© Springer International Publishing AG Switzerland 2015
Luca Aldrighetti, Francesco Cetta and Gianfranco Ferla (eds.)Benign Tumors of the Liver10.1007/978-3-319-12985-3_55. Imaging Modalities
Francesco De Cobelli1 , Gabriele Ironi1 , Paolo Marra1 , Antonio Esposito1 and Alessandro Del Maschio1
(1)
Department of Radiology, San Raffaele Hospital, Via Olgettina, 60, 20132 Milan, Italy
Keywords
Imaging modalitiesUltrasoundComputed tomographyMagnetic resonanceBenign liver lesionsDetecting and characterizing focal liver lesions is one of the most controversial challenges in imaging today, as the differential diagnosis is wide.
Imaging diagnostics of the liver are hampered by the fact that 20–50 % of the population exhibits benign focal liver lesions, mostly cysts and hemangiomas, lowering the pretest probability of identifying malignant instances [1]. In addition, MRI, CT, and ultrasound provide increasing spatial resolution, improving the likelihood of disclosing very small changes with uncertain significance.
Due to the complexity of liver focal disease, imaging evaluation must follow the investigation of patient clinical background with particular attention given to concomitant findings such as specific laboratory abnormalities, history of infections or toxic exposure, and underlying systemic diseases or known malignancies. Taking into account the broad prevalence and incidence of focal liver lesions, clinical imaging and differential diagnosis should primarily focus on the most common entities and thereafter consider the more rare entities. Due to the high prevalence of benign tumors, imaging of the liver is challenging in terms of distinguishing benign liver lesions, which in most instances can be left alone, from malign neoplasms requiring therapy. By far the most common entity is hemangioma with a prevalence of 2–20 % [1, 2] in general population, followed far behind by focal nodular hyperplasia (FNH) and liver adenoma, which is a rather rare entity. From a practical point of view, a confident diagnosis of hemangioma and FNH resolves 90 % of all focal benign liver lesions [3]. However, liver adenoma has to be excluded, although being benign, since it is often a candidate for surgical resection due to the risk of bleeding and malignant transformation.
Cross-sectional imaging modalities , such as US, CT, and MR imaging, are used in most centers to assess liver abnormalities. These modalities, often used in various combinations, have fundamental differences in data acquisition and hence differences in the type of physical characteristics of tissues that they scan.
5.1 Ultrasound
Ultrasound (US) is usually the initial imaging modality for the assessment of upper abdomen including the liver. Because of the high incidence of benign liver abnormalities in the general population, the initial US for many patients is not the final diagnostic tool but the beginning of an extensive work-up that can evolve into many directions (US-guided biopsy, CT or MR imaging). The main advantages of hepatic sonography include its ability to guide biopsy, its ability to characterize common benign lesions (cysts, hemangiomas), and its safety and low cost (both from biological and social point of view). Limits include its inability to image the entire liver in some patients and lower accuracy compared to CT and MR in the detection and characterization of focal liver lesions.
The ability of US to detect a focal lesion depends on its size, location, echogenicity, and mass effect. Small echogenic lesions are easily detectable, while large isoechoic masses are more difficult to diagnose. Mass effect is more important for the diagnosis of large isoechoic lesions, and it may appear as deviation or invasion of intrahepatic vasculature, bulges on liver margins, or displacement of extrahepatic structures. The characterization of focal liver lesions and thus the specificity of US rely on grayscale and Doppler appearances [4].
The liver is best evaluated using a probe with a center frequency ranging from 2 to 5 MHz; higher-frequency transducers may be used to image superficial abnormalities of the liver surface. The left lobe is optimally scanned through a subxyphoid approach with the patient in supine decubitus, while the right lobe can be approached either subcostally or intercostally. Intercostal scanning should be performed in a supine position either during quiet respiration or breathhold on expiration to allow visualization of the superior regions of the liver and subphrenic area. The subcostal approach is best performed with the patient in the left lateral decubitus position in deep inspiration allowing insonation of the majority of the right lobe.
The first US level of liver evaluation is the traditional standard brightness mode (B-mode) a grayscale examination in which no Doppler is used.
The second level superimposes a color Doppler interrogation on the region of interest. This level produces an image that shows blood flow in vessels: color pixels are integrated into the conventional B-mode grayscale images. Color Doppler displays blood flow toward the transducer as red and blood flow away from the transducer as blue. The intensity of color encoding enables the examiner to distinguish between slow flow and fast flow. Additional benefits include direct visualization of turbulent flow by a mixture of the two colors (phenomena seen in arteriovenous malformations). The amount of color pixels in a lesion correlates with the degree of vascularization. Additionally, color coding of vessels allows guided placement of cursors for spectral Doppler imaging [5].
The third level superimposes a small interrogation region, called “sample volume,” over a vessel of interest. Ideally, the sample volume should be placed in the midportion of the lumen, rather than toward the periphery, for optimal estimation of laminar flow. Targeted interrogation of the vessel produces a spectral Doppler waveform that is displayed at the bottom of the screen, while the color Doppler image is at the top. Every spectral waveform has morphologic features that provide information regarding direction, velocity, and acceleration. Directional information is determined on the basis of whether the waveform lies above or below the baseline; velocity information is determined on the basis of distance from the baseline at any given point of the curve; and acceleration information is obtained from the slope of the curve, with changes in acceleration being marked by waveform peaks, or inflection points. These inflection points (acceleration changes) generate characteristic audible sounds at Doppler US [5].
Conventional color Doppler and spectral Doppler are not always able to image the vascularity of small lesions because in some cases signals are under level of detection. This may be partially correct with the use of power Doppler and contrast agents.
Power Doppler imaging displays the integrated Doppler signal power and displays tumor vascularity in an angiogram-like appearance. Due to better noise differentiation, this technique demonstrates small tumor vessels more precisely. However, power Doppler does not encode flow direction.
Tissue harmonic imaging is now an important part of liver US evaluation because it provides extra information, altering management and revealing lesions not seen on conventional B-mode [6, 7]. Tissue harmonic imaging is a grayscale imaging technique that uses the information from harmonic signals (multiples of the insonating frequency) generated by nonlinear propagation of a sound wave as it passes through the tissue. While conventional US transmits and receives at the same frequency, in tissue harmonic imaging, the receiver separates the harmonic signal from the fundamental echoes. This results in the use of only the returning harmonic signal with a dramatic reduction in artifacts.
Sonography can effectively guide biopsy allowing real-time visualization of the needle tip as it moves toward the lesion. This makes biopsy of small lesions and lesions in uncooperative patients easier. If a lesion can be imaged, biopsy is generally more efficient and cost effective using sonographic guidance, even when initially detected with some other modalities [8].
The introduction of US contrast agents has had a remarkable impact on liver sonography, and they are being increasingly used for the evaluation of focal liver lesions. Contrast-enhanced US (CEUS) allows masses to be interrogated for the degree and distribution of enhancement during the early phases and the washout of contrast during the later phases. Unlike Doppler US, which can only image the macrocirculation, CEUS allows assessing of both macro- and microcirculation of lesions. Modern US contrast agents are gas microbubble contained within a stabilizing lipid shell. Microbubbles are less than 10 μm in diameter and are safe and effective ultrasound scatterers because of the large impedance difference between the surrounding fluid (e.g., blood) and the encapsulated gas. When given intravenously, microbubbles produce marked enhancement of the US signal for several minutes, in gray scale and Doppler mode. The interactions of microbubbles with US beam are complex [4]. When a microbubble is exposed to an oscillating acoustic signal, it undergoes alternate expansion and contraction. At low acoustic power, these alternate expansions and contractions are equal and symmetrical, and the frequency of scattered signal is unaltered. As the acoustic power increases, more complex nonlinear interactions occur as the expansion and contraction phases become unequal because the microbubbles resist compression more strongly than expansion. This nonlinear resonant behavior is associated with the production of harmonic signals at multiples (or fractions) of the insonating frequency and can be imaged with harmonic imaging techniques. At still higher powers, highly nonlinear behavior is associated with microbubble disruption. This disruptive phase can be imaged using color Doppler mode or in grayscale. Microbubble disruption is seen as a transient bright color or B-mode signal which persists only for a few frames after insonation of an individual area. Contrast-enhanced nonlinear imaging of focal liver lesions can be performed during the vascular phase assessing the dynamic enhancement pattern and the vascular morphology of the lesion. The signal enhancer is injected intravenously and is purely intravascular without the extracellular phase of CT and MR contrast agents. Its effect becomes almost immediately noticeable a few seconds after bolus injection and persists for 90 up to 180 s. Following injection, continuous scanning can be performed and, although the timing is dependent on the individual’s cardiac function, it can be broadly divided into an arterial phase (approximately 10–25 s), portal venous phase (approximately 30–120 s), and a late phase (over 120 s) [9]. Due to high sensitivity of modern scanners, the supplied vials of contrast agent can be divided and administered as small boluses, each followed by a saline flush of 5–10 ml. This allows multiple injections to be used in the same session from one vial. The injections can be repeated after 5–10 min, allowing additional lesions to be analyzed [10]. Several studies have already demonstrated a dramatic improvement of diagnostic performance of CEUS in the detection and characterization of focal liver lesions when compared with baseline US, with reported sensitivity and specificity values approaching those of CT and MRI [11–17].
5.2 Computed Tomography
The broad availability of computed tomography (CT) and recent development and implementation of faster multi-row detector machines make this modality an excellent tool for the detection and characterization of focal liver lesions. CT is still the most commonly used modality for the evaluation of focal liver lesion after initial sonography.
The indication for CT evaluation of the liver include the detection and characterization of focal liver lesions, detection and characterization of diffuse liver disease, staging of an intrahepatic or extrahepatic malignancy, follow-up after treatment for an intrahepatic or extrahepatic malignancy, and image guidance for percutaneous drainage or biopsy. However, it should be noted that percutaneous biopsy of liver lesion is an uncommon indication for CT, since more than 90 % of these biopsies can be performed using ultrasound guidance.
Multidetector-row CT (MDCT) is a technological evolution that allows the simultaneous acquisition of multiple images during a single rotation of the X-ray tube. With MDCT the entire liver is rapidly imaged (in about 5 s) with thin collimation (1–3 mm) during a single breathhold. MDCT scanners allow acquisition of thin slices with isotropic voxel size. The advantages of thinner slices are less partial volume averaging and, more importantly, multiplanar reconstructions of superior quality (MPR) and true three-dimensional reconstructions . There have been controversial data in the literature about the role of thin-slice imaging for the detection and characterization of liver lesions. The benefits and limitations of varying slice thickness in small liver lesion diagnosis have been investigated: A 2- and 4-mm slice reconstruction provided improved diagnostic results for the assessment of small liver lesions sized 11 mm or less. Larger section of 6, 8, and 10 mm yielded significantly inferior detection rates as the partial volume effects become more significant at lower resolution. However 1-mm slices also turned out to be inferior because the benefit of higher resolution is counteracted by the marked decrease in signal-to-noise ratio at lowest beam collimation [18].
The use of thin slices produces a data set that can be manipulated and reviewed on a workstation to produce high-quality multiplanar reformations. Superior quality MPRs obtained in various planes (coronal, sagittal, or arbitrarily angulated sections) may improve the detection of small subcapsular lesions in the dome of the liver, show the feeding arteries and venous drainage of detected lesions, or help in the characterization of lesions by better evaluation of their enhancement patterns. Examples include peripheral nodules of enhancement of small hemangiomas or central scars in focal nodular hyperplasia [19–21]. Thus, the peripheral nodular enhancement of hepatic hemangiomas may be indeterminate at transverse CT alone, while in the sagittal or coronal planes, this nodular enhancement may be more clearly demonstrated, allowing a confident diagnosis of hemangioma. In addition, the anatomic coronal plane may even help surgeons for preoperative planning, through delineation of the relationship of the lesions with blood vessels and bile ducts [22].
The detection of liver lesions relies on creation of images that provide optimum liver-to-tumor contrast. The shorter scan times also allow the capture of distinct phases of contrast enhancement following the bolus administration of iodinated contrast material through a peripheral vein, typically in the arm. These phases provide important informations concerning the enhancement patterns and hence the possibility of characterization of focal liver lesions. Although unenhanced CT is not really a phase of enhancement, some lesions are readily apparent, especially those that are larger and/or more peripheral. Foley et al. [23] described the different phases of liver enhancement.
The first phase of enhancement that occurs following the intravenous contrast administration of iodinated contrast material is the early hepatic arterial phase, also termed the hepatic arterial phase [23]. This phase begins approximately 15 s after initiating the bolus and lasts for about 10–15 s. During the early arterial phase, there is vivid arterial enhancement only, with essentially no enhancement of hepatic parenchyma and portal system. Although this phase is advantageous for CT angiography, it is not useful for detecting hepatic tumors.
The next phase of enhancement is the late hepatic arterial phase, termed the portal venous inflow phase [23]. This phase begins approximately 30 s after initiating the bolus and lasts for about 15–20 s. During the late arterial phase, there are marked, although slightly less, arterial enhancement, early hepatic parenchymal enhancement, early portal venous enhancement, and no enhancement of the hepatic veins. This phase is best for detecting tumors that have a higher degree of vascularity, which tend to show vivid enhancement. By comparison, tumors that have lower degree of vascularity demonstrate little enhancement during this phase and are not well seen. For this reason, it is rarely advantageous to include the late arterial phase in patients with less vascular tumors.
The following phase of enhancement is the portal venous phase, which is termed the hepatic venous phase [23]. This phase begins approximately 60 s after initiating the bolus and lasts for about 45–60 s. During the venous phase there are less arterial enhancement, vivid hepatic parenchymal enhancement, vivid portal venous enhancement, and vivid hepatic venous enhancement. Tumors that have a lower degree of vascularity are well visualized as hypoattenuating masses during this phase. Tumors that have a higher degree of vascularity may have one of three different appearances during this phase [24–26]: they may continue to be hyperdense; they may be isoenhancing and disappear; or they may be hypoenhancing. Imaging the portal venous phase is important in all patients with suspected hepatic tumors, regardless of the degree of vascularity.
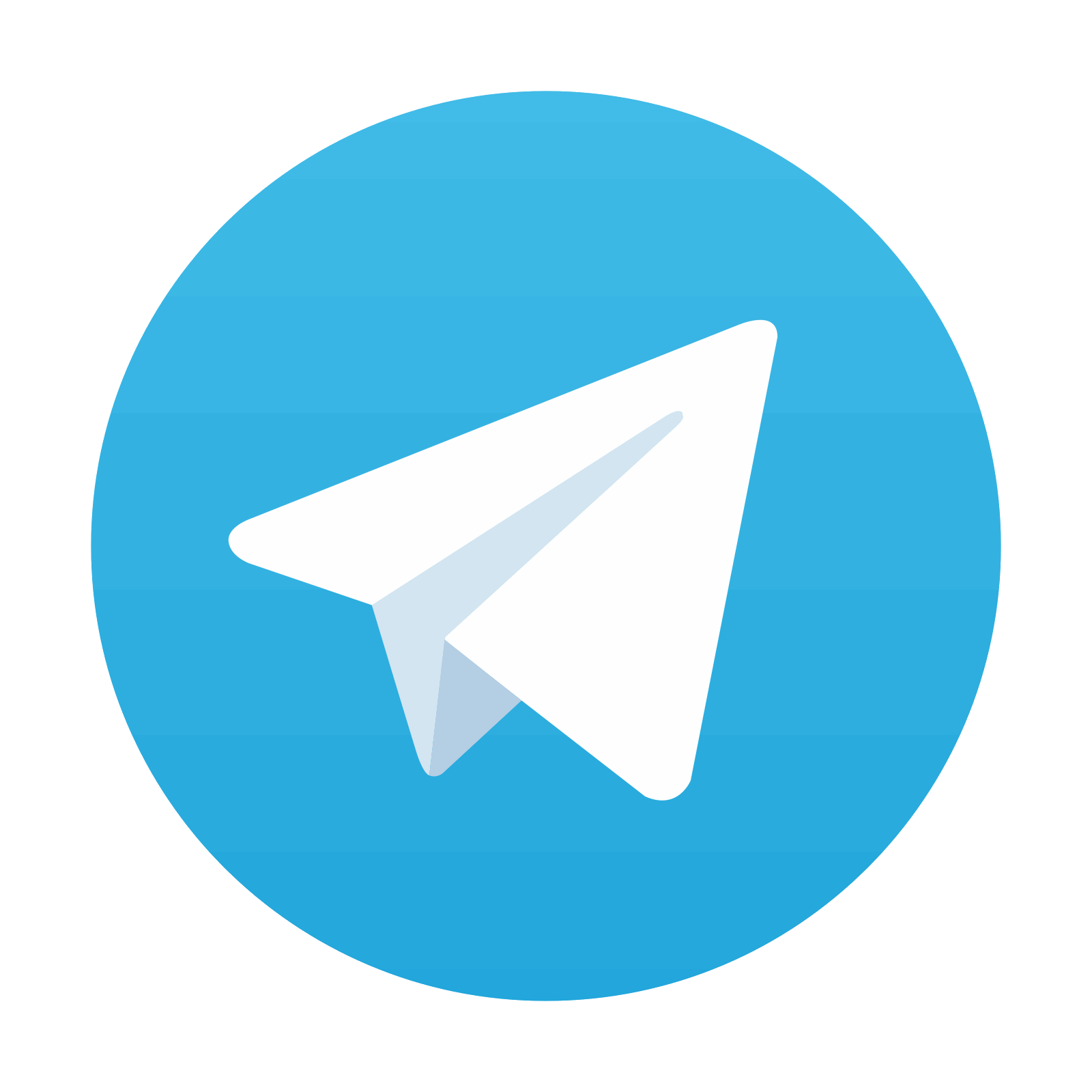
Stay updated, free articles. Join our Telegram channel

Full access? Get Clinical Tree
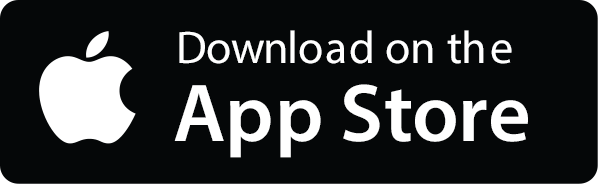
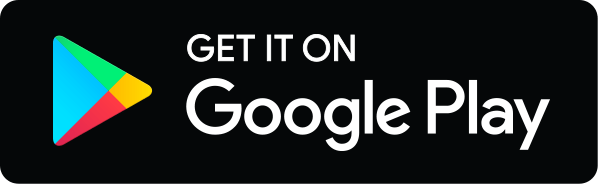