typically, the collection of each image frame shown in the figures took 1–3 s, and acquisition of an entire image stack (20–40 images) took 1–1.5 min. Minor post-processing was done using Adobe Photoshop CS4 (San Jose, CA), which primarily included adjustment of intensity and color balance and occasional removal of single-photon shot noise by Gaussian blurring. However, we would like to point out that a research pathologist who was present during the imaging session was able to make correct diagnoses in most cases by just looking at the real-time images as they were being acquired (i.e., without any post-processing). Thus, while post-processing improves the quality of the images for presentation, it is not strictly necessary for rendering real-time diagnosis.
In addition to the imaging of ex vivo tissue described above, we also report MPM imaging of urological tissues (prostate and spermatic cord) in live rats under anesthesia, to document the feasibility of its use in intra-surgical contexts.
Applications for the Management of Bladder Diseases
Approximately 70 % of all newly diagnosed urothelial carcinoma present as early-stage superficial (i.e., non-muscle-invasive) disease [23, 24]. Of these superficial cancers, carcinoma in situ (CIS) is the most aggressive intraepithelial neoplasm. It is a high-grade lesion, which when left untreated will progress to muscle-invasive disease in 80–100 % of cases and cause of mortality in 39 % of cases [25–27]. White light cystoscopy (WLC) and urine cytology are current diagnostic tools for detection of CIS. Although urine cytology is positive in over 90 % of patients with CIS, it cannot determine the extent and location of the disease [28]. White light cystoscopy accompanied by histopathologic diagnosis of the TURBT (transurethral resection of bladder tumor) from the suspicious lesions is the standard of management for bladder cancer patients. However, approximately 50 % of CIS lesions are missed on WLC alone [28]. This is mainly due to flat architecture of these lesions and the lack of cellular resolution of WLC. Thus, patients with positive urine cytology but no obvious tumor during cystoscopic exam may be subjected to repeat cystoscopy and numerous repeat biopsies in order to rule out carcinoma. In fact, an unpublished analysis of all cystoscopic submissions to surgical pathology over a 2-month period at our institution revealed that ~70 % of the biopsies and ~32 % of the TURBT resections were found to be benign upon histopathologic diagnosis (Robinson et al., unpublished). Additionally, although TURBT resections and bladder biopsies are standard procedures, they are not without associated complications, such as bleeding, infection, and, for a small proportion, bladder perforation. Thus, they are currently performed in a hospital setting. Also, patients require a Foley catheter for a mean period of 1.7 days post-procedure.
MPM-assisted cystoscopy, if successful, would reduce unnecessary biopsies, thus minimizing the above discomforts and complications. Moreover, a better cystoscopy procedure that does not miss any malignant lesions and especially any flat lesions of CIS [29] means a better surveillance for the patients who have a high chance of recurrence [30]. Additionally, accurate assessment of surgical margins during radical or partial cystectomies in situ would obviate the extra time and statistical uncertainties of frozen section analysis.
Here, we show that MPM is able to identify all flat lesions of the bladder mucosa, including cystitis and CIS (Fig. 6.2), low- and high-grade papillary carcinoma (Fig. 6.3), and invasive urothelial carcinoma (Fig. 6.3), as confirmed by gold standard H&E histopathology. Some of these results have been previously published [15, 19]. One of these studies [19] reported an accuracy of 88 % in diagnosing benign and malignant lesions with overall sensitivity and specificity of 90 and 77 %, respectively. A positive (neoplastic) diagnosis on MPM had a high predictive value (94 %), and negative (benign) diagnoses were sustained on histopathology in two-thirds of cases. Cytological grade, however, was accurate only in 38 of 56 (68 %) of cases in this study [19]. However, this study included both flat and papillary bladder lesions, and the sample size of CIS lesions was small. In a recent follow-up study assessing flat lesions alone, we were able to obtain sensitivity and specificity of MPM diagnosis of 98 % and 94 %, respectively. A positive (neoplastic) diagnosis on MPM had a high predictive value (96 %), and negative (benign) diagnoses had a predictive value of 97 % (Jain et al., unpublished).
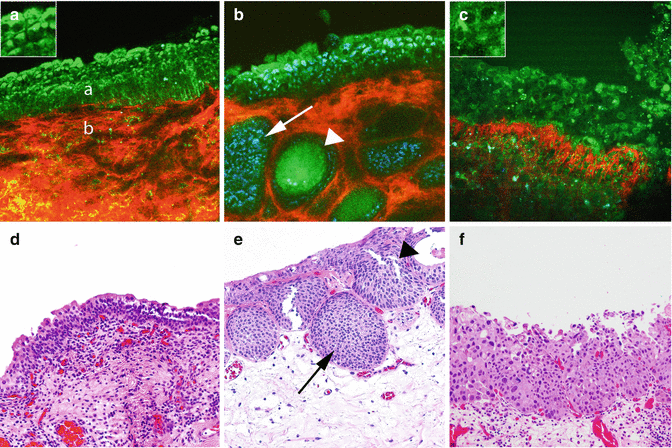
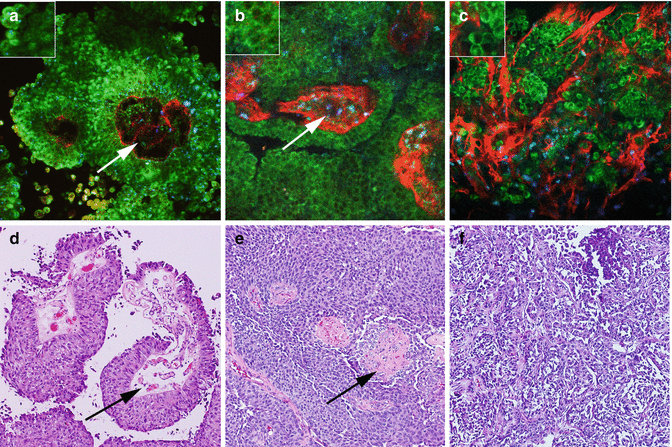
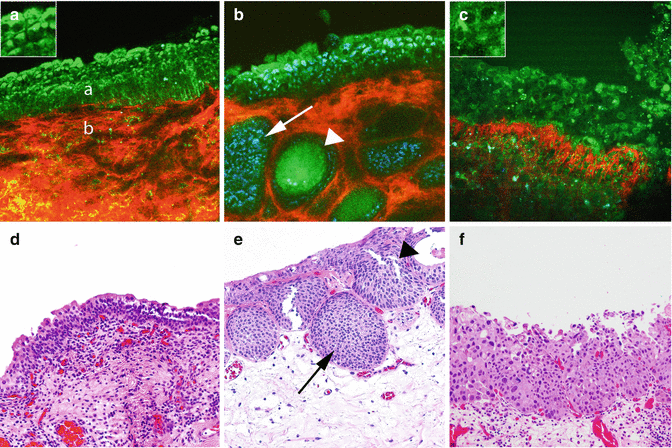
Fig. 6.2
MPM assessment of flat lesions of the bladder. MPM image (a) and corresponding H&E image (d) show a multilayered urothelium (a) with superficial umbrella cells (inset) and lamina propria (b). Lamina propria is composed of collagen bundles (red) and elastin fibers (green). The MPM image (b) and the corresponding H&E image (e) of cystitis showing von Brunn’s nests (arrows) and some with cystic dilatation (cystitis cystica; arrowhead). The MPM image (c) and corresponding H&E image (f) at high magnification show disorganized urothelium with cells having high-grade cytologic features of CIS (marked pleomorphism and increased nuclear-to-cytoplasmic ratio; arrows). Original magnifications: MPM: a–c = 300. Insets = 600×. H&E: d–f = 200×
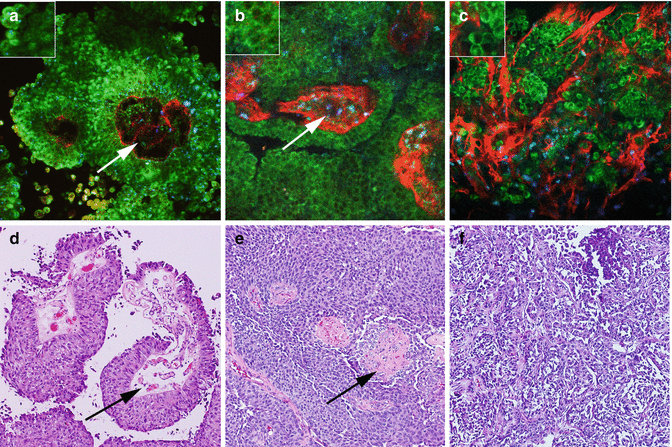
Fig. 6.3
MPM assessment of urothelial carcinoma of bladder. MPM image (a) and corresponding H&E image (d) of low-grade papillary urothelial carcinoma show papillae with fibrovascular core (arrows). Papillae are lined by urothelial cells having low N:C ratio (inset). MPM image (b) and corresponding H&E image (e) of high-grade papillary urothelial carcinoma show papillae with fibrovascular core (arrows). Papillae are lined by urothelial cells having high N:C ratio (inset). MPM image (c) and corresponding H&E image (f) of invasive urothelial carcinoma with tumor cells (color coded; green) invading stroma of lamina propria (color coded; red). Original magnifications: MPM: a–c = 300. Insets = 600×. H&E: d–f = 200×
Applications for the Management of Prostate Cancer
Prostate cancer is the most common male cancer. When confined to the gland, it can be successfully treated by radical prostatectomy. However, the success of this surgery is judged by three measures: (1) complete removal of the cancer-harboring glands, (2) preservation of nerves that control sexual function, and (3) preservation of sphincteric structures, which maintain urinary control. Anatomically, the prostate gland is surrounded by a fibrous capsule, with a thickness that varies significantly in different parts of the gland. This incomplete capsule, in turn, is surrounded by a multilayered, anatomically complex fascia that contains fat cells intermingled with loose areolar tissue, autonomic ganglia, neural plexus, nerve trunks, arteries, and veins. This fascia adheres to the capsule and is pierced by vessels and nerves. Both the erectile nerve, which has a variable branching pattern, and the sphincteric sling are intermingled with the fascial fat, connective tissue, and blood vessels.
Cancer cells sometimes migrate beyond the gland and either involve the surrounding nerves or grow into the sphincter – a phenomenon termed extra-prostatic extension (EPE). Since these nerves and any clusters of cancer cells are too small to be visualized by eye or using the 10–12× magnification of the stereoscope of a surgical robot, the competing goals of cancer extirpation versus preservation of potency and continence have to be balanced during surgery [31]. Surgeons make judgments based on information that includes presurgical PSA levels, MRI results, digital rectal examination results, and intra-surgical subjective cues such as color changes, fibrosis, and the ease of separating the different fascial layers. One option for more detailed feedback, intraoperative frozen sections, takes time, may damage the delicate structures one is trying to save, and suffers from sampling errors. As a result, the current methods for discriminating between EPE and the tissues that need to be preserved during surgery are imprecise and impact the outcomes of this surgery.
Indeed, this inability to identify cancerous cells and their association with nerves can result in incomplete removal of cancer, resulting in positive surgical margins (10–40 % occurrence) [32– 35], postoperative impotence due to the damage to or excision of these nerves (25–70 %) [36–40], or both positive margins and an impotent patient. It has been estimated that half the patients who undergo radical prostatectomy require postoperative treatment for erectile dysfunction. In addition to causing psychological and quality-of-life compromises, these complications exact a staggering financial cost on the US healthcare system. In addition, certain patients are excluded as candidates for nerve-sparing surgery based on the less-than-optimal preoperative assessments and thus may be denied a chance for a better postoperative quality of life.
In summary, access to high-resolution, high-contrast live imaging of the prostatic capsule, apex, sphincter, and the surrounding nerves and tissues would improve surgical decision-making and patient outcomes and reduce the emotional and financial impact of postsurgical complications.
Here, we show that MPM is able to correctly identify all surgically relevant components of the periprostatic tissue (Fig. 6.4) and the prostate gland (Fig. 6.5), including the ability to distinguish between benign and malignant glands (Fig. 6.5). Some of these results have been previously published [17, 41]. Additionally, in a recent study, we utilized MPM imaging in live anesthetized Sprague-Dawley rats with minilaparotomies to expose one lobe of the prostate (Durand et al., unpublished). Images of periprostatic nerve, connective tissue, prostatic glands, seminal vesicle, and vas deference were comparable to their corresponding histology. Motion artifacts could be sufficiently controlled to obtain high-resolution MPM images. At the end of the imaging session, the animals were closed and recovered from anesthesia. They were followed for 15 days after which they were re-laparotimized and reimaged. Neither the second look MPM imaging nor subsequent histopathology of the imaged tissue showed any identifiable damage (Durand et al., unpublished).
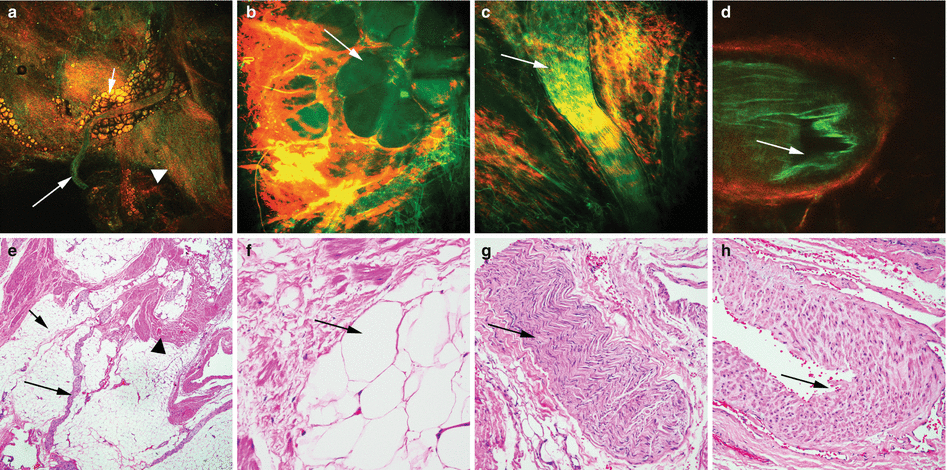
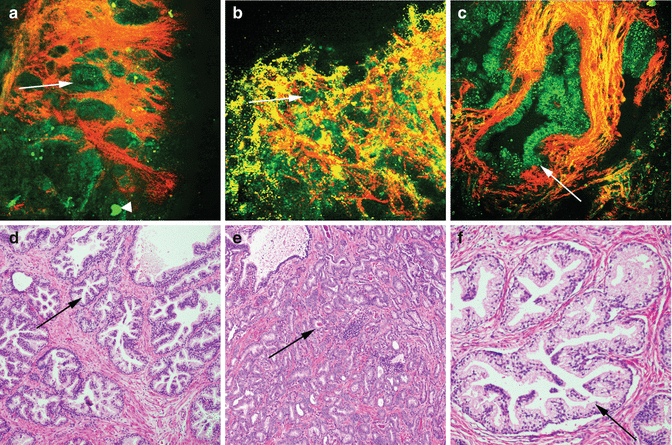
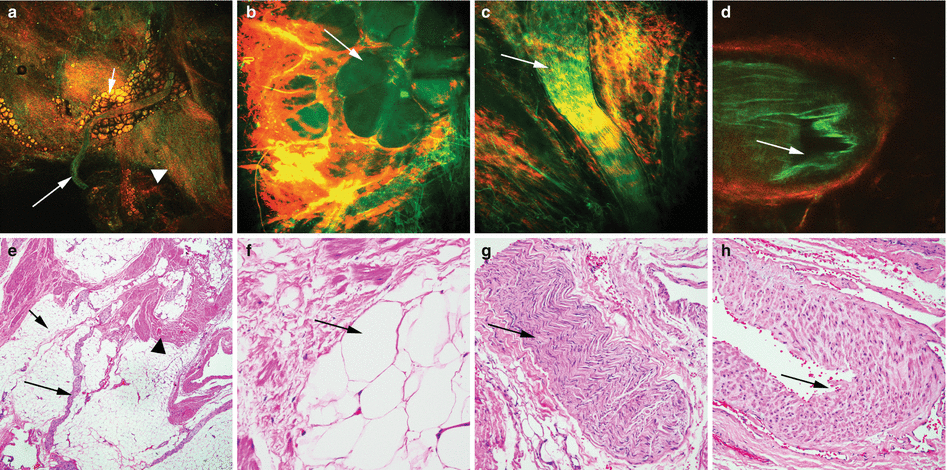
Fig. 6.4
MPM assessment of periprostatic tissue. MPM and corresponding H&E images of periprostatic tissue showing (a–e) a nerve (arrows) embedded in adipocytes (small arrows) and connective tissue (arrowheads) at low magnification. (b–f) Adipocytes (arrows). (c–g) A nerve bundle with fluorescence that derives from the axoplasm or the cytoplasm of the Schwann cells and (d–h) a medium-sized blood vessel with lumen (arrows) at high magnification. Original magnifications: MPM: a = 48×, b–d = 300×. H&E: e = 40×, f–h = 200×
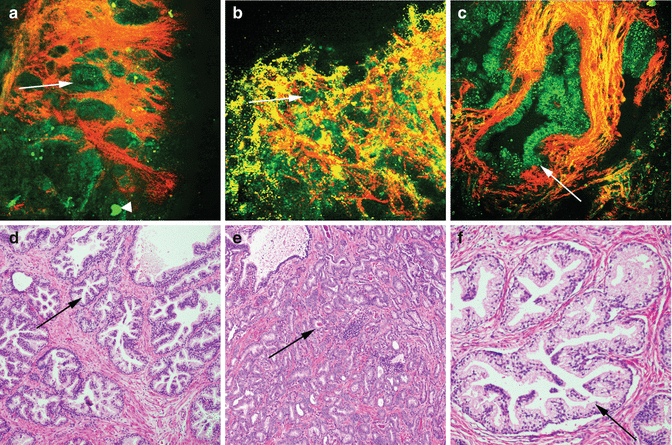
Fig. 6.5
MPM assessment of the prostate gland. MPM and corresponding H&E images of prostate gland showing (a–d) benign glands (arrows) with surrounding connective tissue (collagen; color-coded red). Concretion is shown with an arrowhead in one of the begin glands. (b–e) Adenocarcinoma of prostate with clusters of small tightly packed glands (arrows) and (c–f) benign glands at high magnification with infolded luminal epithelium. Original magnifications: MPM: a = 48×, b = 120×, c = 300×. H&E: d = 40×, e = 100×, f = 200×
Applications for the Management of Kidney Cancers
Over the past two decades or so, there has been an exponential increase in incidentally detected small and localized renal masses due to a substantial increase in noninvasive imaging for a variety of indications [42, 43]. Unfortunately, these noninvasive imaging techniques, such as CT or MRI, are not efficient at distinguishing benign from indolent from aggressively malignant renal carcinomas. Consequently, there is significant overtreatment of renal carcinoma, with imaging-detected masses often turning out to be benign or indolent after radical or partial nephrectomy [42]. Such overtreatment not only has deleterious effects on long-term renal function [44] but has also been shown to have negative effects on cardiovascular disease [45].
There is thus a real need to diagnose renal tumors more accurately before a surgical intervention. However, traditional excisional renal biopsies under ultrasound guidance are less than optimal at the current time. In a recent single-center retrospective study of pediatric renal patients, it was found that ~12 % of the biopsies did not shed light on the diagnosis and were unhelpful in patient management, another ~11 % were nondiagnostic, and an additional 1.5 % failed to yield enough tissue for examination [46]. Thus, MPM-assisted optical biopsy may serve to overcome some of the current bottlenecks in renal carcinoma management. Furthermore, during open or robotic nephrectomies, MPM could help accurately assess surgical margins in situ and thus prevent any unnecessary loss of renal tissue that may negatively impact patient prognosis.
Here, we show evidence that MPM can accurately identify all important histological components of normal kidney (Fig. 6.6), as well as correctly diagnose non-papillary (Fig. 6.7) and papillary (Fig. 6.8) kidney tumors of various histological subtypes.
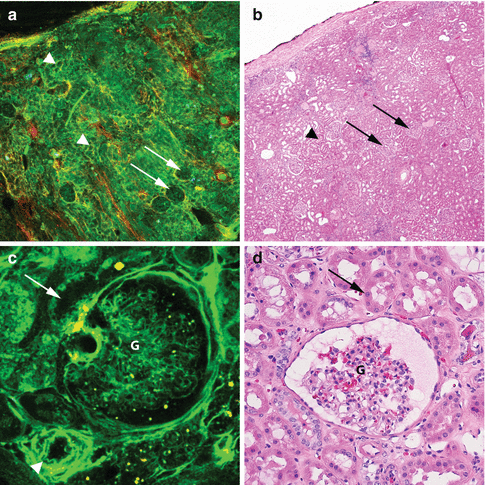
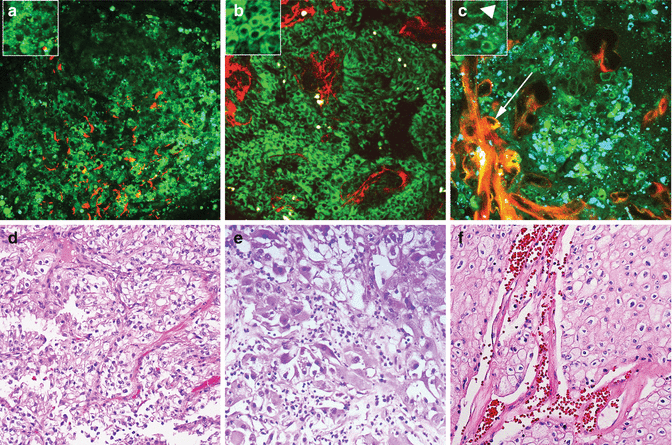
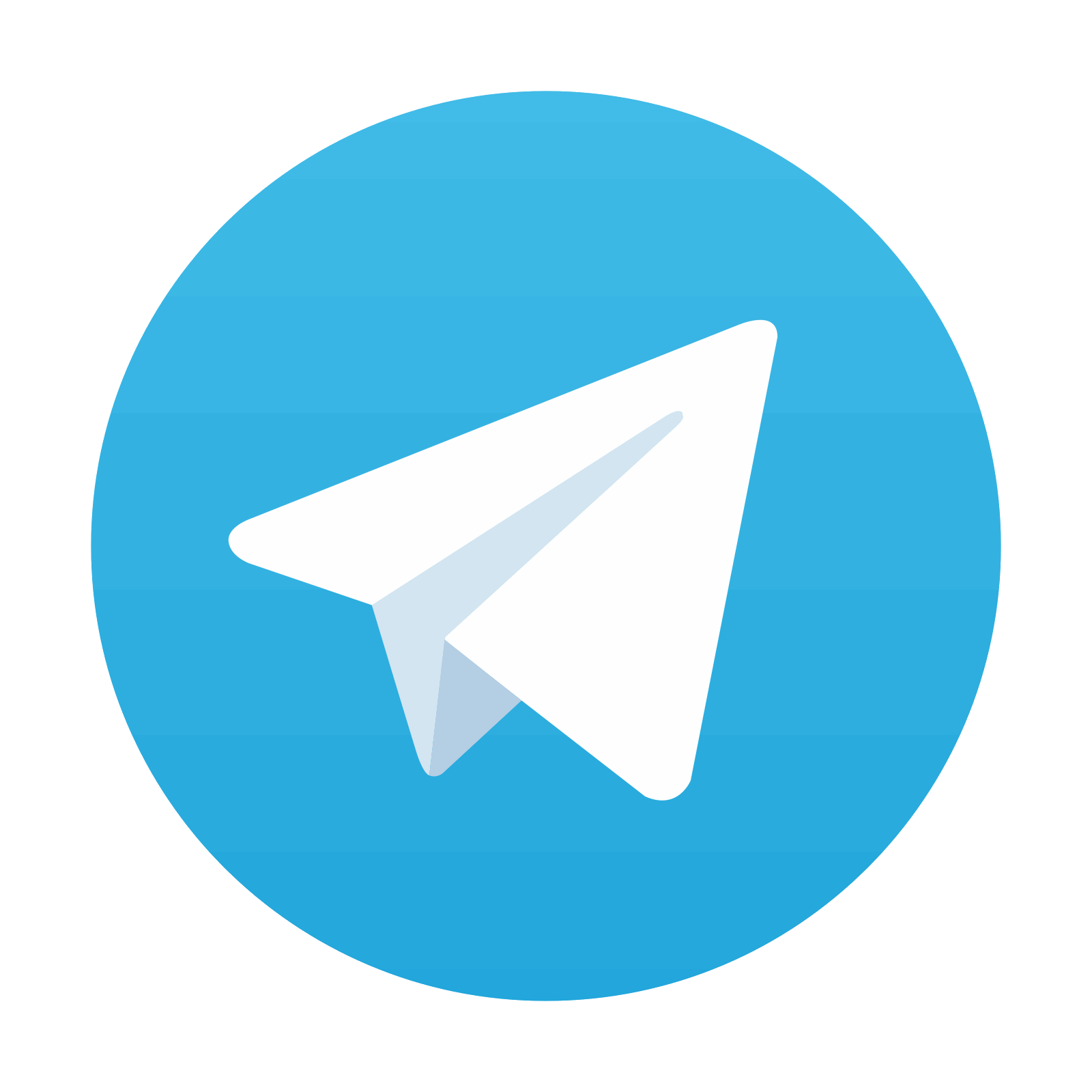
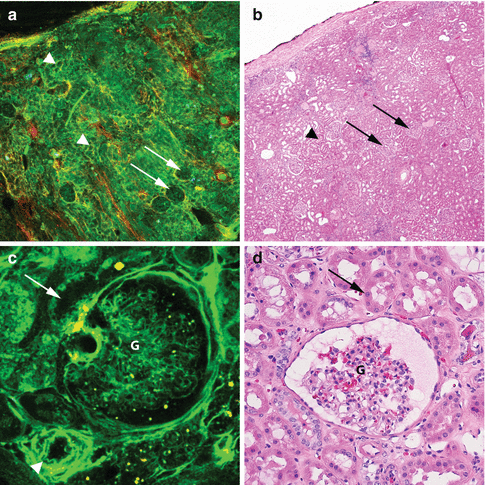
Fig. 6.6
MPM assessment of normal histology of kidney. MPM image (a) and corresponding H&E image (b) show a low-magnification image of normal kidney with glomeruli (arrows) and surrounding tubules (arrowheads). The MPM image (c) and the corresponding H&E image (d) show a glomerulus (g), tubule (arrow), and an arteriole (arrowhead) at high magnification. Original magnifications: MPM: a = 48×, c = 300×. H&E: b = 40×, d = 200×
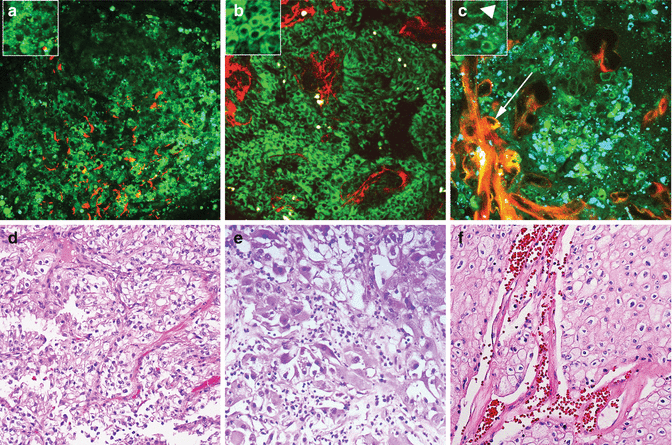
Fig. 6.7
MPM assessment of non-papillary kidney tumors. MPM image (a) and corresponding H&E image (d) of low-grade clear cell renal cell carcinoma, showing sheets of cells with intervening collagen fibers (red). Inset shows tumor cells with lipidic droplets in the cytoplasm and low N:C ratio. MPM image (b) and corresponding H&E image (e) of high-grade clear cell renal cell carcinoma showing tumor cells and stroma (red). Inset shows cells with homogeneous cytoplasm lacking lipid droplets and high N:C ratio. MPM image (c) and corresponding H&E image (f) of chromophobe renal cell carcinoma, showing sheets of tumor cells (green) and thickened blood vessels (arrow). Inset shows prominent intracytoplasmic granules (blue, hypothesized to correspond to cytoplasmic vesicles) and a cell with irregular enlarged nuclei (arrowhead). Original magnifications: MPM: a–c = 300×. H&E: d–f = 200×
< div class='tao-gold-member'>
Only gold members can continue reading. Log In or Register a > to continue
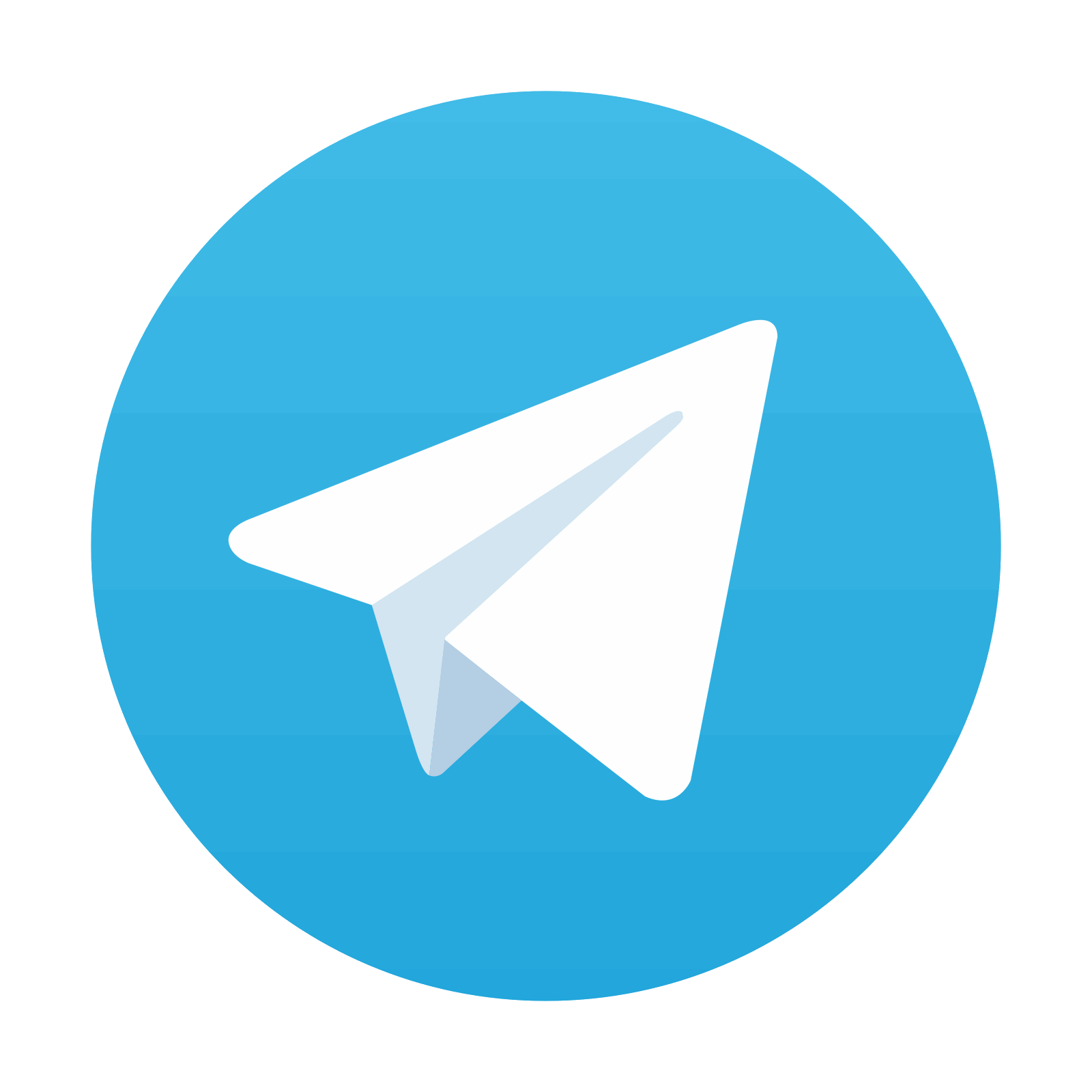
Stay updated, free articles. Join our Telegram channel

Full access? Get Clinical Tree
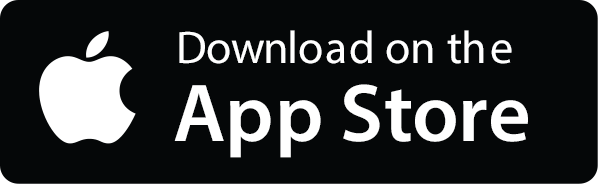
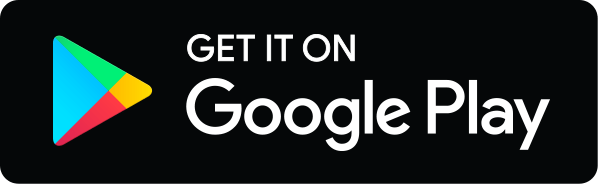
