Chapter 14 Alan J. Wein, MD, FACS, PhD (hon); Ariana L. Smith, MD The lower urinary tract (LUT) functions as a group of interrelated structures with a joint function in the adult to bring about efficient and low-pressure bladder filling, low-pressure urine storage with perfect continence, and periodic complete voluntary urinary expulsion, again at low pressure. Because in the adult the LUT is normally under voluntary neural control, it is clearly different from other visceral organs, which are regulated primarily by involuntary mechanisms. For description and teaching, the micturition cycle is best divided into two relatively discrete phases: bladder filling/urine storage and bladder emptying/voiding. The micturition cycle normally displays these two modes of operation in a simple on-off fashion. The cycle involves switching from activation of storage reflexes and inhibition of the voiding reflex to inhibition of the storage reflexes and activation of the voiding reflex and back again. In this chapter, we first summarize some relevant facts regarding the anatomy, neuroanatomy, physiology, and pharmacology of the LUT. We then answer certain important functional questions related to the filling and storage phase and the emptying and voiding phase of micturition. Certain “rules” are formulated that must be satisfied for the LUT to function normally. By extrapolation, these rules are used as a basis for a very simple functional classification of voiding dysfunction and as a framework to understand urodynamic evaluation and the rationale for all types of treatment. The neurourologic evaluation, classification schemes for voiding dysfunction, and the more common types of neurogenic and nonneurogenic voiding dysfunction are considered, followed by a synopsis and summation of pertinent points relative to all types of treatment for filling/storage and for emptying/voiding disorders. Benign prostatic hyperplasia (BPH) and related issues are discussed in Chapter 15. 2. The classic view of the external urethral sphincter, or external sphincter, is that of a striated muscle within the leaves of a “urogenital diaphragm” that extends horizontally across the pelvis. It is responsible for stopping the urinary stream when the command “stop voiding” is obeyed. The striated sphincter concept expands this definition to include intramural and extramural portions. The extramural portion is under voluntary control and corresponds roughly to the “classic” external urethral sphincter, although no unbroken sheet of muscle extends across the pelvis in either male or female, and thus no true urogenital diaphragm exists. The intramural portion denotes skeletal muscle that is intimately associated with part of the urethra in both sexes above the maximal condensation of extramural striated muscle, which is continuous from that level for a variable distance to the bladder neck in the female and at least to the apex of the prostate in the male, forming an integral part of the outer muscular layer of the urethra. Some call this intramural portion the intrinsic rhabdosphincter. Although differences of opinion exist regarding the ultrastructure and physiologic type of striated muscle fibers at various points within the striated sphincter mechanism, there is agreement on the general concept of a gradual increase in activity in the striated sphincter during bladder filling, maintenance with the potential of increases in this activity during bladder storage, and virtual disappearance of this activity just prior to normal emptying/voiding. 2. Sympathetic and parasympathetic: The terms sympathetic and parasympathetic refer simply to anatomic divisions of the ANS. The sympathetic division consists of those fibers that originate in the thoracic and lumbar regions of the spinal cord, whereas the parasympathetic division refers to those fibers that originate in the cranial and sacral spinal nerves. 3. Innervation and neuronal interaction: The classic view of the peripheral ANS involves a two-neuron system — preganglionic neurons emanating from the CNS and making synaptic contact with cells within ganglia, from which postganglionic neurons emerge to innervate peripheral organs (Figure 14-1). This relatively simple concept is still useful for the purposes of discussion but has undergone much expansion and modification. Most autonomic innervation of the LUT actually emanates from peripheral ganglia that are at a short distance from, adjacent to, or within the organs they innervate (the urogenital short neuron system). Additionally, the efferent autonomic pathways frequently do not conform to the classic two-neuron model, as they are often interrupted by more than one synaptic relay. For many years, the only autonomic neurotransmitters recognized were acetylcholine and norepinephrine. It has become obvious that other transmitters are involved in various components of the ANS, and a once relatively simple concept of chemical neurotransmission has been expanded to include synaptic systems that involve modulator transmitter mechanisms, prejunctional inhibition or enhancement of transmitter release, postjunctional modulation of transmitter action, cotransmitter release, and secondary involvement of locally synthesized hormones and other substances. All of these are subject to neuronal and hormonal regulation, desensitization, and hypersensitization. Finally, these relationships may be altered by changes that occur secondary to disease or destruction in the neural axis, obstruction of the LUT, aging, and hormonal status. 4. Bladder smooth muscle contraction and relaxation: The classic model of smooth muscle contraction involves synaptic release of neurotransmitters in response to neural stimulation, with the transmitter agents subsequently combining with a recognition site, or receptor, on the postsynaptic smooth muscle cell membrane. The transmitter-receptor combination then initiates changes in the postsynaptic effector cell that ultimately results in what we recognize as the characteristic effect of that particular neurotransmitter on that particular smooth muscle. 5. Neurotransmitter terminology — cholinergic and adrenergic subtypes: Clinicians are often confused because they assume that the terms sympathetic and parasympathetic imply particular neurotransmitters. These terms imply only anatomic origin within the ANS. Other adjectives are used to describe the nature of the neurotransmitter involved (see Figure 14-1). 6. Other peripheral neurotransmitters: Other nonadrenergic noncholinergic (NANC) peripheral neurotransmitters exist in the LUT and their roles in normal and abnormal states is the object of much current investigation. These are summarized in Table 14-1. Note, however, that the presence of a potential neurotransmitter and a laboratory tissue response to an agonist or/and antagonist does not necessarily imply physiologic function. Table 14-1 Possible Peripheral Transmitters and Modulators in the Lower Urinary Tract (Adapted from Andersson KE, Wein AJ: Pharmacology of the lower urinary tract: basis for current and future treatments of urinary incontinence. Pharmacol Rev 56:581–631, 2004; Michel MC, Andersson KE, editors: Handbook of experimental pharmacology: urinary tract, Berlin, 2011, Springer.) 7. Peripheral innervation: The pelvic and hypogastric nerves supply the bladder and urethra with efferent parasympathetic and sympathetic innervation, and both convey afferent sensory impulses from these organs to the spinal cord (Figure 14-2). The parasympathetic efferent supply is classically described as originating in the gray matter of the interomediolateral cell column of sacral spinal cord segments S2-S4. This preganglionic supply is ultimately conveyed by the pelvic nerve. These fibers synapse with cholinergic postganglions in the pelvic plexus or in ganglia within the bladder wall. Efferent sympathetic fibers to the bladder and urethra are thought to originate in the interomediolateral cell column and nucleus intercalatus of spinal cord segments T11-L2 and are carried within the hypogastric nerves. Bilaterally, at a variable distance from the bladder and urethra, the hypogastric and pelvic nerves meet and branch to form the pelvic plexus. Divergent branches of this pelvic plexus innervate the pelvic organs. Efferent innervation of the striated sphincter is classically thought to be somatic and to emanate from the Onuf nucleus in sacral spinal cord segments S2-S4, exiting the spinal cord as the pudendal nerve. Some clinicians believe that the striated sphincter is innervated by branches of the ANS as well. 8. Cholinergic innervation and parasympathetic stimulation: Cholinergic innervation is abundant to all areas of the bladder of animals and humans. Although most researchers agree on the existence of a cholinergic innervation at least of the proximal urethra in animals, there is disagreement regarding the extent (and in some cases existence) of a similar innervation in humans. It is generally agreed that abundant muscarinic cholinergic receptor sites exist throughout the bladder body and base musculature of various animal species and of humans and that they are more numerous in the bladder body. A sustained bladder contraction is produced by stimulation of the pelvic nerves, and it is generally agreed that reflex activation of this pelvic nerve excitatory tract is primarily or exclusively responsible for the emptying bladder contraction of normal micturition and for the involuntary bladder contractions seen with various neurologic and nonneurologic diseases/conditions. Whether acetylcholine is the sole neurotransmitter released in a normal human during such stimulation is highly controversial. Atropine resistance refers to the incomplete antagonism produced by atropine of the bladder response to pelvic nerve stimulation or of isolated bladder strips to electrical field stimulation (producing intramural neural stimulation). This is in contrast to atropine’s ability to completely block the response of bladder smooth muscle strips to exogenous acetylcholine. It is generally agreed that atropine resistance occurs in various experimental animal models, and the most logical explanation seems to be release of additional neurotransmitter(s) besides acetylcholine in response to nerve stimulation. Adenosine triphosphate (ATP) is most commonly mentioned as the prime candidate. Normally, atropine resistance does not seem to occur in humans. However, one should not ignore the possibility that different types of atropine resistance may exist in various types of bladder overactivity, regardless of the normal state of affairs. 9. Adrenergic innervation and sympathetic stimulation: Adrenergic innervation of the bladder and urethral smooth musculature has been extensively demonstrated in animal studies. These studies have shown that the smooth musculature of the bladder base and proximal urethra possesses a rich adrenergic (norepinephrine containing) innervation, whereas the bladder body has a sparse but definite adrenergic innervation. The density of innervation seems, in all areas, to be less than that of the cholinergic systems. Considerable disagreement exists, however, as to even the presence of postganglionic sympathetic innervation in the human bladder and proximal urethra. There is general agreement that the smooth muscle of the human male bladder neck possesses a dense adrenergic innervation, but there is little consensus otherwise. Even those researchers who ascribe a significant influence on the micturition cycle to the sympathetic nervous system (SYMPNS) have difficulty demonstrating more than a sparse adrenergic innervation in other areas of the bladder and urethra. There is general agreement, however, that the smooth muscle of the bladder and proximal urethra in a variety of animals and in humans contains both α- and ß-adrenergic receptors. Alpha-adrenergic contractile responses predominate in the bladder base and proximal urethra, whereas ß-adrenergic relaxation responses predominate in the bladder body. These are mediated primarily by the α1A and β-3 receptor subtypes, respectively. b. Increasing outlet resistance by stimulation of the predominantly α1A adrenergic receptors in the bladder base and proximal urethra c. Increasing accommodation by stimulation of the predominantly β-3 adrenergic receptors in the bladder body Micturition is basically a function of the peripheral ANS. However, the ultimate control of LUT function obviously resides at higher neurologic levels. There is general consensus that a micturition “center” in the spinal cord is localized to segments S2-S4, with the major portion at S3. Early workers believed that micturition was a simple sacral spinal reflex activity that was modulated by a number of central and peripheral reflexes. It is now acknowledged that the micturition cycle is coordinated in the pontine mesencephalic reticular formation. Input to this area is derived from the cerebellum, basal ganglia, thalamus, hypothalamus, and cerebral cortex. Bladder contraction elicited by stimulation at or above and through this area seems to occur with a decrease in activity of the periurethral striated musculature, as in normal micturition. In general, the tonic activity of the cerebral cortex and midbrain is inhibitory. The regions of the cerebral hemispheres primarily concerned with bladder function are the superomedial portion of the frontal lobes and the genu of the corpus callosum. There is debate as to whether the cerebral areas controlling bladder and striated sphincter activity are geographically separate. Evidence exists that endogenous opioid peptides influence micturition by a tonic inhibitory effect on detrusor reflex pathways. These inhibitory effects could be mediated at several levels, including the peripheral bladder ganglia, sacral spinal cord, and brainstem micturition center. Different types of opioid receptors may be responsible at different sites for different types of effects on bladder contractility. Numerous other potential neurotransmitters can be found in various areas of the CNS. A partial list is in Table 14-2. Table 14-2 Potential CNS Neurotransmitters Other than Opioids and Their Effects on the Micturition Reflex CNS, Central nervous system; SC, spinal cord. The normal adult bladder response to filling at a physiologic rate is an almost imperceptible change in detrusor pressure. During at least the initial stages of bladder filling, this very high compliance (Δ volume/Δ pressure) is due primarily to passive properties of the bladder wall. The elastic and viscoelastic properties of the bladder wall allow it to stretch to a certain degree without any increase in tension exerted on its contents, and at physiologic filling rates, intravesical pressure remains virtually unchanged. In the usual clinical setting, filling cystometry shows a slight increase in detrusor pressure, but this pressure rise is a function of the fact that cystometry filling is carried out at a greater than normal physiologic rate. Compliance can be decreased clinically by (1) any process that alters the viscoelasticity or elasticity of the bladder wall components, (2) certain types of neurologic injury or disease, and (3) filling the bladder beyond its limit of distensibility. The viscoelastic properties of the stroma (bladder wall less smooth muscle and epithelium) and the noncontraction of detrusor muscle account for the passive mechanical properties seen during filling. The main components of stroma are collagen and elastin. When the collagen component increases, compliance generally decreases. This can occur with various types of injury, bladder outlet obstruction (BOO), and neurologic decentralization. Once decreased compliance occurs because of a replacement by collagen or other components of the stroma, it is generally unresponsive to pharmacologic manipulation, hydraulic distention, or nerve section. Most often under those circumstances, augmentation cystoplasty is required to achieve satisfactory reservoir function. There may also be an active but nonneurogenic component to the filling/storage properties of the bladder. Some have suggested that an as yet unidentified relaxing factor is released from the urothelium, and others have suggested that urothelium-released nitric oxide (NO) may exert an inhibitory effect on afferent mechanisms during filling. At a certain level of bladder filling, spinal sympathetic reflexes are clearly evoked in all animals, and there is some indirect evidence to support such a role in humans. An inhibitory effect on bladder contractility is thought to be mediated primarily by sympathetic modulation of cholinergic ganglionic transmission. Through this sympathetic reflex, two other possibilities exist for promoting filling and storage. One is neurally mediated stimulation of the predominantly α1A adrenergic receptors in the area of the smooth sphincter, the net result of which would be to cause an increase in resistance in that area. The other is neurally mediated stimulation of the predominantly ß-3 adrenergic receptors in the bladder body smooth musculature, causing a decrease in tension. Good evidence also seems to support a strong tonic inhibitory effect of endogenous opioids on bladder activity at the level of the spinal cord, the parasympathetic ganglia, and perhaps the brainstem as well. Finally, bladder filling and wall distention may release autocrinelike factors, which themselves influence contractility, either by stimulation or inhibition. There is a gradual increase in urethral pressure during bladder filling, contributed to by at least the striated sphincter element and perhaps by the smooth sphincter element. The rise in urethral pressure seen during the filling and storage phase of micturition can be correlated with an increase in efferent pudendal nerve impulse frequency. This constitutes the efferent limb of a spinal somatic reflex that is initiated when a certain critical intravesical pressure is reached. This is the so-called guarding reflex, which results in an increase in striated sphincter activity (see Figure 14-8). Although it seems logical and certainly compatible with neuropharmacologic, neurophysiologic, and neuromorphologic data to assume that the muscular component of the smooth sphincter also contributes to the change in urethral response during bladder filling, it is extremely difficult to prove this either experimentally or clinically. The passive properties of the urethral wall undoubtedly play a large role in the maintenance of continence. Urethral wall tension develops within the outer layers of the urethra; however, it is a product not only of the active characteristics of smooth and striated muscle but also of the passive characteristics of the elastic collagenous tissue that makes up the urethral wall. In addition, this tension must be exerted on a soft, plastic inner layer capable of being compressed to a closed configuration — the “filler material” representing the submucosal portion of the urethra. The softer and more plastic this area is, the less the pressure required by the tension-producing layers to produce continence. Finally, whatever the compressive forces, the lumen of the urethra must be capable of being obliterated by a watertight seal. This mucosal seal mechanism explains why a very thin-walled rubber tube requires less pressure to close an open end when the inner layer is coated with a fine layer of grease than when it is not — the latter case being analogous to scarred or atrophic urethral mucosa. Normally, it is increased detrusor pressure producing the sensation of distention that is primarily responsible for the initiation of voluntary induced emptying of the LUT. Although the origin of the parasympathetic neural outflow to the bladder, the pelvic nerve, is in the sacral spinal cord, the actual organizational center for the micturition reflex in an intact neural axis is in the brainstem (pontine-mesencephalic formation), and the complete neural circuit for normal micturition includes the ascending and descending spinal cord pathways to and from this area and the facilitory and inhibitory influences from other parts of the brain. The final step in voluntarily induced micturition initially involves inhibition of the somatic neural efferent activity to the striated sphincter and an inhibition of all aspects of any spinal sympathetic reflex evoked during filling. Efferent parasympathetic pelvic nerve activity is ultimately what is responsible for a highly coordinated and sustained contraction of the bulk of the bladder smooth musculature. A decrease in outlet resistance occurs with adaptive shaping or funneling of the relaxed bladder outlet. In addition to the inhibition of any continence-promoting reflexes that have occurred during bladder filling, the change in outlet resistance may also involve an active relaxation of the smooth sphincter through a mechanism mediated by NO. The adaptive changes that occur in the outlet are also in part due to the anatomic interrelationships of the smooth muscle of the bladder base and proximal urethra (continuity). Other reflexes elicited by bladder contraction and by the passage of urine through the urethra may reinforce and facilitate complete bladder emptying. Superimposed on these autonomic and somatic reflexes is complex modifying supraspinal input from other central neuronal networks. These facilitory and inhibitory impulses, which originate from several areas of the nervous system, allow for the full conscious control of micturition in the adult. During voluntarily initiated micturition, the detrusor pressure becomes higher than the outlet pressure and certain adaptive changes occur in the shape of the bladder outlet with consequent passage of urine into and through the proximal urethra. Why do such changes not occur with increases in pressure that are similar in magnitude but produced only by changes in intraabdominal pressure (IAP), such as straining or coughing? First, a coordinated bladder contraction does not occur in response to such stimuli, clearly emphasizing the fact that increases in total intravesical pressure are by no means equivalent to emptying ability. Normally, for urine to flow into the proximal urethra, not only must there be an increase in intravesical pressure but the increase must also be a product of a coordinated bladder contraction, occurring through a neurally mediated reflex mechanism and associated with characteristic conformational and tension changes in the bladder neck and proximal urethral area. Assuming the bladder outlet is competent at rest, a major factor in the prevention of urinary leakage during increases in IAP is the fact that there is normally at least equal pressure transmission to the proximal urethra during such activity. Failure of this mechanism, generally associated with hypermobility of the bladder neck and proximal urethra (another way of describing pathologic descent with abdominal straining), is an almost invariable correlate of “genuine” stress or effort-related urinary incontinence (UI) in the female. No such hypermobility occurs in the male. The increase in urethral closure pressure that is normally seen with increments in IAP actually exceeds the extrinsic pressure increase. This indicates that active muscular function due to a reflex increase in striated sphincter activity and/or other factors such as midurethral compression that increase urethral resistance are also involved in preventing such leakage. A more complete description of the factors involved in sphincteric incontinence and its prevention can be found in the section on incontinence. Bladder accommodation during filling is a primarily passive phenomenon. It is dependent on the elastic and viscoelastic properties of the bladder wall and the lack of parasympathetic excitatory input. An increase in outlet resistance occurs via the striated sphincter somatic guarding reflex. In at least some species a sympathetic reflex also contributes to storage by (1) increasing outlet resistance by increasing tension on the smooth sphincter, (2) inhibiting bladder contractility through an inhibitory effect on parasympathetic ganglia, and (3) causing a decrease in tension of bladder body smooth muscle. Continence is maintained during increases in IAP by the intrinsic competence of the bladder outlet and urethral compression against a suburethral supporting layer. A further increase in striated sphincter activity, on a reflex basis, is also contributory during increases in IAP (e.g., by coughing or straining). Emptying (voiding) can be voluntary or involuntary and involves an inhibition of the spinal somatic and sympathetic reflexes and activation of the vesical parasympathetic pathways, the organizational center for which is in the brainstem. Initially, there is a relaxation of the outlet musculature, mediated not only by the cessation of the somatic and sympathetic spinal reflexes but probably also by a relaxing factor, very possibly NO, released by parasympathetic stimulation or by some effect of bladder smooth muscle contraction itself. A highly coordinated parasympathetically induced contraction of the bulk of the bladder smooth musculature occurs, with shaping or funneling of the relaxed outlet, due at least in part to a smooth muscle continuity between the bladder base and proximal urethra. With amplification and facilitation of the bladder contraction from other peripheral reflexes and from spinal cord supraspinal sources, and the absence of anatomic obstruction between the bladder and the urethral meatus, complete emptying will occur. Whatever disagreements exist regarding the anatomic, morphologic, physiologic, pharmacologic, and mechanical details involved in both the storage and expulsion of urine by the LUT, we believe that agreement is found regarding certain points. First, the micturition cycle involves two relatively discrete processes: bladder filling/urine storage and bladder emptying/voiding. Second, whatever the details involved, these processes can be summarized succinctly from a conceptual point of view. Bladder filling/urine storage requires the following: 2. A bladder outlet that is closed at rest and remains so during increases in IAP. 3. Absence of involuntary bladder contractions (detrusor overactivity [DO]) Bladder emptying/voiding requires the following: 2. A concomitant lowering of resistance at the level of the smooth and striated sphincter (absence of functional obstruction) 3. Absence of anatomic obstruction Any type of voiding dysfunction must result from an abnormality of one or more of the factors previously listed regardless of the exact pathophysiology involved. This division, with its implied subdivision under each category into causes related to the bladder and the outlet, provides a logical rationale for discussion and classification of all types of voiding dysfunction and disorders as related primarily to bladder filling/urine storage or to bladder emptying/voiding (Table 14-3 and Table 14-4). There are some types of voiding dysfunction that represent combinations of filling and storage and emptying and voiding abnormalities. Within this scheme, however, these become readily understandable, and their detection and treatment can be logically described. Further, using this scheme, all aspects of urodynamic, radiologic, and video urodynamic evaluation can be conceptualized as to exactly what they evaluate in terms of either bladder or outlet activity during filling and storage or emptying and voiding (see Table 14-6). Treatments for voiding dysfunction can be classified under broad categories according to whether they facilitate filling and storage or emptying and voiding and whether they do so by acting primarily on the bladder or on one or more of the components of the bladder outlet (see Tables 14-7 and 14-8). Finally, the individual disorders produced by various neuromuscular dysfunctions can be considered in terms of whether they produce primarily storage or emptying abnormalities or a combination. Table 14-4 Expanded Functional Classification of Lower Urinary Tract Dysfunction a. Involuntary contractions (detrusor overactivity [DO]) Bladder outlet obstruction (BOO; myogenic) Inflammation Idiopathic b. Decreased compliance Fibrosis Idiopathic c. Combination 2. Hypersensitivity b. Neurologic c. Psychologic d. Idiopathic 3. Underactivity (with retention and overflow incontinence) B. Because of the outlet 1. Stress urinary incontinence (SUI) a. Lack of suburethral support b. Pelvic floor laxity, hypermobility 2. Intrinsic sphincter deficiency (ISD) a. Neurologic disease or injury b. Fibrosis 3. Combination (SUI and ISD) C. Combination (bladder and outlet factors) D. Fistula II. Failure to empty A. Because of the bladder (underactivity) 2. Myogenic 3. Psychogenic 4. Idiopathic B. Because of the outlet b. Bladder neck contracture c. Urethral stricture, fibrosis d. Urethral compression 2. Functional a. Smooth sphincter dyssynergia (bladder neck dysfunction) b. Striated sphincter dyssynergia C. Combination (bladder and outlet factors) Table 14-6 Urodynamics Simplified This functional conceptualization of urodynamics categorizes each study as to whether it examines bladder or outlet activity during the filling/storage or emptying phase of micturition. In this scheme, uroflow and residual urine integrate the activity of the bladder and the outlet during the emptying phase. 1,2 Total bladder (Pves) and detrusor (Pdet) pressures during a filling cystometrogram (FCMG). 3 Filling cystometrogram. 4 Detrusor leak point pressure. 5 Urethral pressure profilometry. 6 Valsalva leak point pressure. 7 Fluoroscopy of outlet during filling/storage. 8,9 Total bladder and detrusor pressures during a voiding cystometrogram (VCMG). 10 Voiding cystometrogram. 11 Micturitional urethral pressure profilometry. 12 Fluoroscopy of outlet during emptying. 13 Electromyography of periurethral striated musculature. 14 Flowmetry. 15 Residual urine. Table 14-7 Therapy to Facilitate Urine Storage/Bladder Filling A. Behavioral therapy, including any or all of: 2. Bladder training 3. Timed bladder emptying or prompted voiding 4. Fluid restriction 5. Pelvic floor physiotherapy ± biofeedback B. Pharmacologic therapy (oral, intravesical, intradetrusor) 2. Beta-adrenergic agonists 3. Botulinum toxin 4. Drugs with mixed actions 5. Calcium antagonists 6. Potassium channel openers 7. Prostaglandin inhibitors 8. Alpha-adrenergic antagonists 9. Tricyclic antidepressants; serotonin and norepinephrine reuptake inhibitors 10. Dimethyl sulfoxide (DMSO) 11. Polysynaptic inhibitors 12. Capsaicin, resiniferatoxin, and similar agents C. Bladder overdistention D. Electrical stimulation (sacral neuromodulation, posterior tibial and other peripheral nerve stimulation) E. Acupuncture and electroacupuncture F. Interruption of innervation 1. Very central (subarachnoid block) 2. Less central (sacral rhizotomy, selective sacral rhizotomy) 3. Peripheral motor or/and sensory G. Augmentation cystoplasty (auto, bowel, tissue engineering) II. Outlet related (increasing outlet resistance) A. Behavioral therapy, including any or all of: 2. Bladder training 3. Timed bladder emptying or prompted voiding 4. Fluid restriction 5. Pelvic floor muscle training ± biofeedback B. Electrical stimulation C. Pharmacologic therapy 2. Tricyclic antidepressants; serotonin and norepinephrine reuptake inhibitors 3. Beta-adrenergic antagonists, agonists D. Vaginal and perineal occlusive and/or supportive devices; urethral plugs E. Nonsurgical periurethral bulking 1. Synthetic bulking agents, tissue engineering F. Retropubic vesicourethral suspension ± prolapse repair (female) G. Sling procedures ± prolapse repair (female) H. Midurethral tapes ± prolapse repair (female) I. Perineal sling procedure (male) J. Artificial urinary sphincter K. Myoplasty (muscle transposition) L. Bladder outlet closure III. Circumventing the problem B. External collecting devices C. Antidiuretic hormonelike agents D. Short-acting diuretics E. Intermittent catheterization F. Continuous catheterization G. Urinary diversion Table 14-8 Therapy to Facilitate Bladder Emptying/Voiding I. Bladder related (increasing intravesical pressure or facilitating bladder contractility) A. External compression, Valsalva B. Promotion or initiation of reflex contraction 2. Bladder “training,” tidal drainage C. Pharmacologic therapy (oral, intravesical) 2. Prostaglandins 3. Blockers of inhibition a. Alpha-adrenergic antagonists b. Opioid antagonists D. Electrical stimulation 1. Directly to the bladder or spinal cord 2. Directly to the nerve roots 3. Intravesical (transurethral) 4. Sacral neuromodulation E. Reduction cystoplasty F. Bladder myoplasty (muscle wrap) II. Outlet related (decreasing outlet resistance) A. At a site of anatomic obstruction 1. Pharmacologic therapy — decrease prostate size or tone a. Alpha-adrenergic antagonists b. 5-alpha-reductase inhibitors c. Luteinizing hormone-releasing hormone agonists/ antagonists d. Antiandrogens 2. Prostatectomy, prostatotomy (diathermy, heat, laser) 3. Bladder neck incision or resection 4. Urethral stricture repair or dilation 5. Intraurethral stent 6. Balloon dilation of stricture/contracture B. At level of smooth sphincter a. Alpha-adrenergic antagonists 2. Transurethral resection or incision 3. Y-V plasty C. At level of striated sphincter 1. Behavioral therapy ± biofeedback 2. Pharmacologic therapy b. Baclofen c. Dantrolene d. Alpha-adrenergic antagonists e. Botulinum toxin (injection) 3. Urethral overdilation 4. Surgical sphincterotomy 5. Urethral stent 6. Pudendal nerve interruption III. Circumventing the problem A. Intermittent catheterization B. Continuous catheterization C. Urinary diversion (conduit or reservoir) The pathophysiology of failure of the LUT to fill with or store urine adequately or to empty adequately must logically be secondary to reasons related to the bladder, the outlet, or both. Absolute or relative failure of the bladder to fill with and store urine adquately results from bladder overactivity (involuntary contraction and/or decreased compliance), decreased outlet resistance, heightened or altered sensation, or a combination. 2. Outlet underactivity Stress or effort-related UI is a condition that arises primarily from damage to muscles and/or nerves and/or connective tissue within the pelvic floor. Urethral support is important in the female, the urethra normally being supported by the action of the levator ani muscles through their connection to the endopelvic fascia of the anterior vaginal wall. Damage to the connection between this fascia and this muscle, damage to the nerve supply, or direct muscle damage can therefore influence continence. Bladder neck function is likewise important, and loss of normal bladder neck closure can result in incontinence despite normal urethral support. In older writings, the urethra was sometimes ignored as a factor contributing to continence in the female, and the site of continence was thought to be exclusively the bladder neck. However, in approximately 50% of continent women, urine enters the urethra during increases in abdominal pressure. The continence point in these women (highest point of pressure transmission) is at the midurethra. Urethral hypermobility implies weakness of the pelvic floor supporting structures. During increases in IAP, there is descent of the bladder neck and proximal urethra. If the outlet opens concomitantly, SUI ensues. In the classic form of urethral hypermobility, there is rotational descent of the bladder neck and urethra. However, the urethra may also descend without rotation (it shortens and widens), or the posterior wall of the urethra may be pulled (sheared) open while the anterior wall remains fixed. However, urethral hypermobility is often present in women who are not incontinent, and thus the mere presence of urethral hypermobility is not sufficient to make a diagnosis of a sphincter abnormality unless UI is also demonstrated. The “hammock hypothesis” of John DeLancey (1994) proposes that for stress incontinence to occur with hypermobility, there must be a lack of stability of the suburethral supportive layer. This theory proposes that the effect of abdominal pressure increases on the normal bladder outlet, if the suburethral supportive layer is firm, is to compress the urethra rapidly and effectively. If the supportive suburethral layer is lax and/or movable, compression is not as effective. Intrinsic sphincter dysfunction denotes an intrinsic malfunction of the urethral sphincter mechanism itself. In its most overt form, it is characterized by a bladder neck that is open at rest and a low Valsalva leak point pressure (VLPP) and urethral closure pressure and is usually the result of prior surgery, trauma with scarring, or a neurologic lesion. Urethral instability refers to the rare phenomenon of episodic decreases in outlet pressure unrelated to increases in bladder or abdominal pressure. The term urethral instability may be a misnomer because many believe that the drop in urethral pressure represents simply the urethral component of a normal voiding reflex in an individual whose bladder does not measurably contract because of either myogenic or neurogenic reasons. Little has appeared in the literature about this entity since the last edition of this text. It is sometimes cited as a cause of urgency and urgency incontinence but can be seen in a “normal woman.” In theory at least, categories of outlet-related incontinence in the male are similar to those in the female. Sphincteric incontinence in the male is not, however, associated with hypermobility of the bladder neck and proximal urethra but is similar to what is termed ISD in the female. It is seen most often after radical prostatectomy or in neurologic disease or trauma affecting the sacral spinal cord or pathways distal to this. It is seen occasionally after outlet-reducing surgery as well. There is essentially no information regarding the topic of urethral instability in the male. The treatment of filling/storage abnormalities is directed toward inhibiting bladder contractility, decreasing sensory output, mechanically increasing bladder capacity, and/or toward increasing outlet resistance, the latter either continuously or just during increases in IAP. Absolute or relative failure to empty the bladder results from decreased bladder contractility (a decrease in magnitude, coordination, or duration), increased outlet resistance, or both. 2. Outlet overactivity or obstruction
Lower Urinary Tract Function and Dysfunction; Urinary Incontinence
Introduction
Relevant lower urinary tract anatomy, physiology, pharmacology, and terminology
Bladder, urethra, smooth and striated sphincter
Innervation and receptor function
Excitation-contraction coupling in bladder smooth muscle has been classically described as mediated by mobilization of intracellular calcium via activation of phosphoinositide hydrolysis. The cytosolic calcium binds to calmodulin, initiating the cascade of events necessary to phosphorylate myosin and cause contraction. Relaxation is mediated by a decrease in intracellular calcium. This is accomplished by extrusion extracellularly or reuptake into intracellular stores. Smooth muscle relaxation can also be produced by causing intracellular potassium efflux, resulting in membrane hyperpolarization. Both of these latter actions are potential pharmacologic targets for decreasing bladder contractility. Unfortunately, there are no bladder-selective calcium channel blockers or potassium channel openers. Recently it has also been proposed that a main pathway for activation of bladder contraction is by calcium influx through L-type calcium channels and increased sensitivity to calcium of the contractile machinery by inhibition of myosin light chain phosphatase through activation of Rho kinase.
The term cholinergic refers to those receptor sites where acetylcholine is a primary neurotransmitter. Peripheral cholinergic fibers include somatic motor fibers, all preganglionic autonomic fibers, and all postganglionic parasympathetic fibers. The cholinergic receptor sites on autonomic effector cells are termed muscarinic. Atropine and its congeners competitively inhibit muscarinic receptor sites. Cholinergic receptor sites on autonomic ganglia and on motor end plates of skeletal muscle are designated nicotinic. These are not atropine sensitive.
The term adrenergic is applied to those receptor sites where a catecholamine is the neurotransmitter. Most postganglionic sympathetic fibers are adrenergic receptor sites, including those to LUT smooth muscle, where the catecholamine responsible for neurotransmission is norepinephrine. Adrenergic receptor sites are further classified as alpha (α) or beta (β) on the basis of the differential effects elicited by a series of catecholamines and their antagonists. Classically, the term α-adrenergic effect designates vasoconstriction and/or contraction of smooth musculature in response to norepinephrine. These effects are inhibited by phentolamine, phenoxybenzamine, prazosin, and related compounds. The term β-adrenergic effect implies smooth muscle relaxation in response to catecholamine stimulation and also includes cardiac stimulation, vasodilation, and bronchodilation. These effects are stimulated most potently by isoproterenol (much more so than by norepinephrine) and antagonized by multiple β-blocker compounds, of which propranolol is a prototype.
Receptor subtyping is a relevant concept that explains why some neurotransmitters have differing effects in different organs or anatomic localizations. Subtyping can be based on functional assays, radioligand-binding affinity, or on cloning established genotypes. For instance, there are five different muscarinic receptor subtypes (M1-M5). Although it appears that the majority of these in human bladder smooth muscle are of the M2 subtype, bladder smooth muscle contraction is mediated primarily by the M3 subtype. There are multiple subtypes of α- and β-adrenergic receptors as well. These are generally tissue specific and explain the selectivity of certain responses. The concept also underlies the development of relatively receptor subtype selective drugs for the treatment of certain LUT dysfunctions.
Alpha-adrenergic receptors (α-ARs) can be found in the detrusor smooth muscle, the vasculature, afferent and efferent nerve terminals and intramural ganglia. The predominant receptor subtypes are α1D and α1a, but the total α-1 AR expression is low, and they do not seem to have a significant role in an emptying bladder contraction. Their density is highest in the bladder base (the area circumferentially below the entrance of the ureter) posteriorly, bladder neck, and proximal urethral where they are thought to play a role in the increasing outlet resistance that occurs during bladder filling/storage.
There are three subtypes of β-ARs, the most predominant in the bladder being β3, and the response to activation is detrusor smooth muscle relaxation. Beta-adrenergic receptors (β-ARs) predominate in the bladder body (area above the ureteral entrances circumferentially) and are less dense in the base and proximal urethra.
Adding to the complexity is the fact that neurotransmitters may have no effect or differing effects at different sites (i.e., brain, pons, spinal cord, efferent ganglia, presynaptic and postsynaptic neural effector junction, sensory afferent fibers, ganglia). In this chapter, we concentrate on peripheral smooth muscle actions.
Transmitter (Receptor)
Effect
Site of Action
Acetylcholine (M3)
Contraction
Bladder smooth muscle
Acetylcholine (M3, M2)
Excitation
Peripheral afferents
Acetylcholine (M2)
Contraction
Bladder smooth muscle
Acetylcholine (M1, M3)
Contraction
Prejunctional
Acetylcholine (M2, M4)
Relaxation
Prejunctional
Norepinephrine (ß3)
Relaxation
Bladder smooth muscle
Norepinephrine (α1)
Contraction
Bladder smooth muscle
Adenosine triphosphate (P2X1)
Contraction
Bladder smooth muscle
Adenosine triphosphate (P2X3)
Excitation
Peripheral afferents
Nitric oxide (NO)
Relaxation
Bladder base smooth muscle
Nitric oxide (NO)
Inhibition
Peripheral afferents
Serotonin (5-HT1, 5-HT2)
Contraction
Bladder smooth muscle
Prostanoids
Contraction
Bladder smooth muscle
Prostanoids
Excitation
Peripheral afferents
Leukotrienes (LTB4)
Contraction
Bladder smooth muscle
Angiotensin (AT1)
Contraction
Bladder smooth muscle
Bradykinin (B2)
Contraction
Bladder smooth muscle
Endothelin (ETa)
Contraction
Bladder smooth muscle
Tachykinins (NK2)
Contraction
Bladder smooth muscle
Vasopressin (V1)
Contraction
Bladder smooth muscle
Vasoactive intestinal peptide (VPAC1/VPC2)
Relaxation
Bladder smooth muscle
Parathormone
Relaxation
Bladder smooth muscle
The afferents traveling in the pelvic nerve are primarily responsible for the initiation of the micturition reflex in the normal state. Myelinated A-delta fibers normally subserve this function and convey mechanoreceptor input. Unmyelinated C fiber afferents are more prevalent but remain relatively silent during normal filling and storage. These become “awakened” and functional under various conditions (i.e., responses to distention after spinal cord injury [SCI], to cold — the “ice water test,” to nociceptive stimuli). Myelinated somatic afferents from the striated sphincter travel in the pudendal nerve. Afferents also travel in the hypogastric nerve, but little is known about their specific function. The most important afferents for initiating and maintaining normal micturition are those in the pelvic nerve, relaying to the sacral spinal cord. These convey impulses from tension, volume, and nociceptors located in the serosal, muscle, and urothelial and suburothelial layers of the bladder and urethra. In a neurologically normal adult, the sensations of filling and distention are what develops during filling/storage and initiate the reflexes responsible for emptying/voiding.
Additionally, there is general agreement that, at least in certain animal models (the cat, primarily), there is a significant inhibitory influence exerted on parasympathetic ganglionic transmission by postganglionic sympathetic fibers (see Figure 14-1 and 14-2). Those who advocate a major role of the SYMPNS in the micturition cycle summarize the influences as primarily to facilitate the filling and storage phase of micturition by three mechanisms.
It should be noted, however, that some researchers are of the opinion that the SYMPNS plays a very minor role in the micturition cycle in the normal human although acknowledging that the adrenergic receptors can be activated pharmacologically.
CNS influences on micturition
Neurotransmitter
Site
Predominant Action
Glutamate
Brain, SC
+
Glycine
Brain, SC
–
GABA (gamma-amino-butyric acid)
Brain, SC
–
Serotonin
SC
–
Acetylcholine
Brain
±
Dopamine (D-2)
Brain
+
Dopamine (D-1)
Brain
–
Norepinephrine (α-1, α-2)
Brain, SC
±
Tachykinins (NK1, NK2)
Brain, SC
+
Normal lower urinary tract function
What determines bladder response during filling?
What determines outlet response during filling?
Why does voiding ensue with a normal bladder contraction?
Why does urinary continence persist during abdominal pressure increases?
Overview of the micturition cycle: simplification and summary
Bladder
Outlet
Filling/storage phase
Pves1 Pdet2 (FCMG3)
DLPP4
UPP5
VLPP6
FLUORO7
MUPP11
Emptying phase
Pves8 Pdet9 (VCMG)10
FLUORO7
MUPP11
FLUORO12
EMG13
__________ FLOW14
__________ RU15
____________
____________
Abnormalities of filling/storage and emptying/voiding: overview of pathophysiology (Tables 14-3 and 14-4)
Filling/storage failure
Decreased outlet resistance may result from any process that damages the innervation or structural elements of the smooth and/or striated sphincter or support of the bladder outlet. This may occur with neurologic disease or injury, surgical or other mechanical trauma, or aging. Classically, sphincteric incontinence in the female was categorized into relatively discrete entities: (1) so-called genuine stress incontinence (GSI) and (2) intrinsic sphincter deficiency (ISD), originally described as type III stress incontinence. GSI in the female was described as associated with hypermobility of the bladder outlet because of poor pelvic support and with an outlet that was competent at rest but lost its competence only during increases in IAP. ISD described a nonfunctional or very poorly functional bladder neck and proximal urethra at rest. The implication of classical ISD was that a surgical procedure designed to correct only urethral hypermobility would have a relatively high failure rate, as opposed to one designed to improve urethral coaptation and compression. The contemporary view is that the majority of cases of effort-related incontinence in the female involve varying proportions of support-related factors and ISD. It is possible to have outlet-related incontinence due only to ISD but not due solely to hypermobility or poor support — some ISD must exist.
Emptying/voiding failure
Pathologically increased outlet resistance is much more common in men than in women. Although it is most often secondary to anatomic obstruction, it may be secondary to a failure of relaxation or due to active contraction of the striated or smooth sphincter during bladder contraction. Striated sphincter dyssynergiaStay updated, free articles. Join our Telegram channel

Full access? Get Clinical Tree
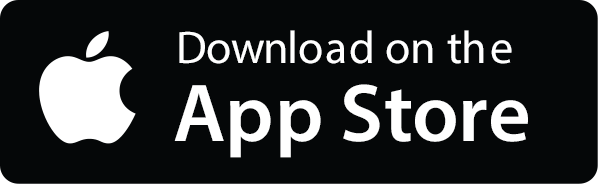
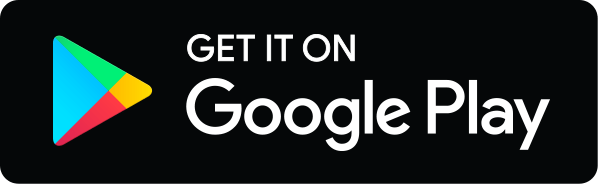