Clinical usage of magnetic resonance imaging. DCE dynamic contrast-enhanced; ASL arterial spin labeling; DWI diffusion-weighted imaging; DTI diffusion tensor imaging; BOLD blood oxygen level-dependent; MRE magnetic resonance elastography; P MR P magnetic resonance spectroscopy; GBCA gadolinium-based contrast agents
8.4.1 Dynamic Contrast-Enhanced (DCE)
Gadolinium-based contrast agents (GBCA) are used worldwide for DCE-MRI examinations. The tissue containing GBCA exhibits shortened tissue T1 and T2∗ values, through the so-called relaxation effect. By acquiring a series of dynamic tissue T2∗ data, an MR signal-time curve is depicted, which is typically used to describe the exchange rates between compartments (e.g., GFR) and hemodynamic parameters such as RBF, renal blood volume, MTT, and regional filtration fraction, within more sophisticated models that involve two or more compartments. Therefore, DCE-MRI could be useful for the assessment of parenchymal perfusion, including dysregulation, atherosclerosis, and microvascular rarefaction. Another application of DCE-MRI images is noninvasive estimation of GFR. Contrast media in the blood rapidly circulates through the parenchymal vasculature, but slowly accumulates in the tubule, which is similar to the behavior of other body fluids; thus, it can be used to calculate the single-kidney GFR by mathematical modeling. A correlation has been reported between standard radioisotope measures and the DCE-MRI approach [23].
However, the limitations of this technique are obvious. (1) Nephrotoxicity of the contrast agent: GBCAs have been reported to cause nephrogenic systemic fibrosis and gadolinium body storage [24, 25], and they are primarily excreted from the body by the kidneys; thus, safety should be thoroughly considered in cases with severe renal dysfunction. (2) DCE-MRI requires an extensive duration (3–10 min), such that it is clearly impacted by respiratory motion [26]. (3) Lacking standard mathematics models and internal controls, the sensitivity and specificity of this technique must be considered. It has been reported that DCE-MRI is reliable in cases with severe renal injury (e.g., artery stenosis >80%) [27]. There remains a gap between DCE-MRI results and “real” renal perfusion and function.
8.4.2 Arterial Spin Labeling (ASL)
ASL-MRI is an imaging technique to obtain a series of perfusion-weighted images by using inflowing blood as an endogenous contrast agent, which temporarily alters blood flow magnetization. ASL is primarily used for measurement of cortex perfusion, but rarely for such measurement of the medulla. Recently, ASL was applied to assess renal perfusion in patients with metabolic syndrome, and to detect hemodynamic responses to pharmacologic interventions [28]. However, because of its low signal-to-noise ratio (SNR), and because it requires complex imaging sequences and mathematical modeling to analyze the resulting data, ASL-MRI remains largely used for research.
8.4.3 Diffusion-Weighted Imaging (DWI)
DWI-MRI is a powerful imaging technique to provide diffusivity information by detecting random Brownian motion of water molecules in the kidneys. In DWI-MRI, water molecules accumulate in a strong magnetic gradient field (positive diffusion gradient), and un-accumulate in a second gradient field (negative diffusion gradient). When moving between these two fields, water molecules in motion exhibit an MR signal loss; when water molecules exhibit greater freedom of movement, a greater signal loss occurs. By adjusting the applied gradient pulse with respect to different diffusion sensitivities (b-values, where a higher b-value requires stronger diffusion weighting), the signal intensity-time curve is fitted to calculate the apparent diffusion constant (ADC), a quantitative measure of MR signal loss. Lower ADC values indicate slower free water movement, which is more common in pathological conditions.
DWI-MRI has shown high potential for use in a variety of acute and chronic kidney diseases. In CKD, the ADC value is reduced in both cortex and medulla, compared with healthy kidneys; it correlates with kidney injury in histological analysis of core biopsy specimens [29–31]. There is a statistically significant association between reduced ADC values and deterioration of renal function [32]. Paired comparisons show that ADC values exhibit statistically significant differences among CKD stages; the sensitivity and specificity were 75.44% and 69.81% to detect CKD stages 3–5 [33]. Several factors might lead to alterations of ADC value, such as the reduction of glomerular filtration and tubular reabsorption functions, and the accumulation of cellularity and extracellular matrixes.
The advantages of this technique are obvious: it does not require exogenous contrast agents and it acquires images in a short time (<1 min), within a few breath-hold intervals, such that it is minimally affected by breath movement. However, DWI-MRI remains limited in research use, and the “true” meaning of ADC values is controversial. Thus far, research involving DWI-MRI comprises small, single-center studies; larger, multicenter studies must be performed to ascertain the power of ADC to diagnose CKD. Moreover, ADC values vary along with b values, such that a standard protocol and generic analysis model are needed for large-scale clinical application.
8.4.4 Diffusion Tensor Imaging (DTI)
Compared with DWI-MRI, the DTI technique provides more information regarding the diffusion direction of free water in tissues. This information can be provided by markers such as fractional anisotropy (FA), a measure of directional diffusivity within a range of 0–1. When FA = 0, water molecule diffusion occurs in all directions, whereas FA = 1 reflects restricted diffusion along a single orientation. In healthy kidney, both cortex and medulla are highly organized and exhibit high integrity, so water may theoretically diffuse preferentially along a fixed orientation (e.g., the direction of the tubules). Recently, several small clinical studies have shown that FA is more reflective of microstructure and spatial organization related to CKD, compared with ADC [34–36]. However, like other novel functional MRI techniques, DTI-MRI is limited in clinical application by the required sophisticated analysis model and time-intensive data acquisition processing.
8.4.5 Blood Oxygen Level-Dependent (BOLD)
Tissue hypoxia is a distinctive characteristic of CKD; in some degree, hypoxia is consistent with the morphometric and functional changes that contribute to the progression to ESRD. BOLD-MRI was initially developed for neuroimaging; in the kidney, it has attracted much attention because it can provide information regarding blood oxygenation and tissue hypoxia in vivo. Deoxyhemoglobin is a paramagnetic molecule that can act as an endogenous T2∗ contrast agent. With increasing concentration of deoxyhemoglobin, the MR signal decay becomes more rapid (shorter T2∗). Tissue parameter R2∗ (1/T2∗) has been considered to be associated with renal oxygen levels.
In healthy kidney, PO2 in the medulla is approximately 10–20 mmHg, which is much lower than that in cortex (50 mmHg). Active reabsorption by the Na/K/Cl cotransporter in the thick ascending limb is a process of high energy consumption. Thus, the renal medulla is more sensitive to hypoxia. In healthy tissue, the demarcation of cortex and medulla is sharp in MR R2∗ images, and R2∗ gradually increases from the cortex to the medulla near the renal hilus. This effect is validated by administering furosemide, an inhibitor of the Na/K/Cl cotransporter. There is no difference in R2∗ value between the left and right kidney; this value is positively related to age, but not to gender. The acquisition time of BOLD-MRI is relatively short (1–5 min) and is collected over several breath-hold intervals, which is also an advantage in clinical applications.
It has been reported that renal R2∗ (especially in the medulla) is suitable for the assessment of different pathologic conditions in kidney, including CKD [37, 38]. Recent studies in an animal model of diabetic nephropathy have shown that R2∗ values increase in the outer medulla, suggesting lower tissue oxygenation [31, 39]. However, this change only occurs in advanced stages of disease. Some studies have also shown differences in baseline T2∗ in diabetic kidneys in humans. However, other research showed that medullary BOLD signals were nonspecific and did not reflect renal function in diverse chronic renal diseases [40]. Thus, the correlation between measured T2∗ and disease stage remains controversial.
However, the sensitivity and specificity of this technique must be considered. Firstly, CKD is a sophisticated pathologic condition. Its hemodynamics, such as microvascular density and regional renal blood flow, can influence the MRI signal, as indicated by the R2∗. Secondly, with the assumption that the blood and tissue are in strict accordance with tissue oxygenation, some pathological conditions, such as fibrosis, may restrict oxygen diffusion across the microvascular lumen, and may cause heterogeneity between the vascular and renal parenchyma. Finally, kidneys are retroperitoneum organs, so intestine gas might increase R∗ values of a specific area of kidney leading to misdiagnosis as a region of hypoxia or kidney scars. Nevertheless, BOLD-MRI remains the most popular and effective technique to invasively measure tissue oxygenation in vivo.
8.4.6 Magnetic Resonance Elastography (MRE)
Based on the assumption that excessive extracellular matrix deposition in fibrotic tissues increases the stiffness of the kidneys, MRE-MRI is used to estimate tissue stiffness, as an index of fibrosis, by using translocation of mechanical shear waves. Some studies have shown the utility of this technique in patients with transplant and renal artery stenosis [41–43]. However, similar to US elastography, the results are influenced by anisotropy, vascularization, hydronephrosis, and external pressure. Therefore, advanced development of appropriate models is likely to increase the use of this novel technique.
8.4.7 Molecular Imaging
Energy dys-metabolism with a loss of ATP is an important early functional change in renal failure. 31P MR spectroscopy, which can detect the ratio of phosphomonoesters to inorganic phosphorus, is able to evaluate renal metabolism in vivo. This technique was initially used to evaluate posttransplant graft function, and is now used to assess CKD progression [44]. However, this technique remains limited by low SNR and the advanced data acquisition approach.
8.5 Nuclear-Based Technique
Radionuclide imaging, which is a functional imaging technique that uses different types of radioisotopes bound to non-metabolized molecules with known pharmacokinetics (tracers), is considered to show glomerular filtration (99mTc-DTPA and 99mTc-MDP), tubular function (99mTc-DMSA), and effective renal plasma flow (99mTc-MAG3M and 131I-OIH) [45, 46]. Both noninvasive and non-nephrotoxic, it is widely used in the clinic to evaluate total and partial divide kidney functions in CKD patients. Importantly, its disadvantage is obvious, such as relatively high radiation exposure, as well as low quantitation and spatial resolution with respect to morphological abnormities.
The development of scintigraphic methods, such as positron emission tomography (PET) and combined PET/CT imaging, enhanced the value in acquisition of reliable renal blood flow (using 82Rb or 64Cu-PTSM as a tracer) and GFR (using 68Ga-EDTA or 55Co-EDTA as a tracer) information because of good correlation with gold standard determination by radioactive microspheres [47]; moreover, this information is more valuable when fused with anatomic information. However, the clinical usage remains limited because of its high cost and complicated process.
Another attractive but limited aspect, predominately used in experimental studies, is that nuclear-based technique can directly assess molecular targets, such as membrane transporters, receptors, signal transduction, and gene expression; this makes it quantitative and suitable for in vivo assessment of tissue hypoxia, apoptosis, and endothelial dysfunction in pathological conditions. It has been reported in studies of other organs that hypoxia could by visualized by PET with 18F-fluoroazoycinarabinofuranoside or 18F-fluoromisonidazole [48]; the expression of some molecules, such as integrin, could be monitored in vivo by 18F-labeled integrin antagonists [49]. However, the usage of this approach in CKD is likely in the distant future.
8.6 Conclusion

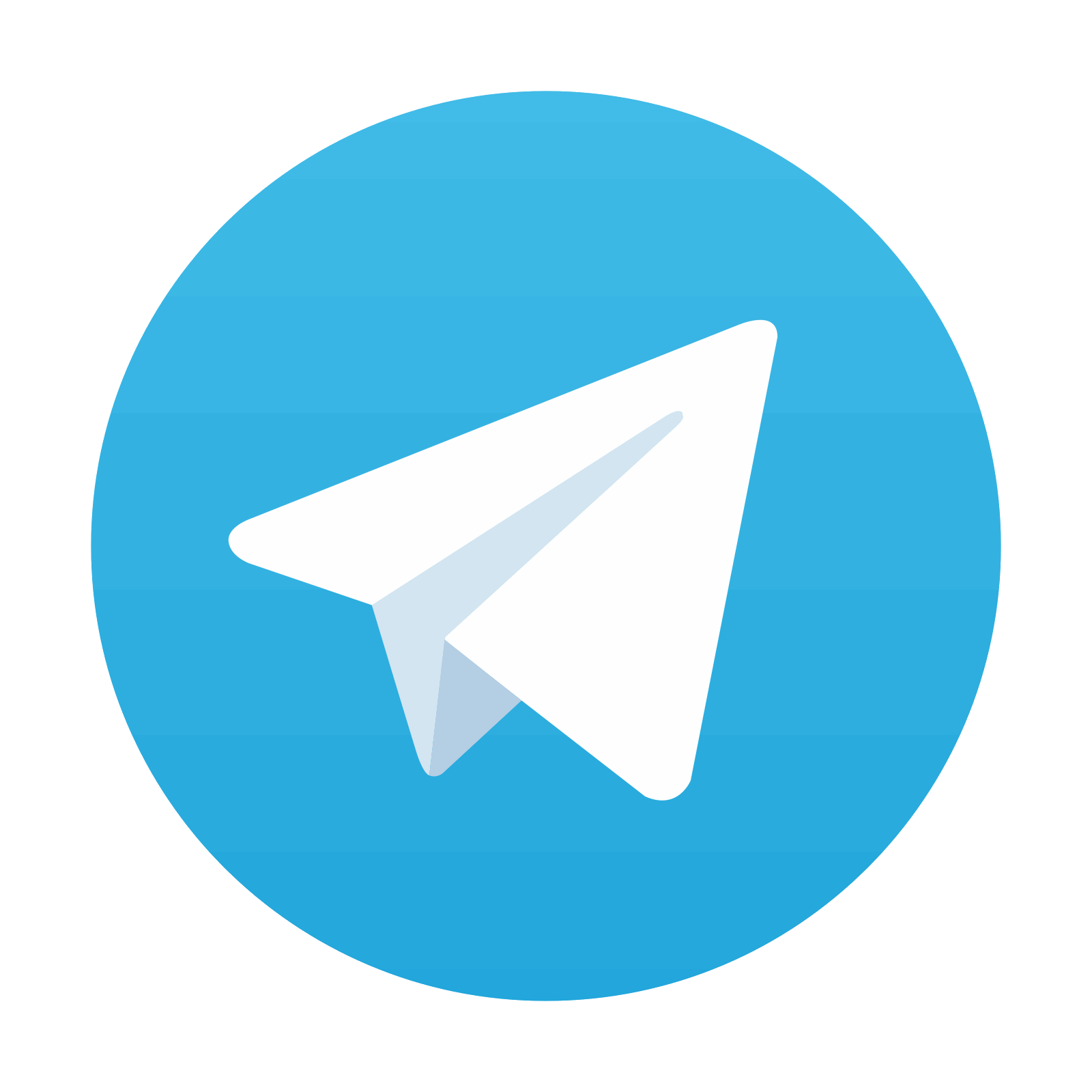
Stay updated, free articles. Join our Telegram channel

Full access? Get Clinical Tree
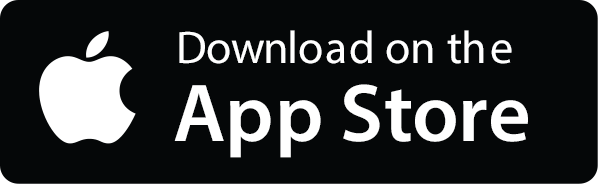
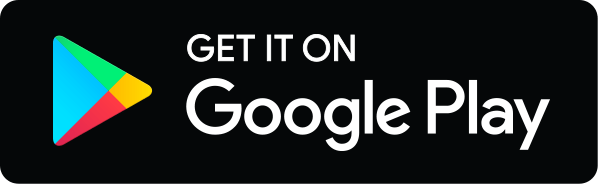