Clinical phase
Compound
Type of compound
Target
Study details +/- preliminary findings
I
MGAH22
mAb
HER2
Dose escalation study of MGAH22 in patients with refractory HER2-positive breast cancer and patients with other HER2-positive carcinomas [including gastric] for whom no standard therapy is available is currently recruiting patients [124]
I/II
HM781-36B [Poziotinib]
Small molecule, pan-HER TKI
HER1, HER2, and HER4
A Phase I-II Study of HM781-36B[Poziotinib]Combined with paclitaxel and trastuzumab in HER-2-positive advanced gastric cancer is currently in the recruitment phase
II
MM-111
Bi-specific Ab
HER2 and HER3
A randomized, open label, Phase 2 Study of MM-111 and paclitaxel with trastuzumab in patients with HER2-positive gastric cancer who have failed first line therapy is currently recruiting participants (estimated enrollment: 120) [125]
ASLAN001
Small molecule, pan-HER TKI
HER1, HER2, and HER4
Dacomitinib
Irreversible pan-HER TKI
HER1 and HER2
7.4 % response rate and 7.1 month median survival in HER2-positive advance gastric cancer after failure of at least one prior chemotherapy [n = 27]. Concluded to be an active and safe treatment option [128]
Afatinib
Irreversible pan-HER TKI
HER1, HER2, and HER4
43 % of patients with metasttic HER2-postive trastuzumab-refractory esophagogastric cancer treated with afatinib derived clinical benefit. Study currently ongoing, being expanded to include additional patients [n = 7] [129]
II/III
Pertuzumab
mAb
HER2[subdomain1], HER2 hetero dimerization
A phase II trial evaluating 2 different doses of pertuzumab in combination with trastuzumab and chemotherapy in patients with HER2-positive advance gastric cancer is currently in progress [130]
An international double-blinded, placebo-controlled, randomized phase III study, JACOB, is currently in progress evaluating pertuxumab (versus placebo) with trastuzumab and chemotherapy in patients with HER2-positive metastatic gastric or GEJ cancer (estimated enrollment:780) [131]
Ado-trastuzumab
ADC
HER2 [subdomainIV]
Preclinical studies have found ado-trastuzumab emtansine to be more effective than trastuzumab in vitro as well as in vivo [132, 133]. A randomized, multicenter, adaptive Phase II/III study to evaluate the efficacy and safety of trastuzumab emtansine versus taxane (Docetaxel Or Paclitaxel) in patients with previously treated locally advanced or metastatic HER2-Positive gastric cancer is currently underway [134]
III
Lapatinib
Reversible pan-HER TKI
HER1[EGRF] and HER2
LoGIC, a randomized, placebo-controlled, phase III study evaluating lapatinib in combination with chemotherapy [capecitabine plus oxaliplatin] in HER2-positive advanced or metastatic gastric, esophageal or GEJ adenocarcinoma found the addition of lapatinib did not improve clinical outcomes, with a hazard ratio (HR) of 0.91. However, certain subgroups, such as Asian patients and patients under 60 years old, showed improvement, HR = 0.68 and HR = 0.69, respectively (n = 545) [135]. TYTAN, randomized, placebo-controlled, phase II study evaluating labatinib in combination with paclitaxel as second line therapy in HER2-positve gastric cancer is currently underway. The interim safety analysis reported no significant issues (n = 107) [136]
HER2
Human epidermal growth factor receptor 2 (HER2) is overexpressed in several types of human cancers, and has become a well-established player in the pathogenesis of up to 54 % of gastric carcinomas [72]. The HER2 gene is located on chromosome 17q21, and encodes a 185 kDa protein which belongs to the epidermal growth factor receptor (EGFR) family of transmembrane tyrosine kinase receptor proteins [73]. The EGFR family comprises four structurally-related members, HER1 (ErbB1 or EGFR), HER2 (ErbB2, c-erbB2 or Her2/neu), HER3 (ErbB3), and HER4 (ErbB4), which are all involved in activating downstream signaling pathways associated with cell proliferation, differentiation, migration, adhesion, survival, and angiogenesis [74].
HER2 is well recognized as a proto-oncogene, as its amplification results in overexpression of the HER2 RTK protein, which promotes cell proliferation and survival, properties promoting malignant transformation. In addition to being found on the cell membrane, HER2 receptors have also been localized to the cellular nucleus, functioning as transcription factors for proto-oncogenes such as cyclin D1 [75, 76].
HER2 was first identified as being overexpressed in gastric cancer in 1986; further investigations have reported its upregulation in as low as 4 % to as high as 53 % of gastric tumors [72, 77–79]. However, the exact timing of HER2 overexpression in the pathogenesis of gastric cancer is controversial. Several studies have demonstrated that HER2 upregulation is a late event during tumor progression, and hence describe it as a marker of advanced disease [80]. In contrast, other studies report HER2 overexpression in early stages as well as high concordance between HER2 expression in metastatic tissue and its associated primary tumor. This implicates HER2 overexpression as an early event with maintenance of HER2 expression being an important factor throughout the metastatic process [81, 82]. It has been well-established that HER2 overexpression varies across histological subtypes. HER2 overexpression is consistently found to be highest in the intestinal phenotype, followed by mixed, then diffuse phenotypes [78, 79, 81, 83–89]. In addition to histological subtype, anatomic localization of the primary tumor has been found to correlate with HER2 status. HER2 overexpression is more commonly found in tumors originating more proximally from the gastrooesophageal junction (GEJ) or stomach cardia, compared to those arising from the mid-and distal stomach [78, 79, 90]. In regards to the influence of patient demographics on HER2 status, a systematic review by Chua et al. found there to be no association between HER2 overexpression and age or gender [91]. Interestingly, a screening program part of a multicenter trial investigating treatment of HER2-positive gastric cancer in 24 countries found that HER2 status varies geographically: Australia was found to have the highest percentage (33.2 %) of HER2-positive gastric cancers and Taiwan the lowest (5.9 %) [84].
HER2 overexpression has been clearly identified as a poor prognostic marker affiliated with decreased survival in breast cancer; however, its role in gastric cancer is less clear [92–94]. The relationship between HER2 expression in gastric cancer and prognosis was first studied in 1991, at that time it was identified as an unfavorable prognostic marker [95]. Over the past 2 decades, dozens of studies attempting to replicate the original finding that HER2 overexpression correlates with a favorable prognosis have yielded varying results. In 2012, two independently conducted systematic reviews concluded HER2 overexpression is associated with poorer prognosis [83, 91]. The review conducted by Chua and Merrett, which included 49 studies, totaling 11,337 patients, found median survival to be shorter in HER2-positive gastric cancers (21 months compared to 33 months). However, when assessing Overall Survival (OS) as a primary outcome measure, the majority of studies reported no significant difference in 20 of 35 studies which assessed OS. Additionally, there was no correlation between HER2 status and clinicopathologic characteristics such as tumor depth of invasion, TNM stage, presence of microvascular invasion or presence of perineural invasion. In contrast, Jorgensen and Hersom found that 71 % of 42 studies, which included 12,749 patients, correlated HER2-positive status with poor survival and/or clinicopathological characteristics such as those mentioned above. More recent studies have identified HER2-positive status as a favorable prognostic factor in stage III and metastatic gastric cancers, however, on multivariate analysis HER2 was not identified as an independent prognostic factor [96, 97]. Additionally, a large surgical series found no correlation between HER2 status and OS in 829 stage II/III resected gastric cancer cases [98]. In summary, the role of HER2 in gastric cancer prognosis remains highly controversial and further investigation must be done before definitive conclusions can be drawn.
Despite controversies around prognostic properties of HER2, evidence supporting HER2 as an important therapeutic target in gastric cancer, especially when used in combination with conventional chemotherapy, is convincing. Treatment of HER2-positve breast cancer with trastuzumab , a fully humanized monoclonal antibody directed against the extracellular domain of HER2, has been proven to confer a survival advantage and its use is recognized as the standard of care [93, 99, 100]. Trastuzumab’s antiproliferative effect is proposed to act through blockade of signaling pathways, downregulation of the HER2 protein, activation of apoptotic signals, and induction of antibody-depended cell medicated cytotoxicity [101, 102]. The 2010 Trastuzumab for Gastric Cancer (ToGA) phase III, open-label, randomized control trial, identified trastuzumab as the first molecular target shown to improve survival in metastatic gastric cancer when combined with platinum-5-fluorouracil chemotherapy, revolutionizing the treatment of HER2-positive gastric cancers. The addition of trastuzumab to chemotherapy was shown to significantly improve OS compared to chemotherapy alone (13.8 months compared to 11.1 months). An exploratory, post-hoc analysis that patients with HER2-positive tumors (IHC score of 2 with positive FISH or IHC score 3 independent of FISH score) were found to have a median OS of 16 months, compared to 10 months in the HER2-positve tumors that were FISH+ with an associated IHC score of 0 or 1 [103]. The results of the ToGA trial confirmed the safety and efficacy of the addition of trastuzumab to standard chemotherapy, prompting food and drug administration (FDA) approval for its application in combination with cisplatin and a fluoropyrimidine for the treatment of HER2-positive metastatic gastric or GEJ cancers [104]. Following the success of ToGA trial, a number of other HER2 targeted compounds have gone into clinical development, including several other monoclonal antibodies, an antibody-drug conjugate, and more recently, small molecule inhibitors (see Table 3.1; [78, 104–106]) .
Molecular Signatures in Gastric Cancer
The clinical classification of GC as diffuse or intestinal does not currently dictate treatment [8, 19]. However, from the point of view of prognostication, these histologic classifications do predict different clinical courses [8, 19]. For example, different patterns of metastatic spread are observed in diffuse as opposed to intestinal type tumors [19]. Similarly, proximal gastric tumors carry a worse prognosis than more distal ones [19]. These findings imply differences at the genetic level [19]. Accordingly, contemporary studies demonstrate the existence of distinct molecular GC phenotypes, both between and within given histologic subtypes [13, 17, 19]. This permits classification along molecular lines, according to specific genetic changes. When considered within the context of known driver mutations, such classification may pave the way for more individualized, potentially effective treatments.
Molecular Signatures Within Histologic Subtypes
In the study by Shah et al., the authors sought to determine if genetic differences could be used to discriminate between histopathological subtypes of GC [19]. The authors categorized GC along three histologic subtypes: Proximal nondiffuse GC, diffuse GC, and distal nondiffuse GC. An analysis of gene up or downregulation between these groups revealed significant differences between them. Pathway analysis between GC subtypes and adjacent normal tissue revealed upregulation of metabolic pathways involved in lipid and carbohydrate metabolism in both proximal and distal non-diffuse type cancers. All subtypes demonstrated downregulation of known tumor suppressors including TP53. These results highlight the fact that genetic features underlie known histologic phenotypes and are responsible, at least in part, for their behavior. However, the authors did not identify clear differences between each GC subtype. This finding may reflect weaknesses inherent to histologic classification and is reflected in the more recent attempt to divide GC entirely along molecular lines [13, 17, 19].
Molecular Signatures Independent of Histologic Subtype
One problem with characterizing the molecular phenotype of GC along histologic lines is the assumption that all tumors belonging to a given group are the same at the genetic level. Current evidence suggests that this is not the case. For example, the study by Lei et al. demonstrates that genetically similar groups of GC can include both diffuse and intestinal type tumors [17]. By examining gene expression profiles, copy number alteration and DNA methylation patterns in 248 GC patients, the authors identified 3 GC subtypes: mesenchymal, proliferative, and metabolic [17].
The mesenchymal subtype demonstrated an overrepresentation of genes involved in focal adhesion, extracellular matrix receptor interactions, and cell adhesion compared to the other two subtypes. Mesenchymal tumors were predominantly of the diffuse type according to histologic classification (58.2 %). However, significant proportions are of intestinal or mixed types (29.9 and 11.9 % respectively) [17]. This tumor subtype was found to demonstrate cancer stem cell (CSC) like properties based on the observation of high CD44 expression and low CD24 expression [17]. This phenotype has been observed in CSC derived from prostate, breast, pancreatic, and gastric tumors [17]. Interestingly, CD44 overexpression has been associated with poor prognosis and reduced overall survival in GC [107]. CD44 is a cell surface receptor, which mediates interaction with ECM component, particularly HA [107].
CD24 is a GPI-anchored cell surface glycoprotein whose expression has been demonstrated in a variety of malignancies. It is thought to play a role in tumor growth, invasion and metastasis as well as in mediating sensitivity to certain chemotherapeutic agents [108]. In breast cancer, CD44 high/CD24 low tumors demonstrate stem cell-like properties, resistance to chemotherapeutic agents and may predispose patients to higher rates of relapse [108]. To date, such an association in GC remains unclear.
The proliferative subtype is enriched with regard to CNA compared to the mesenchymal and metabolic subtypes [17]. In addition to genomic amplifications, this group exhibited a higher frequency of TP53 mutations compared to the other two. Additional characteristics of this group include a high degree of genomic instability, CpG hypomethylation, and amplification of known oncogenes , particularly CCNE1, MYC, ERBB2, and KRAS as well as deletions of PDE4D and PTPRD. As a consequence, the authors postulate excessive signaling via activation of E2F, MYC, and RAS pathways [17].
CCNE1 encodes cyclin E1, which functions in conjunction with Cdk 2 to regulate the G1/S phase transition [108]. While its specific role in driving GC carcinogenesis remains incompletely understood, its overexpression has been described in the context of other malignancies such as breast, and ovarian carcinomas [108, 109]. Its contribution to tumor progression is thought to be mediated by its ability to drive cell cycle progression [108, 109].
The MYC gene encodes the myc proto-oncogene, which functions as a regulator of proliferation, differentiation, and apoptosis [110]. Deregulated myc function alone can induce cellular transformation both in vitro and in vivo [110]. Aberrant myc function has been extensively demonstrated in gastric cancer ranging in frequency form 23.5–100 % of samples studied [110].
As previously stated, ERBB2 encodes the HER2 cell surface RTK whose activation leads to downstream signaling via both the PI3—Akt and p38-MAPK pathways [17, 22]. Aberrant KRAS signaling via the MAPK pathway has similarly been demonstrated in a significant proportion of GC patients, with KRAS representing an attractive potential therapeutic target in GC [25].
The gene products of PDE4D and PTPRD encode proteins involved in cAMP degradation and inhibition of RTK signal transduction [17, 111–113]. As such, they function as tumor suppressors with their inactivation contributing to unregulated and excessive signal transduction. In vitro inhibition of PDE4D has been shown to result in apoptosis and growth inhibition in a variety of human cancer cell lines including GC [111]. Similarly, PTPRD mutations have been demonstrated in a small proportion of gastric cancers and may be associated with tumor progression [112, 113].
Further examination if the proliferative subtype of GC demonstrates a preponderance of intestinal type tumors (73 %) compared to diffuse and mixed (17.3 and 9.1 % respectively) [17].
The final GC subtype was termed the metabolic subtype [17]. These tumors were found to bear genetic similarity to a premalignant gastric lesion known as spasmolytic polypeptide expressing metaplasia (SPEM) [17]. Like Intestinal metaplasia (IM), this lesion arises in the context of environmental insults such as H. pylori infection, and dietary nitrosamines. SPEM tends to localize to the body of the stomach and is associated with the loss of mature parietal and chief cells and a relative abundance of mucous producing neck and antral gland cells which express TFF2 (spasmolytic polypeptide), a secreted polypeptide normally expressed in the antrum and intestinal mucosa [114]. Roughly, equal proportions of diffuse and intestinal types characterized tumors of this type histologically (40.6 % diffuse, 53.6 % intestinal) [17]. Taken together, these results demonstrate an important heterogeneity in the molecular phenotype of gastric cancer that exhibits discordance with histologic classification [17].
Molecular Signatures in Gastric Cancer: Implications for Treatment
Interestingly, while no difference in survival was noted among the three subtypes identified by Lei et al., the molecular signatures predicted response to therapy [17]. Patients with tumors of the metabolic subtype were the most likely to demonstrate a survival benefit while receiving 5 FU-based chemotherapeutic regimens. This was attributed to the significantly lower levels of thymidylate synthase (TS) and dihydropyrimidine reductase (DHPR) , which represent the target of 5FU and the enzyme responsible for its degradation respectively, in metabolic type tumors [17]. Along these lines, mesenchymal subtype cancers were postulated to demonstrate increased sensitivity to specific inhibitors of the PI3K-Akt-mTOR pathway . This was based on the observed activation of this pathway in mesenchymal subtype tumors versus proliferative and metabolic subtypes. Accordingly, GC cell lines of the mesenchymal subtype demonstrate increased sensitivity to PI3-Akt-mTOR inhibitors [17].
The importance of genomic classification with respect to predicting response to treatment has been suggested by additional studies [13]. Recently, a study put forward by the Cancer Genome Atlas Group proposed the classification of GC into 4 groups entirely along molecular lines [13]. The authors identified four groups: EBV positive tumors, microsatellite unstable tumors, genomically stable tumors, and tumors with chromosomal instability. EBV positive tumors demonstrated a high degree of hypermethylation and frequent mutations in PIK3CA, suggesting a high degree of involvement of the PI3K-Akt pathway in such tumors. MSI tumors demonstrated silencing of genes associated with mismatch repair such as MLH1. In addition, frequently mutated genes in these tumors included current targeted therapy targets such as ERBB2. The genetically stable group demonstrated a high frequency of mutation within RHOA and CDH1, both the known driver mutations in GC, and the preponderance for diffuse type histology [13, 16]. Finally, tumors characterized by Chromosomal instability (CIN) demonstrated marked aneuploidy and frequent mutations in RTK. As in the previous study, no clear differences in the patient’s survival could be identified between the 4 different groups [13]. However, a clear understanding of the characteristic genomic alteration associated with a given tumor subtype could suggest, which targeted therapies to apply and to whom [13]?
This approach to phenotypic characterization permits classification of gastric cancer types according to global changes in the gene expression as opposed to simply describing genetic abnormalities compared to nonneoplastic tissue. The implications of molecular characterization in this manner are that, more effective therapies can be administered based on a personalized approach to the diagnosis and management of GC [13, 17, 19].
Epigenetic Alterations in Gastric Cancer
In addition to mutations of the coding regions of genes, cellular expression profiles can be influenced by DNA modifications occurring within the noncoding regions [15, 16, 18, 25, 115]. Furthermore, such processes do not necessarily, directly alter the DNA sequences within a cell’s genome. Rather, a number of structural chromatin modifications and alterations to mRNA transcripts can profoundly influence gene expression at the transcriptional and the translational levels [15, 16, 18, 25, 115]. Such alterations are said to be epigenetic and play an essential role in the normal tissue development [15, 115]. In addition, epigenetic processes have been shown to play an important role in the gastric tumorigenesis [15, 16, 18, 25, 115]. Among the epigenetic mechanisms describe thus far involved in the pathogenesis of GC are DNA methylation and the more recently described microRNA’s [15, 16, 18, 25, 115].
Methylation
DNA methylation occurs at sites rich in the GC nucleotides termed as CpG islands (CGI) [15, 18]. These tend to cluster within the 5’UTR regions upstream of specific gene promoters [15]. Methylation within regions close to gene promoters, mediated by DNA methyltransferases, has a tendency to silence gene expression. Conversely, demethylation has a tendency to exert the opposite effect. In addition, methylation may occur outside the promoter region, sometimes within the coding sequences themselves. The effects associated with this type of methylation are more variable [15].
DNA methylation is the most extensively studied epigenetic change to take place in the context of GC to date [15]. Along these lines, a number of studies have highlighted genes whose transcription is modified by either hyper or hypo methylation. These include a large number of genes involved in diverse fundamental cellular pathways including DNA repair, cell cycle progression, cellular adherence, invasion and migration, growth and differentiation, apoptosis, and transcriptional regulation [15].
As previously stated, hypermethylation within the promoter region has a tendency to reduce gene expression. Along these lines, a number of tumor suppressor genes are silenced in GC patients including MLH1, APC, and CDH1, involved in mismatch repair, Wnt signal transduction and cellular adhesion respectively [15–17, 25]. In addition to hypermethylation, a number of genes have been identified whose expression is upregulated by hypomethylation including genes such as CDX 1, which is involved in the development of intestinal metaplasia, MET, which encodes the HGF receptor, CLDN15, which belongs to the claudin family involved in tight junction formation, and TFF3, a transcription factor whose upregulation has previously been described in gastric cancer and which is expressed within normal columnar epithelium [15–17, 25, 116, 117]. These are but a few genes within a slew of others listed solely to highlight the diversity of cellular processes affected by the methylation status [15].
Given, the number and breadth of functions associated with proteins affected by DNA methylation in the GC, drawing any meaningful conclusions by simply listing them becomes prohibitive. In light of this fact, some studies have attempted to characterize methylation patterns in the GC patients within groups, or methylation signatures, in an attempt to draw conclusions regarding their biologic, and more importantly, their clinical significance [18]. Zouridis et al. characterized the DNA methylation profile in 203 primary GC samples and compared them to 94 matched control samples [18]. In so doing they identified distinct patterns of methylation associated with transcriptional repression or activation. In this manner, the authors demonstrated CGI methylator phenotypes (CIMP) in certain GC samples and identified DNA methylation inhibitors as a possible therapeutic avenue in CIMP tumors [18].
Methylation analysis of primary GC tumors demonstrated that the vast majority clustered into distinct groups, differentiating malignant tissue from benign gastric mucosa [18]. In addition, the majority of tumors [83 %] demonstrated hypermethylation. The remainder demonstrated marked and significant hypomethylation. In keeping with the observation that hypermethylation silences gene transcription, the authors identified that hypermethylation in CpG island (CGI) near to gene promoters resulted in gene silencing. They similarly demonstrated that the opposite also holds true, with hypomethylation near the promoter region correlating with gene upregulation. However, when hypermethylation within the coding regions of genes was identified, it was associated within genetic upregulation, with the converse being associated with genetic silencing. In addition, hypermethylation near promoter regions tended to cluster with hypomethylation within the coding regions and vice versa. Taken together, this date demonstrates a novel “tandem control” mechanism, whereby, methylation within the promoter region may interact with CpG methylation within genes themselves in order to exert global effects on transcriptional control [18]. Furthermore, tumors demonstrating long regions of hypomethylation were found to be significantly more prone to chromosomal breakage and accordingly demonstrated increased chromosomal instability compared to hypermethylated tumors [18]. Collectively, this data suggests that methylation patterns can exert profound effects on genetic control over great genetic distances [18].
The ability to categorize tumors according to methylation pattern has important implications [15, 18]. This is because methylation patterns are associated with neoplastic progression and patient outcome [15, 18]. For example, CIMP tumors tended to occur in younger patients, harboring poorly differentiated tumors. Such patients also demonstrated poorer outcomes independent of tumor stage compared to non-CIMP patients. Although CIMP tumors demonstrate downregulation of known tumor suppressors such as MLH1 and CDH1, their gene expression pattern did not permit their differentiation from non-CIMP tumors as readily as did their methylation pattern [18]. These finding led the authors to postulate that CIMP gastric cancers represent a distinct tumor subset, not related to gene expression per se, but rather methylation pattern with profound implications with respect to clinical course [18].
In keeping with their previous observations, the authors sought to determine if ongoing methylation plays an important role in the tumorigenesis of CIMP neoplasms [18]. Treatment of CIMP cell lines with a DNA methyltransferase inhibitor resulted in diminished proliferation in vitro. Conversely, treatment of non-CIMP cell lines with a DNA methyltransferase inhibitor failed to reduce tumor cell growth. Furthermore, in vivo, significant reductions in tumor development were observed in CIMP positive tumors following concurrent treatment with a DNA methyltransferase inhibitor and cisplatin [18]. Thus, collectively this data indicates that GC may exhibit distinct methylation patterns that can predict a patient’s clinical course and potential susceptibility to therapy [18]. Conceptually, these results imply that targeting specific mutations within coding regions may not encompass the entirety of where therapeutic modalities should be focused.
Micro RNA
Micro RNA’s are a recently described class of RNA which play an extensive role in the posttranscriptional control of mRNA [14]. They are approximately 18–25 nucleotides in length and exert their effects via complementary binding with an mRNA molecule [14]. Such interactions can result in inhibition of translation or accelerated mRNA degradation [14]. Furthermore, a given mRNA can fall under the regulation of a single micro RNA or multiple different micro RNA. Similarly, a given micro RNA can interact with multiple mRNA transcripts [14, 115, 118]. These molecules play a significant role in posttranscriptional control and are thought to regulate up to 60 % of human genes at this level [14]. The preponderance of micro RNA is encoded within introns. However, they can localize within exons as well [14]. Transcriptional control of micro RNA is diverse, such that those located within introns fall under the transcriptional regulation of shared promoters [14]. Conversely, those located within exons are regulated by their own unique promoter [14]. As with all other genes, micro RNA transcription is subject to modification/disruption at the genetic level, as a result of genetic mutation, deletion, or amplification for example [14, 115, 118–120]. In addition, epigenetic processes such as DNA methylation can play a role [14, 115, 118–120]. However, globally, very little is known to date regarding the regulation of micro RNA.
As previously stated, micro RNA exert widespread regulatory effects within the cell, influencing basic cellular processes such as cell cycle progression, growth, and differentiation [14, 115, 118–120]. Accordingly, their dysregulation has been shown to play an important role in tumorigenesis [115]. With respect to GC, micro RNA molecules can function as either tumor suppressors or in an oncogenic capacity [115]. Along these lines both upregulation and downregulation of specific micro RNA molecules has been shown to exert pro-tumorigenic effects related to a number of fundamental processes including cell cycle progression, apoptosis, invasion, and metastasis [115, 118–120].
< div class='tao-gold-member'>
Only gold members can continue reading. Log In or Register a > to continue
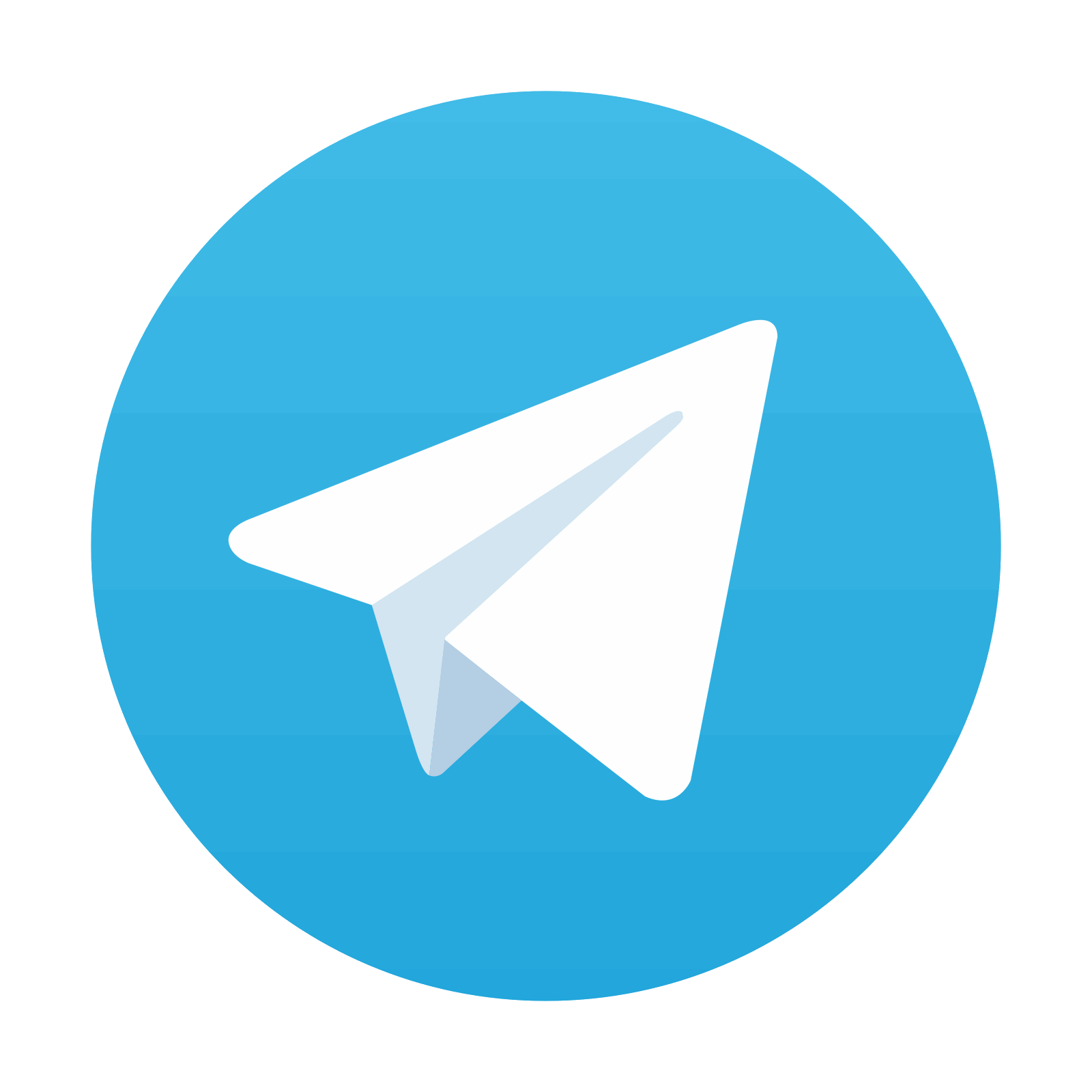
Stay updated, free articles. Join our Telegram channel

Full access? Get Clinical Tree
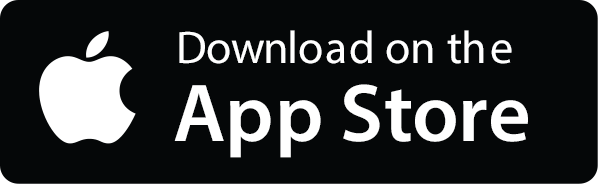
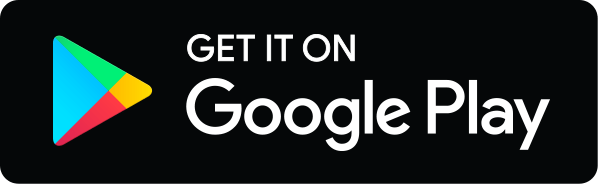