!DOCTYPE html>
14. Nutritional Management of Chronic Kidney Disease
Abstract
Since chronic kidney disease (CKD) may lead to a unique constellation of nutritional and metabolic abnormalities, a suboptimal nutritional status among the population with CKD is common, and this poses a direct risk for protein-energy wasting, disease progression, and increased mortality. Therefore, given the high incidence and prevalence of CKD and the urgent need for alternative disease management strategies, the patient-centered and cost-effective nutritional management with disease-specific dietary ranges may be a cornerstone required not only to simply adjust suboptimal nutritional status but also to help manage uremia and other complications. Moreover, it may help increase longevity and prolong the dialysis-free interval for millions of people worldwide. Nutritional interventions may be increasingly chosen as an effective management strategy for CKD.
14.1 Introduction
With an occurrence rate of approximately 10–15%, chronic kidney disease (CKD) has become a major noncommunicable disease with increasing epidemic proportion worldwide. Moreover, the increasing incidence of diabetes suggests that the frequency of CKD will continue to grow in the near future. With a gradual loss in kidney function, CKD might lead to a unique constellation of nutritional and metabolic abnormalities. Altered protein and energy homeostasis, abnormal protein catabolism, acid–base derangements, bowel flora alteration, and hormonal dysfunction usually ensue. Hence, a suboptimal nutritional status among the population with CKD is common, and this poses a direct risk for protein-energy wasting (PEW), disease progression, and increased mortality [1–3]. Therefore, nutritional intervention is a cornerstone required not only to simply adjust suboptimal nutritional status but also to help manage uremia and other complications such as electrolyte and acid–base imbalances, water and salt retention, mineral and bone disorders, and failure to thrive [1, 2, 4]. In this chapter, we mainly focus on nutritional interventions as an important management strategy for CKD.
14.2 Nutritional Assessment
As renal failure advances in patients with CKD, nitrogen-containing products accumulated from dietary and intrinsic protein catabolism might distort taste and blunt appetite. Additionally, since uremia status might affect the gut microbiome and disrupt the intestinal barrier function, gastrointestinal nutrient absorption may also become abnormal. Hence, poor nutritional status, defined as PEW and manifested as decreased body storage of protein and energy fuels, is common in patients with renal insufficiency.
Although a consensus on the importance of identifying and treating malnutrition in patients with CKD existed for decades until now, a well-established assessment system to accurately reflect the nutritional status remains unclear and not well-used in the clinical setting [1–3]. Several biochemicals, clinical, anthropometric, and nutritional parameters are associated with and frequently indicative of PEW in patients with CKD. However, the National Kidney Foundation K/DOQI Clinical Practice Guidelines for Nutrition in Chronic Renal Failure recommends that nutritional status should not be evaluated with only a single parameter alone but instead using a combination of valid and complementary parameters. According to an expert panel directed by the International Society of Renal Nutrition and Metabolism, the current procedure for the diagnosis of PEW recommends the analysis of serum chemistry (s-albumin, s-prealbumin, and s-cholesterol), body mass (body mass index, body weight variation, and percent of body fat), signs of muscle wasting over time (reduced mid-arm muscle circumference and creatinine appearance), as well as unintentional low dietary intake for at least 2 months [5–8]. Overall, the data for nutritional evaluation could be classified into four categories four categories—biochemical, anthropometric, clinical, and dietary—discussed in detail as follows:
14.2.1 Biomarkers of Nutritional Status
14.2.1.1 Serum Albumin
Serum albumin is an important nutrient manufactured by the liver that helps support muscle growth, repair damaged tissue, and defense against pathogen infection. Its concentration is influenced by many factors, such as rates of synthesis and breakdown, volume of distribution, exchange between intra- and extravascular spaces, as well as losses and wasting from the body. In patients with CKD, hypoalbuminemia can be the result of factors such as fluid overload, proteinuria, and losses to the dialysate. Counter-regulatory mechanisms may also influence the serum albumin concentration. In the short term, protein deficiency might reduce the hepatic albumin-synthesizing activity; however, in the long term, compensation might occur through a decreased albumin breakdown and a shift of albumin from the extravascular to the intravascular space. Furthermore, because albumin has an extraordinarily long circulatory half-life of about 20 days and is present in large quantities, the impact of reduced dietary protein intake on serum albumin levels is limited. Hence, even in extreme cases of malnutrition, such as marasmus and anorexia nervosa, serum albumin concentrations could remain normal or only slightly reduced. Numerous studies have shown that serum albumin levels in CKD patients and dialysis patients are usually low. However, it is important to consider that patients with CKD commonly have comorbid conditions, such as insufficient food intake, fluid overload, and chronic inflammation, all of which are known to affect serum albumin levels. Hypoalbuminemia in patients with CKD seems to be associated with a chronic inflammation rather than with malnutrition. Despite the wide use of albumin as a nutritional status biomarker in CKD in many researches, serum albumin levels might actually be a marker of disease severity rather than nutrition and may be a poor parameter of nutritional status in patients with CKD [9, 10].
14.2.1.2 Prealbumin
Prealbumin, which is also primarily synthesized by the liver, is a 55-kD homotetrameric protein in blood that plays a role in carrying thyroxine and retinol throughout the body. Prealbumin has a half-life in plasma of ∼2 days, much shorter than that of albumin. It is catabolized partly in the kidneys, and consequently any renal dysfunction causes an increase in its serum levels. Generally, prealbumin correlates with the level of nutrition support, increasing with sufficient dietary intake and decreasing when dietary intake is declining. In patients with CKD, the levels of prealbumin show a linear relationship to the degree of protein-energy malnutrition in patients with CKD, which are restored by refeeding. However, as with serum albumin, prealbumin decline sensitively in response not only to inadequate protein intake but also to acute or chronic inflammation (i.e., “acute-phase reactant”), limiting its specificity as a marker of nutritional status. Therefore, although prealbumin is more sensitive in assessing changes of nutritional status than serum albumin, however, since it could be influenced by many non-nutritional factors, serum prealbumin is not considered to be a valid indicator of nutritional status in patients with CKD.
14.2.1.3 Creatinine Height Index
Creatinine is a waste product that comes from continuous creatine phosphate breakdown in muscle. Since almost all creatinine is filtered from the blood into the urine by kidneys, serum creatinine level is usually a good indicator of kidney functions. Under normal renal function, creatinine is typically produced by the body at a relatively constant rate at a level proportional to total muscle mass. Urinary creatinine excretion rate is generally related to muscle mass [11]. Normalized for height, the measurement of 24-h creatinine excretion is an indicator of muscle mass, particularly in young males. However, it is dependent on complete 24-h urine collections and urinary excretion; low excretions of urinary creatinine may result in an inappropriate diagnosis of malnutrition. Therefore, the creatinine height index might be an inaccurate indicator of malnutrition in patients with CKD.
14.2.1.4 C-Reactive Protein (CRP)
C-reactive protein is an inflammation marker and not a direct nutritional marker. However, it also plays an important role in the overall assessment of nutritional status in patients with CKD because it is an acute-phase reactant that is inversely correlated with the concentrations of visceral proteins. In clinical practice, one should remember that very low levels of visceral proteins, for instance, serum albumin, are often due to the presence of inflammation rather than the low protein intake or protein depletion [9]. Thus, both in the clinical and research settings, it is recommended to check CRP levels in conjunction with other nutritional markers and when deciding possible nutritional intervention as well as interventions against factors causing increased CRP levels.
14.2.1.5 Cholesterol
Cholesterol is a lipoprotein that functions as a precursor for the synthesis of steroid hormones, bile acids, and vitamin D. Serum cholesterol and several other blood lipids and lipoproteins such as total cholesterol, triglycerides, low-density lipoprotein [LDL] cholesterol, high-density lipoprotein [HDL] cholesterol, and non-HDL cholesterol are indicators of patients’ nutritional status. Indeed, s-cholesterol has been proposed as an assessment criterion for malnutrition and PEW. Contrary to the general population, a high s-cholesterol level in the CKD and dialysis population is associated with improved survival [1, 2]. However, this association seems to only be true in patients with inflammation and/or malnourishment, which suggest that low levels of s-cholesterol may be a surrogate marker of inflammation and/or malnutrition.
14.2.2 Clinical and Anthropometric Markers of Nutritional Status
14.2.2.1 Subjective Global Assessment (SGA)
Subjective Global Assessment (SGA) is a simple and reliable nutritional assessment tool. It is composed of five components of a medical history (recent dietary intake, gastrointestinal symptoms, weight change, functional capacity, disease, and its relationship to nutritional requirements) and three components of a physical examination (signs of fat and muscle wasting, nutrition-associated alternations in fluid balance) to predict nutrition-associated complications. Since SGA evaluations could effectively demonstrate the changes of nutritional status occurring throughout the course of the disease, it was found to be highly predictive of malnutrition and outcome in those who are nutritionally compromised in any stage of CKD or in danger of becoming malnourished [12]. Using the SGA, many studies reported that patients with CKD, whether or not on dialysis, met only 50–70% of their energy needs and only about 50% of their protein needs. The SGA has been used as a surrogate for PEW in many studies [13–15]. The Academy recommends that SGA be performed in patients with CKD at the initial visit and quarterly to determine the patients’ nutrition status. These numbers and SGA scores improve if the dietitians followed their patients monthly.
Although SGA has recently been recommended by the National Kidney Foundation (NKF) Kidney Disease/Dialysis Outcomes and Quality Initiative (K/DOQI) for assessing the nutritional status in the adult dialysis population, it is a subjective score that may be biased by inter- and intrapersonal differences. Therefore, a longitudinal research study conducted by the well-trained investigator following patients over time might be necessary.
14.2.2.2 Malnutrition Inflammation Score (MIS)
Stemming from the original SGA, a new comprehensive nutritional assessment tool called the MIS which involves seven components from the SGA questionnaire (weight change, dietary intake, gastrointestinal symptoms, functional capacity, comorbidity, subcutaneous fat, and signs of muscle wasting) and the three additional biochemical parameters influenced by inflammation status (body mass index, serum albumin, and total iron-binding capacity (TIBC)) was proposed by Kalantar-Zadeh et al. [16]. In total, MIS system comprises ten parameters, each including four degrees of severity (where 0 = normal and 3 = severely abnormal) and summing each component to produce a final score (0 = normal nutritional status and 30 = severely malnourished). Numerous studies have demonstrated that higher MIS scores were associated with worse nutritional status, poorer health-related quality of life, as well as higher hospitalization and mortality rates in maintenance hemodialysis patients [17].
14.2.2.3 Other Nutritional Scoring Systems
In addition to the SGA and MIS, alternative scoring systems have also been developed to assess nutritional status. Among those, Mini Nutritional Assessment (MNA) has been originally developed as nutritional assessment tools for the geriatric population [18]. It is made up of a screening section (six questions), known as Mini Nutritional Assessment-Short Form (MNA-SF), which can be further complemented by 11 questions in order to gain a malnutrition indicator score scale (full-MNA form). In addition, these tools have also been used in CKD and dialysis patients; however, the usefulness of the MNA-SF remains questionable.
14.2.2.4 Body Mass Index (BMI)
Body mass index (BMI), which is calculated as body weight in kilograms divided by the square of height in meters, is an indicator of body fat. According to the World Health Organization (WHO) guidelines, the healthy range for BMI is between 18.5 and 24.9 kg/m2 for adults. Obesity is defined as a BMI ≥30.0 kg/m2, overweight as a BMI of ≥25.0 kg/m2, and underweight as a BMI <18.5 kg/m2. In patients with CKD, the BMI may not reflect the real nutritional status, as a gross imbalance in fluid status in these patients may cloud the results. Furthermore, loss of muscle mass is characteristic of PEW; however, a relatively well-preserved fat mass usually remains, resulting in small changes in BMI that can be disguised by imbalances in fluid homeostasis. Finally, overweight patients with end-stage renal disease (ESRD) may also suffer from PEW. For these reasons, BMI alone does not accurately reflect the nutritional or PEW status in patients with CKD.
14.2.2.5 Hand Grip Strength (HGS)
Muscle strength in patients with CKD is often evaluated by muscle dynamometry such as hand grip strength (HGS) [19], which associates with lean body mass as assessed by anthropometry, dual-energy X-ray absorptiometry (DEXA, see below), and creatinine kinetics and with nutritional status as assessed by SGA score. In addition, the combined assessment of body composition (lean body mass) and muscle function has become more prevalent as a composite marker of nutritional status. Indeed, a dynamometric HGS measurement standardized to age and gender has emerged as an easily performed bedside test that is considered to be a reliable and available marker of both nutritional status and future mortality risk.
14.2.3 Dietary Intake Information
There are many methods to estimate dietary intake; however, the accuracy of document is frequently fluctuating. In general, during a nutrition interview, the clinical dietitian may ask what the individual ate during the previous 24 h, beginning with the last item eaten prior to the interview. Alternatively, practitioners can also train individuals on completing a food record typically obtained for 3–7 days. The documentation should include portion sizes and how the food was prepared. However, documenting portion sizes is usually difficult, and requesting that every food be measured or weighed is usually very difficult to implement and time-consuming. Therefore, nutrition interviews are not commonly used in practice.
14.2.4 Other Methods to Assess Nutritional Status
14.2.4.1 Bioelectrical Impedance and Conductance
Bioelectrical impedance analysis (BIA) has been proposed as a noninvasive and simple technique to measure body hydration status of patients, especially with regard to the determination of “dry” body weight in hemodialysis patients [20, 21]. BIA has also been suggested as a valuable tool in subjects undergoing peritoneal dialysis. BIA assumes that the human body may be viewed as a number of parallel connected resistors. By connecting electrodes to various body parts (typically the arms and legs) with a conductive gel, an electric current passes through the body at various frequencies and the conductance is measured. The current standard BIA models used in CKD view the body as five interconnecting cylinders: two each for the arms and legs and one for the trunk, instead of the more common previous assumption that the whole body can be visualized as a single cylinder. BIA models are prone to errors of simplification which depend on body size, shape, and regional fluid accumulation.
14.2.4.2 Dual-Energy X-Ray Absorptiometry
Dual energy X-ray absorptiometry (DEXA), which was primarily used for assessing bone mineral density, has become another more reliable tool to estimate body composition [20]. When DEXA passes low-energy X-rays through the body, a low-resolution image could be generated to measure fat distribution throughout the body [22]. However, although DEXA could accurately measure fat mass with high-precision, low X-ray exposure, and short scanning time, it is hardly feasible in routine clinical practice due to its high cost and its inaccuracy in severely overhydrated patients. Furthermore, the amount of radiation used during repeated DEXA scans, although small, may be of concern in patients.
14.2.4.3 Magnetic Resonance Imaging (MRI)
Magnetic resonance imaging is a noninvasive medical imaging technique measuring nuclear relaxation times from nuclei of atoms with magnetic moments that are aligned within a powerful magnetic field. Magnetic resonance imaging can be used to quantify components at the tissue-system levels of body composition, including skeletal muscle, adipose tissue, visceral organs, and the brain. Clinical systems are based on hydrogen, although it is possible to create images and spectrographs from phosphorus, sodium, and carbon. The collected data are transformed into high-resolution images, which allow the quantification of whole-body or regional-body compositions. However, to our knowledge, no high-quality clinical studies have assessed imaging methods in relation to outcome.
14.3 Nutritional Management
14.3.1 Energy Intake
Designing a diet for the patients with CKD to receive enough calories is of most importance. However, in clinical practice, it is really a challenge to estimate accurate energy requirements in patients with CKD, since the daily energy requirements are influenced by many variable factors, such as resting energy expenditure, thermic effect of meals, physical activity level, and so on. Many previous studies have shown that the energy requirements of patients with CKD were similar to those of normal adults. Therefore, in CKD stages 1–3, the Kidney Disease Outcomes Quality Initiative (KDOQI) recommends that energy intake levels support a balanced diet and maintain desirable body weight but does not recommend specific energy intake amounts. However, in CKD stages 4 through 5 (GFR <30 mL/min/1.73 m2), specific energy intake amounts are recommended: 35 kcal/kg/day for those younger than 60 years and 30–35 kcal/kg/day for those older than 60 years. If the patients were overweight and obese, calories should be restricted because calories intake in excess of requirements might cause obesity, and obesity might in turn engender insulin resistance and impaired glucose disposal [1, 2, 4].
14.3.2 Protein Intake
Whether the quantity or quality of ingested protein is a risk factor for chronic kidney disease progression has been debated for nearly a century. Now, there are mounting evidences that the western-type diet, which contains exceeding 1.5 g per kilogram of ideal body weight per day, may cause glomerular hyperfiltration and proinflammatory gene expression, which are known risk factors for CKD progression. Experimental evidence in animal models has also shown that a high-protein diet dilates the glomerular afferent arterioles and increases glomerular filtration, whereas low protein intake constricts the afferent arterioles and lowers intraglomerular pressure. The current evidence has suggested that reducing protein intake could decrease proteinuria as efficiently as angiotensin-converting enzyme inhibitors, mainly because that a low-protein diet has a preglomerular effect that may enhance the postglomerular effect of angiotensin pathway modulators that dilate the efferent arterioles and consequently lower the intraglomerular pressure [1, 2, 4].
Besides, limiting protein intake also results in an instant reduction in urea generation. After protein breakdown, individual amino acids are deaminated by the removal of an α-amino group, leaving a carbon skeleton of ketoacids, which can be recycled to form other amino acids and proteins or can be used for energy generation through the tricarboxylic acid cycle, while urea is generated through the urea cycle. A persistently high blood urea level, termed azotemia, which is a commonly used marker for uremia, may enhance protein carbamylation and generate reactive oxygen species, leading to oxidative stress, inflammation, endothelial dysfunction, and ultimately, cardiovascular disease. Reducing protein intake could effectively decrease the production of wasted products and uremic toxins which in turn diminishes the uremic symptoms. Metabolic consequences of low protein diet have also been extensively researched in recent years: reduced oxidative stress, improved insulin sensitivity, better control of metabolic bone disorders in response to a reduced phosphate load, and improved anemia management.
For healthy persons, the recommended dietary allowance for protein is 0.8 g per kilogram per day, whereas the estimated average requirement for adults with CKD who are otherwise healthy is 0.66 g per kilogram per day. Hence, of the various ranges of low protein intakes, 0.6–0.8 g per kilogram of body weight per day is the most frequently recommended target for adults with moderate-to-advanced kidney disease (estimated GFR <45 mL/min/1.73 m2) and for the management of substantial proteinuria (urinary protein excretion, >0.3 g per day), especially if half of the protein is of “high biologic value” (e.g., dairy products). However, the so-called very-low-protein diet (<0.6 g of protein per kilogram per day), supplemented with essential amino acids or their ketoacids, is also used. People at increased risk for kidney disease, such as those who have undergone nephrectomy for kidney donation or for cancer treatment or who have diabetes mellitus, hypertension, or polycystic kidneys, may benefit from a modest protein intake (<1 g per kilogram per day) in order to maintain a moderately low intraglomerular pressure [1, 2, 4, 23, 24].
As mentioned previously, protein-energy wasting (PEW) is commonly seen in patients with chronic kidney disease (CKD); the safety and feasibility of long-term dietary modification are among the main concerns. From a basic point of view, the direct relationship between low protein intake and muscle wasting should be discovered. Unfortunately, this approach is not clinically relevant: muscle wasting in chronic kidney diseases seems to be caused by the imbalance toward a catabolic state (more protein degradation than synthesis) and is further deteriorated by the lack of physical activity. Additionally, metabolic acidosis closely related to inflammation and insulin resistance is also an important reason. Restrained protein intake has been proven to improve all these catabolic conditions. Therefore, the safety of and adherence to a low-protein diet might be improved by providing adequate energy (30–35 kcal per kilogram per day), ongoing nutritional education, and surveillance.
14.3.3 Sodium Intake
Patients with hypertension and CKD are usually salt-sensitive. The available evidence, detected as an increase in blood pressure of more than 10% when a low salt diet is switched to a high salt intake, demonstrated that a high-sodium diet (>4 g of sodium per day) in CKD has an influence on hypertension, cardiovascular risk factors, and outcomes. In patients with established CKD, dietary sodium restriction is invariably recommended to control fluid retention and hypertension and to improve the cardiovascular risk profile. However, the association between salt intake and renal outcome in subjects with preserved kidney function remains less well investigated and confounding. Several studies have demonstrated that a reduced sodium intake could enhance the effects of a low-protein diet and angiotensin-modulation therapy in decreasing intraglomerular pressure and might also decrease proteinuria and slow the progression of kidney disease. However, some other studies reported that strict sodium restriction might also activate the renin-angiotensin-aldosterone system, sympathetic nervous system, and insulin resistance.
As ingested sodium is primarily excreted via the kidneys, a 24-h urinary sodium excretion is a good indicator of sodium intake. Observational studies using urinary sodium excretion as a surrogate for sodium chloride intake have yielded inconsistent data, with some studies showing no association between dietary sodium intake and renal disease progression and others showing a positive association. A longitudinal study published in 2016, which involved serial 24-h urine collections from 3939 patients with CKD, suggested that the highest quartile of urinary sodium excretion (≥4.5 g per day), as compared with the lowest quartile (<2.7 g per day), was associated with a 45% higher mortality and a 54% higher risk of disease progression. Incrementally worse cardiovascular outcomes were observed when dietary sodium intake exceeded 4 g per day. Observations in the general population suggest a J-shaped association; dietary sodium intake that is higher than 5 g per day and intake that is lower than 3 g per day are each associated with an increased risk of cardiovascular disease and death. Although a daily dietary allowance of less than 2.3 g of sodium (<100 mmol) is often recommended for patients with cardiovascular disease, there is no evidence that patients with kidney disease will benefit from this sodium restriction. Therefore, a daily dietary sodium intake of less than 4 g (<174 mmol) is recommended for the overall management of CKD and its associated risks, with a sodium intake of less than 3 g (<131 mmol) for the specific management of symptomatic fluid retention or proteinuria. Evidence supporting a sodium intake of less than 1.5 g per day (<87 mmol per day) for patients with renal insufficiency is lacking, given the risk of hyponatremia and adverse outcomes. Once sodium excretion is excessive and blood pressure elevates, the nutrition consultation and repeating 24-h urine measurements of sodium are recommend to make dietary planning more easily [1, 2, 4, 23–25].
Whereas adequate fluid intake may mitigate the risk of kidney disease, patients with renal insufficiency generally have isosthenuria. This is the basis for the recommendation that patients with stage 3 CKD limit fluid intake to less than 1.5 L per day in order to avoid hyponatremia; adjustment of that limit for a hot climate and other conditions associated with high insensible fluid losses is imperative. Adjunctive therapy with loop diuretics is often prescribed, particularly for patients who tend to have symptomatic fluid retention or hyponatremia, given the association of such conditions with poor outcomes in CKD. It should also be noted that diuretics alone might fail because an unrestricted salt intake will overcome the effectiveness of diuretic.
14.3.4 Potassium Intake
Substantial evidence has supported that potassium-rich foods could reduce the possibility of developing chronic diseases, such as hypertension, diabetes, and coronary heart disease. Thus, given the well-established association of higher dietary potassium with lower sodium intake and lower incidences of hypertension, stroke, and kidney disease, a relatively high daily intake of potassium of 4.7 g (120 mmol) was recommended in the general population by guidelines from the Institute of Medicine. However, the causal relationship between dietary potassium and hypertension in patients with CKD was less studied in the previous researches.
Accompanied by renal insufficiency, the kidneys’ ability to excrete excess potassium might be impaired. Besides, the impairment in the action of protective hormones (e.g., aldosterone) or use of ACEIs, ARBs, or nonsteroidal anti-inflammatory drugs may also result in the impaired potassium excretion. Therefore, a careful search for other causes of hyperkalemia should be undertaken before restricting dietary potassium. Particularly, drugs that reduce potassium excretion should be eliminated, acidosis should be corrected, and constipation should be relieved. Undoubtedly, more studies are further needed to investigate the advantage and dangers of increasing (or limiting) dietary potassium in patients with CKD.
A higher dietary potassium intake may be associated with a higher risk of kidney disease progression. Among patients with very advanced CKD, the highest quartile of dietary potassium intake, as compared with the lowest quartile, is associated with an increase in the risk of death by a factor of 2.4; the association is independent of the plasma potassium level and other nutritional measures. Therefore, the National Kidney Foundation’s expert panel recommended potassium restriction for individuals with advanced CKD (e.g., stage 4 CKD and an estimated GFR <30 mL/min/1.73 m2). In other epidemiologic studies, both moderately low (<4.0 mmol per liter) and high plasma potassium levels (>5.5 mmol per liter) are associated with more rapid kidney disease progression. Dietary potassium restriction is often recommended in patients with hyperkalemia, especially those with more advanced stages of kidney disease. However, excessive dietary restrictions can expose patients to less heart-healthy and more atherogenic diets and worsen constipation, which may actually result in higher gut potassium absorption. Despite the higher risk of hyperkalemia with the progression of kidney disease, few studies have examined the effects of dietary potassium restriction or methods of extracting potassium during food preparation and cooking. It is not clear whether potassium-binding agents can allow the liberalization of dietary potassium intake with the inclusion of healthier potassium-rich foods. In patients with a tendency toward hyperkalemia (>5.5 mmol of potassium per liter), a dietary potassium intake of less than 3 g per day (<77 mmol per day) is recommended, with the stipulation that the balanced intake of fresh fruits and vegetables with high fiber should not be compromised [1, 2, 4, 23, 24, 26, 27].
14.3.5 Phosphorus Intake
Numerous studies have determined positive phosphate balance and hyperphosphatemia to be the risk factors for vascular calcification, cardiovascular mortality, and left ventricular hypertrophym in chronic kidney disease. Daily dietary phosphorus intake is approximately 1000–1200 mg, of which approximately 950 mg is absorbed. Phosphorus could be removed by two systems, the gastrointestinal tract (150 mg/day) and the urine (800 mg/day). However, as kidney function declines, renal phosphorus excretion might be progressively impaired. Decreased glomerular filtration of phosphorus is initially compensated by decreased tubular reabsorption regulated by parathyroid hormone and fibroblast growth factor 23 (FGF-23). Elevated parathyroid hormone and FGF-23 levels can cause renal bone disease, left ventricular hypertrophy, vascular calcification, and accelerated progression of kidney disease from vascular and tubulointerstitial injury, highlighting the importance of dietary phosphorus management, even in patients without apparent hyperphosphatemia. There is a close relationship between protein and phosphorus intake: 1 g protein brings 13–15 mg phosphate, of which 30–70% is absorbed through the intestinal lumen. High-protein diet may aggravate uremic symptoms and hyperphosphatemia; however, although a low-protein diet also decreases phosphorus intake, the quantity and bioavailability of phosphorus differ according to the type of protein. For example, the phosphorus-to-protein ratios of egg whites and egg yolks (which have 3.6 and 2.7 g of protein per egg, respectively) are 1–2 mg per gram and 20–30 mg per gram, respectively. The gastrointestinal absorption of phosphorus, mostly in the form of phytates, is lower from plants (along with fibers) than that from meat (30–50% vs. 50–70%). Since food additives include readily absorbable inorganic phosphorus, ingestion of processed foods results in an even higher phosphorus burden. Restricting dietary phosphorus intake to less than 800 mg per day (26 mmol per day) is recommended for patients with moderate-to-advanced kidney disease, and the consumption of processed foods with a high phosphorus-to-protein ratio should be minimized. However, in patients with stage 5 CKD who receive dialysis therapy or who are at increased risk for PEW, excessively stringent restriction of protein intake to control hyperphosphatemia may be associated with poor outcomes. Thus, an individualized dietary approach that incorporates ample use of phosphorus binders is optimal [1, 2, 4, 23, 24, 28].
14.3.6 Calcium and Vitamin D
Vitamin D insufficiency and deficiency is widely prevalent in the patients with CKD, which is further exacerbated by the reduced ability to convert 25-(OH) vitamin D into the active form, 1,25 dihydroxy-vitamin D. The associated decline in 1,25-dihydroxy vitamin D may further diminish gastrointestinal absorption of calcium; however, passive diffusion of ionized calcium continues and may lead to a positive calcium balance, which is aggravated by diminished urinary calcium excretion due to secondary hyperparathyroidism. Increased calcium release from bone in hyperactive renal bone disease (increased bone resorption because of secondary hyperparathyroidism) enhances the positive calcium balance and may worsen vascular calcification. Gut calcium absorption varies because of differences in dissociation and bioavailability from one type of elemental calcium to another; for instance, calcium citrate is more readily absorbable than calcium acetate. Two studies suggested that an intake of 800–1000 mg of elemental calcium per day (20–25 mmol per day) can result in a stable calcium balance in people with stage 3 or 4 CKD. Hence, whereas the suggested calcium intake for persons without kidney disease is 1000–1300 mg per day (25–32 mmol per day), 800–1000 mg of elemental calcium from all sources per day should suffice in patients with moderate-to-advanced CKD [1, 2, 4, 23].
Native vitamin D supplementation (cholecalciferol or ergocalciferol) may be offered to patients with CKD and low levels of circulating vitamin D. In some studies, vitamin D analogs have been associated with decreased proteinuria in addition to the healing of renal osteodystrophy. Notwithstanding inconsistent data on the requirement for and the effect of vitamin D in certain subpopulations of patients with CKD, including black Americans, who have lower total vitamin D levels and higher parathyroid hormone levels than those in white Americans, hydroxylated vitamin D agents may be needed in addition to native vitamin D to control progressive secondary hyperparathyroidism.
14.3.7 Vegetarian Diet, Fiber, and the Microbiome
Plant-based diets, despite containing low amounts of protein, are also rich in potassium and phosphorus, and therefore vegetarianism is believed to be unsuitable for CKD patients. However, numerous clinical studies have recently demonstrated that such a plant-based diet could be beneficial for the patients when they learn how to use it wisely. Plant-based foods are recommended as part of many strategies for the prevention and management of kidney disease because these foods contain smaller amounts of saturated fatty acids, protein, and absorbable phosphorus than meat; they also generate less acid and are rich in fibers, polyunsaturated and monounsaturated fatty acids, magnesium, potassium, and iron. Besides, according to several studies, a plant-based diet was found to delay the progression of CKD, help to control high blood pressure, and decrease proteinuria, while others indicated that high plant protein intake is likely to accelerate CKD progression when compared to animal proteins. Therefore, the National Kidney Foundation recommends vegetarianism, or part-time vegetarian diet as being beneficial to CKD patients.
Besides, constipation can lead to higher retention of uremic toxins and hyperkalemia, whereas loosening stools may enhance fluid loss and removal of nitrogenous products. The protein in a vegetarian diet is less fermentable, and has high fiber content, increasing peristalsis, and the number of bowel movements, and is associated with less uremic toxin production, exposure, and absorption.
Uremia itself, as well as dietary restrictions and pharmacotherapy, including antibiotics, may alter the gut microbiome; this change may affect the symptoms and progression of kidney disease. Gut dysbiosis, which is manifested by qualitative and quantitative changes in host microbiome profile and disruption of gut barrier function, was commonly seen in patients with CKD. Endotoxin derived from gut bacteria can incite a powerful chronic inflammation in the host organism. Furthermore, disruption of gut barrier function in CKD may allow translocation of endotoxin and bacterial metabolites to the systemic circulation, which contributes to uremic toxicity, inflammation, progression of CKD, and associated cardiovascular disease. Therefore, targeted microbiome modulation through nutritional interventions, such as consumption of probiotics, may help to control the production, degradation, and absorption of certain uremic toxins that are fermentation by-products of gut microbial activities, including indoxyl sulfate, p-cresol, and trimethylamine [1, 2, 4, 23, 29]. For example, in a study involving 40 patients with moderate-to-advanced CKD, a lower ratio of dietary fiber to protein was associated with higher blood levels of indoxyl sulfate and p-cresol. Nutritional and pharmacologic interventions, including the use of absorbent ingestible agents and high-fiber or vegetarian diets, are being tested as a means of reducing gut absorption of uremic toxins to control uremic symptoms and slow disease progression.
14.3.8 Carbohydrate and Fat Intake
National dietary recommendations have promoted high-carbohydrate, low-fat diets to reduce cardiovascular disease risk. These recommendations were based on observational studies in which low fat intake was associated with a low risk for cardiovascular disease, presumably by lowering LDL cholesterol levels. Unrefined carbohydrates account for half the usual daily energy intake, and the proportion may be even higher in a low-protein diet. In patients with kidney disease, carbohydrates should be complexed with high fiber content (e.g., whole-wheat breads, multigrain cereal, oatmeal, and mixed fruits and vegetables) to help reduce dietary phosphorus and protein as well as urea and creatinine generation. Such a diet may promote a more favorable microbiome with less constipation.
Reducing fat as part of a low-calorie diet is a practical way to reduce energy intake. However, clinical trials of diet therapy to reduce lipids and slow progression of CKD have not been conducted. Dietary fat recommendations for obese patients with CKD should be in accordance with the National Cholesterol Education Program/Adult Treatment Panel III (NCEP/ATPIII) guidelines (2001) developed for cardiovascular risk reduction. In CKD stages 1 through 4, the KDOQI recommends that 25–35% of the total energy intake comes from fat, with 10% of the total from saturated fat. The recommended cholesterol intake is 200 mg/day. The objective of these guidelines is to control blood lipid levels, minimizing elevated blood glucose and triglyceride levels. Because diets for patients with CKD are sometimes mildly restricted in protein, it may be difficult to provide sufficient energy without resorting to a large intake of high-glycemic index carbohydrates that may increase triglyceride production. Another challenge when addressing fat intake is maintaining recommended macronutrient balances when lowering saturated fat in the diet. When saturated fat is reduced in the diet, it can be replaced with unsaturated fat, protein, or carbohydrates. The optimal means of replacement of saturated fats is not known. Data from dietary intervention trials suggest that a diet low in saturated fat that uses either protein or unsaturated fats to replace carbohydrates can have favorable effects on lipids. Unsaturated fat is the preferred lipid in the diet. Replacement of butter with flaxseed, canola, or olive oil, all of which are rich in unsaturated fatty acids, may be worthwhile. For example, a recent study suggested that dietary unsaturated fatty acid supplementation in patients with diabetes and hypertriglyceridemia may reduce albuminuria and preserve renal function. There is currently no evidence that low-fat diets, recommended by some guidelines, improve kidney disease outcomes. In a low-protein diet, fat and carbohydrates should together account for more than 90% of the daily energy intake requirement of 30–35 kcal per kilogram to avoid PEW. Obviously, in patients with diabetic kidney disease, proper glycemic control should be maintained, but adequate energy intake is needed to mitigate the risk of PEW and hypoglycemia, which increases with worsening kidney function [1, 2, 4, 23].
14.3.9 Dietary Management of Acidosis in Chronic Kidney Disease
Daily acid production results from bicarbonate losses in the gut (20–30 mmol of bicarbonate per day), breakdown of amino and nucleic acids from proteins (20–30 mmol per day), and oxidation of carbohydrates and fats to lactic acid and ketoacids (10–20 mmol per day). The kidney plays an important role in regulating of the acid–base balance. The kidneys regenerate the bicarbonate used for buffering by the excretion of both net acid and acid buffers, including phosphate, and by ammoniagenesis through the deamination of glutamine in the proximal tubule and its synthetization to ammonium in the collecting ducts, with subsequent urinary excretion. Hence, chronic kidney disease and reduced glomerular filtration rate may contribute to the development of chronic metabolic acidosis. Kidney disorders, including renal tubular defects, are often associated with chronic metabolic acidosis. Metabolic acidosis is a relatively common complication in patients with renal failure, particularly in those with GFR falls below 30 mL/min/1.73 m2. Also, a large amount of evidence identifies acidosis not only as a consequence of, but as a contributor to, kidney disease progression.
Diet plays an important role in acid–base balance. The modern high-protein diets may yield about 1 mmol/kg body weight/day of net endogenous H+ production, while the fruits and vegetables may generate base from the metabolism of organic anions such as citrate and malate. The 24-h urinary excretion of ammonium and titratable acid minus bicarbonate could be used as an indicator of total net acid excretion (NAE). A well-controlled clinical trial in healthy adults consuming various diets has revealed that pH value in 24-h urine was closely related to the total renal NAE. Moreover, the total urinary NAE can be reasonably estimated from dietary intake, intestinal absorption, and the metabolism of most important inorganic anions and cations in urine. On the basis of these factors, the potential renal acid load (PRAL) can be calculated directly from dietary intakes. While protein-rich foods such as meat, fish, and cheese are the food groups with the highest acid loads, fruits, vegetables, salads, and fruit juices have a high alkalizing potential. An increase in the dietary acid load may be associated with glomerular hyperfiltration. Metabolic acidosis is associated with more rapid kidney disease progression and an increase in the overall risk of death. Hyperparathyroidism, along with chronic buffering of acid by bone, leads to progressive loss of bone minerals and worsening renal osteodystrophy. Hence, reduced protein intake with a greater proportion of diet from plant-based foods to correct acidosis improves bone mineralization and may slow protein breakdown and disease progression. Adjunctive alkali therapy can also be considered to mitigate acidosis in patients with CKD [1, 2, 4, 30].
14.3.10 Trace Elements and Vitamins
PEW has the potential to impact not only macronutrient metabolism but also vitamin and trace element status in patients with CKD. Inadequate food intake may result in an insufficient ingestion of antioxidant vitamins, including vitamins C and E and carotenoids; in addition, patients with advanced renal disease often become deficient in folate, vitamin K, and calcitriol. A micronutrient imbalance in patients with kidney disease may contribute to a higher burden of oxidative stress, inflammation, and cardiovascular disease. Among the trace elements, iron deficiency is most problematic given the high frequency of gastrointestinal blood loss in patients with CKD. Deficiencies of zinc, copper, and selenium may occur, whereas aluminum and magnesium levels may increase. A recent study showed that 800 μg of folic acid per day, when added to enalapril, led to slower disease progression than that with enalapril alone. Experimental models of CKD suggest that vitamin K supplementation may blunt the development of vascular calcification. Daily intake of other vitamins and trace elements at conventional doses is often recommended both for persons at high risk for kidney disease and for those with established renal insufficiency [1, 2, 4].
14.3.11 Practice Strategies
Dietary protein, energy, and micronutrient intakes should be assessed regularly. In addition, 24-h urine collection should be performed to estimate the dietary intakes of protein (based on urinary urea nitrogen), sodium, and potassium; to measure creatinine clearance and proteinuria; and to evaluate adherence to dietary recommendations, with suggestions for improving adherence if necessary. Excessive restrictions may be harmful and should be avoided [4].
14.4 Conclusions
Given the high incidence and prevalence of CKD and the urgent need for alternative disease management strategies, patient-centered and cost-effective nutritional interventions with disease-specific dietary ranges help delay progression of CKD, prolong the dialysis-free interval, improve quality of life, and increase longevity for millions of people worldwide. Nutritional management for renal failure is structured to achieve a lower protein, phosphate, and sodium intake, while supplying with enough energy. In general, the purpose of nutritional management is to improve signs, symptoms, and complications of renal insufficiency, delay the progression of disease, and preserve good nutritional status.
Key Messages
Nutritional status in patients with CKD should not be evaluated with only a single parameter but with the combined use of valid and complementary parameters.
Nephrologists, nutritionists, and other interested physicians have a common principle of discussing the underlying research and translation of best practices for the nutritional management and prevention of renal disease.
The purpose of nutritional management for CKD is to maintain good nutritional status, slow progression, and reduce complications.
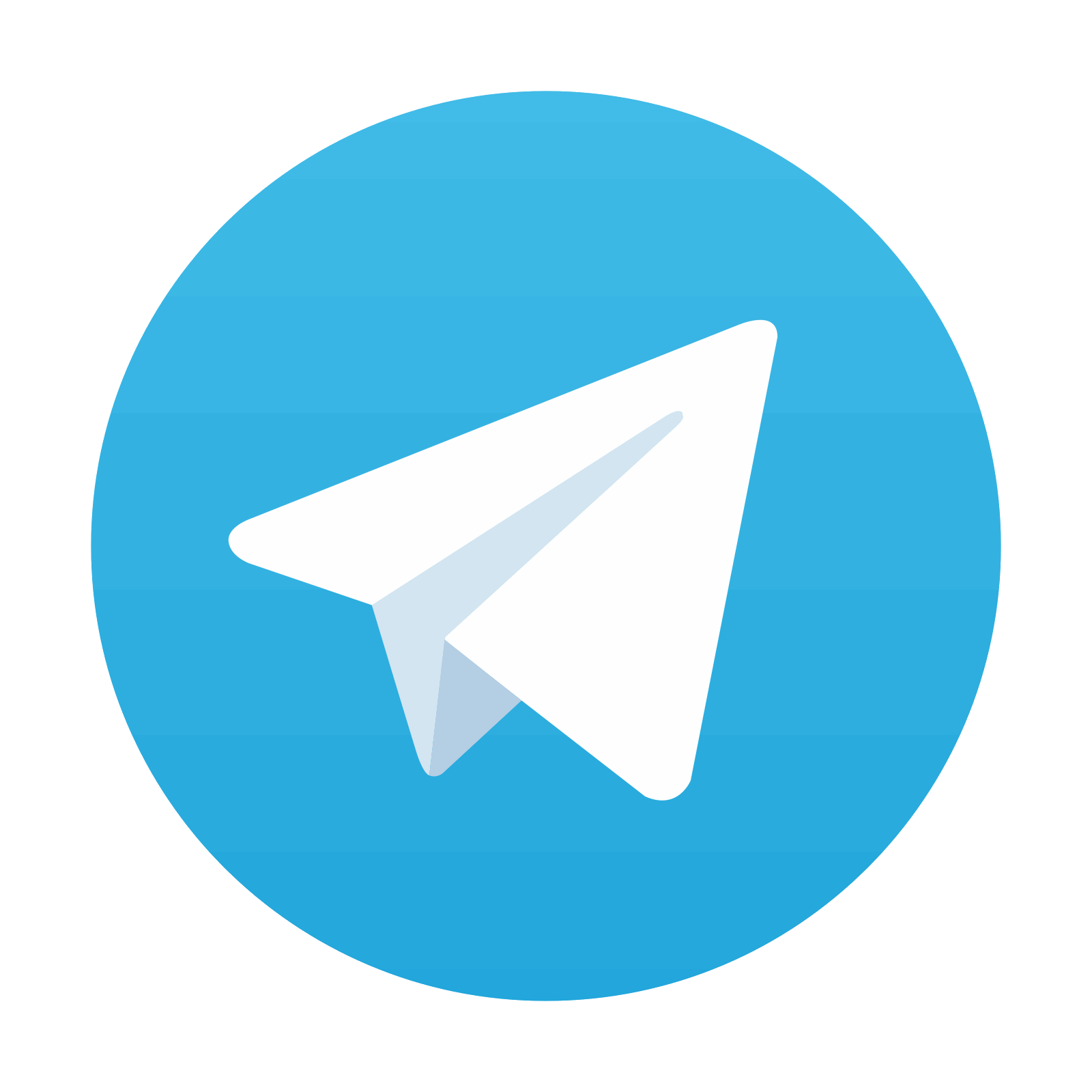
Stay updated, free articles. Join our Telegram channel

Full access? Get Clinical Tree
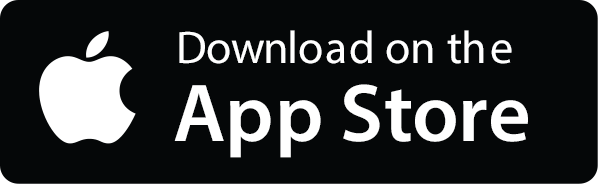
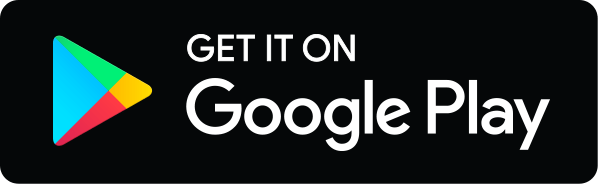