!DOCTYPE html>
11. Chronic Kidney Disease-Mineral and Bone Disorder, Vitamin D Deficiency, and Secondary Hyperparathyroidism
Abstract
Chronic kidney disease-mineral and bone disorder (CKD-MBD) encompasses laboratory and bone abnormalities and vascular or other soft tissue calcification and has adverse effects on clinical prognosis. The 2017 KDIGO clinical practice guideline recommends monitoring serum levels of calcium, phosphate, PTH, and alkaline phosphatase activity beginning in CKD G3a. Treatments of CKD-MBD should be based on serial assessments of phosphate, calcium, and PTH levels, considered together. In patients with CKD G3a–G5D with severe hyperparathyroidism who fail to respond to pharmacological therapy, we suggest parathyroidectomy. The ambiguity and lack of unequivocally actionable recommendations highlight potential challenges for implementation and underscore the need for future research in this important area.
11.1 Introduction
Disorders in calcium, phosphorus, and parathyroid hormone (PTH) are common in chronic kidney disease (CKD). In 2006, Kidney Disease: Improving Global Outcomes (KDIGO) published a position statement proposing a new approach to classification of bone and mineral disorders, termed as “chronic kidney disease-mineral and bone disorder” (CKD-MBD) [1]. KDIGO views CKD-MBD as a systemic disorder manifested by one or more of the following: (1) abnormalities of calcium, phosphorous, parathyroid hormone (PTH), or vitamin D metabolism, (2) abnormalities in bone pathology and histomorphometry marked by changes in bone turnover, mineralization, volume, linear growth, or strength (TMV system), and (3) the presence of vascular or other soft tissue calcification [1].
11.2 Pathophysiology
There exists evidence on bone remodeling that progresses with advanced kidney disease to stage 3 CKD, which is referred to as renal osteodystrophy. Interestingly, these changes may occur earlier [2, 3]. In a large cross-sectional study on MBD biochemical markers, serum PTH elevated in 12% of patients with an estimated glomerular filtration rate (eGFR) >80 mL/min/1.73 m2, whereas other biochemical parameters, serum phosphorous (Pi) and calcium (Ca) levels, remained within the normal range until eGFR <40 mL/min/1.73 m2 [3]. These bone changes are responsible for fractures, bone pain, immobility, and weakness [2, 4, 5], and the associated MBD biochemical disorders were associated with an increase in cardiovascular events and all-cause mortality [6–11]. Observational studies that included hemodialysis patients have reported the correlation of cardiovascular events with serum Pi, serum Ca and Pi product, and PTH levels, whereas a few observational studies that included predialysis patients showed that cardiovascular events have a similar correlation with elevated serum Pi and PTH levels [11, 12]. Elevation in PTH level in CKD patients is an adaptive response to an increase in serum Pi level from reduction of eGFR, a low serum Ca2+ level from a decrease in 1,25-dihydroxy vitamin D3 (1,25[OH]2D3, calcitriol) level, an increase in fibroblast growth factor (FGF)-23 level, and a decrease in calcitriol level from a reduction in 1-α-hydroxylase production, which is correlated with both renal osteodystrophy and the adverse clinical outcomes.
The term “renal osteodystrophy” was previously used to describe both bone histology and biochemistry findings and is currently defined based on only by histology and histomorphometric findings obtained by bone biopsy at an ideal site such as the iliac crest [2, 4]. Bone biopsy should be considered in advanced CKD patients with unexplained fractures, persistent bone pain, unexplained hypercalcemia, unexplained hypophosphatemia, possible aluminum toxicity, and prior to therapy with bisphosphonates [4]. There are four types of renal osteodystrophy: (1) osteitis fibrosis (high turnover) related to an elevated serum PTH level, (2) adynamic bone disease (low turnover), (3) osteomalacia (low turnover), and (4) mixed uremic osteodystrophy [2, 4, 12–14]. The 2017 KDIGO guideline states: “in patients with CKD G3a–G5D, it is reasonable to perform a bone biopsy if knowledge of the type of renal osteodystrophy will impact treatment decisions” [15].
The prevalence of CKD and osteoporosis is particularly increased in the elderly population in whom they commonly coexist, which may further complicate MBD [16, 17]. Osteoporosis is an absorptive process, whereas CKD-MBD is an imbalance of bone formation, mineralization, and volume, and both can result in fractures. Therapy differs for these two disorders; hence, differential diagnosis is very important to avoid inappropriate treatment. The dual-energy X-ray absorptiometry (DEXA), a two-dimensional bone density scan used to diagnose osteoporosis, may not be useful in differentiating these two disorders and, in fact, may sometimes overestimate bone mineral density (BMD) in moderate CKD [16]. Quantitative computerized tomography (qCT) and micro-magnetic resonance imaging (mMRI) better discriminate changes in the cortical and trabecular bone and changes in bone volume [16] and might aid in the differential diagnosis. However, the gold standard for differentiating these two disorders is the two-phase tetracycline labeling bone biopsy [16, 17].
11.3 Biochemistry in CKD-MBD
Hyperphosphatemia, hypocalcemia, low calcitriol level, elevated FGF-23 level, and elevated serum PTH level, all play important roles in the development and progression of CKD-MBD at different periods. As renal function declines, serum Pi level increases, which can stimulate the secretion of FGF-23 from osteocytes. There is a decline in the production of the 1-α-hydroxylase in the proximal convoluting tubule (PCT). The inactivity of the Na-Pi transporter can result in phosphaturia. Low calcitriol levels commonly seen in renal failure may be secondary to inhibition by FGF-23 rather than by a decrease of 1-α-hydroxylase in renal parenchymal [18–20]. More recently, FGF-23 and klotho also have been identified as important regulators of mineral metabolism. Klotho deficiency and high circulating FGF-23 levels precede secondary hyperparathyroidism in CKD patients. Levels of FGF-23 and PTH increase with the progression of CKD to overcome end-organ resistance and to maintain mineral homeostasis [21–23]. It is difficult to define when the increase of both hormones becomes maladaptive. The components of the mineral bone abnormalities and their contribution to MBD will be discussed individually. The 2017 KDIGO clinical practice guideline recommends monitoring the serum levels of calcium, phosphate, PTH levels, and alkaline phosphatase activity beginning in stage G3a CKD [15].
11.3.1 Calcium
Calcium is one of the main cations in the human body, which can maintain the structure of bone and regulate cell function. Furthermore, 99% of the body’s calcium is contained in skeletal and the intracellular compartment, and only 1% is available for calcium homeostasis [24, 25]. The normal serum calcium range in adults is 8.4–10.4 mg/dL, and 50% of serum calcium circulates freely, whereas the remainder is bound to albumin or anions. Serum calcium is maintained by various hormones such as PTH, calcitonin, and calcitriol, which in turn regulate the intestine, kidney, and bone to maintain serum calcium level [24].
In advanced CKD, calcium absorption in the small intestine is reduced secondary to a decrease of calcitriol and dietary intake. Elevation in Pi and a low serum Ca2+ level result in an increase of PTH secretion via the Ca2+ sensing receptor (CaSR) [24, 25]. This increase in serum PTH level can stimulate renal distal tubules to reabsorb more Ca2+ and an increase of bone resorption for attempting to maintain normal serum Ca2+ level.
11.3.2 Phosphate
Phosphorus (Pi) is an abundant element in the body and is essential for a wide variety of key biological processes. It plays an important role in cellular energy metabolism and cell signaling, e.g., adenosine and guanosine triphosphates (ATP, GTP), and in the composition of phospholipid membranes and bone, and is an integral part of DNA and RNA [24, 26]. In the body, 80–85% of Pi is in the skeleton [24], 1% is in the serum in an inorganic form [26], whereas the remainder is in other intracellular and extracellular compartments.
Pi homoeostasis is maintained by a balance between dietary Pi absorption by the gut, mobilization from bone, and renal excretion. The serum Pi is largely unbound [24], ranging from 2.5 to 4.5 mg/dL. Pi is absorbed in the small bowel under the influence of calcitriol. In the kidney, unbound Pi is filtrated by the glomerulus freely, of which 80–90% is reabsorbed in the PCTs (60–70%) and distal nephron (20–30%) [24]. In early CKD, the serum Pi level is maintained by reduced absorption in the GI tract due to decreased calcitriol level [24] and increased Pi excretion through decreased expression of the Na-Pi 2a co-transporter in the PCTs via the action of PTH [24, 26] and FGF-23 [22, 27].
Hyperphosphatemia is associated with a higher prevalence of soft tissue calcification, such as coronary arteries, descending aorta, aortic valve, and mitral valve [7]. Besides extra-skeletal calcification, hyperphosphatemia is also responsible for other adverse outcomes in CKD patients. A study reported that rats with CKD fed with high phosphate diet had higher heart weights than corresponding control rats [28].
11.3.3 Parathyroid Hormone
PTH is the major modulator of bone and mineral metabolism through its regulation of calcium and Pi homeostasis. Synthesis and cleavage of PTH occur within the parathyroid gland. PTH is a polypeptide synthesized in the endoplasmic reticulum following two successive cleavages: 115 amino acid pre-pro-PTH cleaved to 90 amino acid pro-PTH. Pro-PTH is then cleaved again to form an active mature full-length 84 amino acid PTH, with the first 6 amino acids accounting for its activity, while the 7–84 amino acids fragment protein is inactive [4]. The whole process of PTH synthesis, its cleavage and storage, is fast and has been estimated to take less than an hour. After release, PTH is rapidly removed from the serum by the kidney and the liver.
PTH has a major biological function in maintaining ionized calcium and phosphate within the reference range by stimulating specific receptor-mediated responses in cells throughout the body. If a decrease in circulating ionized calcium occurs, PTH increases and has three major functions that help to restore a normal circulating concentration: receptor-mediated tubular reabsorption of calcium (kidney); stimulation of osteoclast resorption to release skeletal calcium (bone); and increasing activity of renal 1-α-hydroxylase, resulting in production of 1,25[OH]2D3 and increasing calcium absorption (bowel). The increase in calcium in response to these effects mediated by PTH acts via a classic endocrine feedback loop on the CaSR, decreasing secretion of PTH [29–33]. PTH is a major regulator of bone turnover and acts directly on osteoblasts and indirectly on osteoclasts [24].
Increased serum PTH levels are prone in African Americans, the obese, and nondiabetic patients with CKD [11]. Although initial evaluation of MBD is recommended for patients with stage 3 CKD, some observational studies suggest that SHPT might occur earlier [3, 11]. In one study, elevated PTH levels were detected in 50.1% of participants with stage 3 CKD, which appeared to increase the risk for cardiovascular disease. Li et al. evaluated the association of iPTH with all-cause mortality in a cohort, comprising 8530 maintain hemodialysis patients who underwent 6–70 months follow-up (with a median of 40 months). Multivariate Cox regression analysis showed that patients with a low iPTH level (<75 pg/mL) at baseline had greater risk of mortality (HR = 1.36, 95% CI 1.16–1.60) than those with an iPTH level within 150–300 pg/mL at baseline [34]. Therefore, close surveillance for hyperparathyroidism should be recommended in patients with CKD.
11.3.4 Fibroblast Growth Factor 23
FGF23 is mainly produced in the bone and, upon secretion, forms a complex with a FGF receptor and coreceptor αKlotho [19, 33], induces renal phosphaturia by decrease 1,25[OH]2D3 synthesis, through enhanced expression of 24-hydroxylase, whereas 1,25[OH]2D3 itself increases FGF-23 transcription. An increase in PTH follows [18, 19, 34]. Healthy medical students on a high Pi diet showed an increase in serum FGF-23 levels at 16 h [33]. Their serum 1,25[OH]2D3 levels declined, and urinary Pi excretion increased. These parameters remained unchanged in the group on a normal Pi diet [33]. An elevated level of FGF-23 may be a mechanism to maintain Pi homeostasis in early CKD [32, 33].
The FGF-23 receptor complex, Klotho–FGFR1, is expressed in the parathyroid cells, and a challenge with recombinant FGF-23 activates the mitogen-activated protein kinase (MAPK) 1 pathway to decrease PTH mRNA levels and PTH secretion both in vivo and in vitro [35, 36]. In both experimental CKD and in parathyroid tissue from patients with CKD, serum PTH and FGF-23 levels are markedly increased, suggesting a resistance of the parathyroid gland to FGF-23. This resistance is caused by a decreased expression of the Klotho–FGFR1 complex in the parathyroid gland in stage 5 CKD and prolonged experimental renal failure but not in short-term experimental renal failure [37, 38].
11.3.5 Vitamin D
Vitamin D3 (cholecalciferol), the natural form of vitamin D, is a steroid hormone that can be synthesized endogenously or taken in from the diet. In the skin, irradiation of 7-dehydrocholesterol produces pre-vitamin D3 that is immediately converted to cholecalciferol. The production of vitamin D in the skin is the most important source of vitamin D and depends on the intensity of UV exposure. Vitamin D can also be provided to a small extent in the diet, being mainly present in fish oils and fortified dairy products [39]. Vitamin D3 is transported to the liver by vitamin D-binding protein (VDBP), which is subsequently converted to calcidiol by 25-hydroxylase (CYP2R1). Serum calcidiol reflects total body vitamin D from dietary intake, skin, and liver stores [39]. Circulating calcidiol bound to VDBP is taken up by megalin-mediated endocytosis in the PCTs and converted to calcitriol, the active form, by 1-α-hydroxylase (CYP2B1) [40]. Serum calcitriol level is under the control of phosphorous, FGF-23, PTH, and calcitriol (negative feedback) [18, 19, 39, 40].
Vitamin D deficiency (<20 ng/mL) and insufficiency (20–29 ng/mL) are common among patients with CKD, particularly the elderly and advanced CKD [39, 40]. In addition to nutritional and sunlight exposure deficits, factors that affect vitamin D deficiency include race, gender, age, obesity, dietary intake and impaired vitamin D synthesis and metabolism [39]. With the deterioration of renal function, serum 1,25[OH]2D3 levels also decrease progressively because of vitamin D3 deficiency, together with impaired availability of CYP2B1 by renal PCTs, high FGF-23.
Vitamin D is involved in the regulation of bone metabolism, skeletal muscle strength and mobility, and in the maintenance of calcium and phosphate homeostasis [39, 40]. Observational studies showed that vitamin D has some beneficial extraosseous functions involving cardiac and immune system. Insufficient vitamin D is related to high-renin hypertension and diabetes [39, 40], whereas sufficient vitamin D is associated with improved outcomes in these diseases [41]. Together with the progressive decline of serum calcitriol, vitamin D deficiency leads to SHPT and its complications, tertiary hyperparathyroidism and hypercalcemia, which require surgical parathyroidectomy or calcimimetics. As in the general population, this condition is associated with increased morbidity and poor outcomes.
11.3.6 Bone
Owing to the lack of evidence showing that BMD measured by DEXA predicts fractures in patients with CKD as it does in the general population, and to the inability of DEXA to indicate the histological type of bone disease, the 2009 guideline recommended that BMD measurement be not routinely performed in patients with stage CKD G3a–G5D and CKD-MBD [4]. An evidence-based review of the 2017 KDIGO CKD-MBD guideline update identified four prospective cohort studies on adults [15], showing that BMD measured by DEXA predicted fractures in the entire spectrum from stage CKD G3a–G5D [42–45]. These studies represent a significant progress since the publication of the original 2009 guideline. Based on these insights, the KDIGO work group concluded that BMD assessment by DEXA is recommended if low or declining BMD will lead to additional interventions for the reduction in falls or the use of medications for osteoporosis.
The term renal osteodystrophy (ROD) is defined as alterations in bone physiology that can be detected histologically and includes CKD-mediated changes in bone turnover, mineralization, and volume (TMV). Double tetracycline labeled bone biopsy is thus far the gold standard for the diagnosis and classification of ROD. Over the past few years, bone biopsy studies have yielded several clinically important insights into CKD-related bone dysfunction and have defined a critical role for bone as an endocrine organ, responsible for many of the complications that accompany CKD-MBD. The 2009 guideline recommended a bone biopsy prior to anti-resorptive therapy in patients with stage G4–G5D CKD and evidence of biochemical abnormalities in CKD-MBD, low BMD, and/or fragility fractures [4]. However, the KDIGO work group understands clinical experience concerning the performance and evaluation of bone biopsies may be limited. There is growing evidence that anti-resorptive therapies are effective in patients with stage G3a–G4 CKD; however, no robust evidence suggests that anti-resorptive medications induce adynamic bone disease. Therefore, the 2017 guideline update no longer suggests performing bone biopsy be performed prior to initiation of these medications [15].
11.3.7 Vascular Calcification
Cardiovascular complications are the main clinical problems in patients with CKD, and the main cause of death in patients with end-stage renal disease [46, 47]. The combination of vascular calcification and disturbed bone metabolism in CKD has been termed as CKD-MBD by the KDIGO in 2009 [4]. Increased vascular calcification in CKD patients is an important risk factor for poor prognosis in overall survival, cardiovascular morbidity and mortality [47–49]. Vascular calcification, previously considered as a passive deposition of calcium and phosphate hydroxyapatite, is now considered as a cellular regulatory process in which vascular smooth muscle cells (VSMCs) undergo molecular and phenotypic changes similar to bone formation during embryogenesis. Paradoxically, in both patients with CKD and animal models of CKD, bone loss occurs simultaneously with increased vascular calcification [50], suggesting that different intrinsic signals in tissue-specific microenvironments may dominate bone and vascular mineralization. The exact mechanism of vascular calcification in CKD and the contribution of impaired bone metabolism to vascular calcification have not been fully elucidated. Systemic uremic factors and bone metabolic disorders play an important role in the pathogenesis of vascular calcification in CKD. The regulation of Runt-related transcription factor 2 (Runx2), a key transcription factor for bone formation, and the emerging role of Runx2-dependent receptor activator of nuclear factor kappa-B ligand (RANKL) in vascular calcification of CKD were emphasized.
For patients with CKD G3a–G5D, we recommend that abdominal lateral radiographs be used to detect the presence or absence of vascular calcification, and echocardiography be used to detect the presence or absence of valvular calcification, as a reasonable alternative to CT-based imaging. We recommend that patients with CKD G3a–G5D with known vascular or valvular calcification be considered to have highest cardiovascular risk. It is reasonable to use this information to guide the management of CKD-MBD.
11.4 Treatment of CKD-MBD
Management of CKD-MBD requires understanding of the complex interactions between ions, hormones and their target organs. Since the release of KDIGO CKD-MBD guidelines in 2009, our understanding of the pathophysiology has improved. However, there is still a lack of high-quality clinical evidence to support specific interventions. Using available data, KDIGO has now updated diagnosis and treatment recommendations for patients with CKD-MBD. In patients with CKD G3a–G5D, the therapy of CKD-MBD should be based on a series of measurements of phosphate, calcium, and PTH levels.
11.4.1 Treatment Targeted at Lowering High Serum Phosphate Level and Maintaining Serum Calcium Level
With the deterioration of renal function, the iPTH level increases earlier than Pi as a response to maintain serum Pi, the later does not increase until the eGFR falls below 40 mL/min/1.73 m2 [3], and FGF-23 acts similarly to iPTH. In an animal study performed in dogs, when the dietary Pi intake was titrated to renal function decline, serum iPTH and Pi levels, and excretion of Pi remained unchanged [51]. This study highlights the importance of dietary Pi restriction in patients with CKD. When kidney disease progresses, for PTH and FGF-23, it is harder to maintain serum Pi in normal range without damaging other organs. The serum Pi level target for the patient with stage G3a–G5D CKD is toward the normal range, and phosphate binders will be prescribed if this target is not achieved by diet Pi restriction alone. The available Pi binders are classified as either calcium based or non-calcium based [52–54]. The former includes calcium carbonate and calcium acetate, whereas the latter includes sevelamer carbonate or hydrochloride (an anion exchange resin), lanthanum carbonate, and aluminum hydroxide. Aluminum hydroxide is now restricted in patients with advanced CKD [55, 56] because of the extrarenal side effects which include low turnover bone decrease, skeleton fractures, encephalopathy, skeletal muscle weakness, and microcytic anemia without iron shortage [19, 57, 58]. Chertow GM et al. compared calcium acetate with sevelamer with respect to the development and progression of calcification in coronary artery and aorta using electron beam technique (EBT) in dialysis patients [60]. At baseline, both groups had severe extensive vascular calcifications. At the end of the 2-year follow-up, there was no further progression of calcification in the sevelamer group, but a significant increase in calcification in the coronary artery and aorta was observed in the calcium acetate group [60]. A systematic review [63] of 60 trials found that although sevelamer hydrochloride significantly reduced serum Pi and PTH levels compared with calcium-based binders, the use of sevelamer was associated with a higher risk of gastrointestinal side-effects but lower risk of hypercalcaemia. There was no significant reduction in all-cause mortality with sevelamer hydrochloride compared with calcium-based phosphate binders.
In adult patients with stage G3a–G5D CKD, we suggest that hypercalcemia be avoided. In children with stage G3a–G5D CKD, we suggest that the serum calcium level be maintained in the age-appropriate normal range. In patients on maintain hemodialysis, we suggest the use of a dialysate calcium concentration between 1.25 and 1.50 mmol/L (2.5 and 3.0 mEq/L). The association between calcium-based Pi binders and extraskeletal calcifications has led to the prescription of non-calcium-based Pi binders. If calcium-based Pi binders are prescribed, the dose should be limited to 1.5 g of elemental calcium per day [14, 59–62]. In adult patients with stage G3a–G5D CKD receiving phosphate-lowering treatment, we suggest that the dose of calcium-based phosphate binders should be restricted. In children with CKD G3a–G5D, it is reasonable to base the choice of phosphate-lowering treatment on serum calcium levels.
11.4.2 Treatment of Abnormal PTH Levels in CKD-MBD
In patients with stage G3a–G5 CKD not on dialysis, the appropriate PTH level is yet not known until now. Even so, we recommend that patients with iPTH levels gradually increasing or continually exceeding the upper normal limit should be assessed for modifiable factors, such as hyperphosphatemia, hypocalcemia, hyperphosphate diet, and vitamin D deficiency.
The updating of the KDIGO guidelines in 2017 gives the following reasons for its emphasis on not starting treatment for a single elevated PTH value: (1) the optimal PTH level is still unclear; (2) a moderate increase in PTH level is an appropriate adaptive response that contributes to phosphaturia in CKD and may therefore be conducive to maintain normal serum phosphate levels as GFR decreases; and (3) high PTH level does not predict high turnover osteopathy, so it is not necessary to correct the slightly elevated PTH levels to normal levels in CKD. The KDOQI work group agreed with the update and its reasons, particularly in view of the high variability of PTH levels within and between individuals [64–67].
We recommend unconventional prescriptions of calcitriol and vitamin D analogues in adult patients with stage G3a–G5 CKD not on dialysis. It is reasonable to retain the use of calcitriol and vitamin D analogues for patients with stage G4–G5 CKD with severe or progressive hyperparathyroidism. In children, calcitriol and vitamin D analogues can be used to maintain serum calcium levels within normal age-appropriate ranges. The PRIMO and OPERA studies demonstrated that in patients with non-dialysis-dependent CKD, the use of vitamin D analogues may be associated with significant reductions in PTH levels, no change in cardiovascular end points, and significant increases in risk for hypercalcemia [68, 69].
For patients with stage G5D CKD, we recommend that iPTH levels be maintained within about 2–9 times the normal upper limit of the test. We suggest that significant changes in iPTH levels beyond this range should be avoided, either in therapy or not. For patients with stage G5D CKD requiring PTH reduction therapy, we recommend the use of calcimimetics, calcitriol, vitamin D analogues, or a combination of calcimimetics with calcitriol or vitamin D analogues. Calcimimetics, calcitriol, and vitamin D analogues have shown efficacy in lowering the PTH level in the patients with stage G5D CKD in the United States [70–72]. Although pre-specified secondary analysis and post hoc analysis of the EVOLVE data noted improvement in some outcomes in a subgroup of patients (e.g., a reduction in fracture incidence when treated with cinacalcet vs placebo in patients aged >65 years) [73], the results of preliminary analysis were not positive. Since the advantage of cinacalcet over other drugs has not been established, the selection of PTH reduction treatment in patients with stage G5D CKD may be based on cost, adverse events, and presence of other mineral metabolism abnormalities.
11.4.3 Parathyroidectomy
For patients with stage G3a–G5D CKD with severe hyperparathyroidism who fail to respond to medical or pharmacological treatment, we recommend parathyroidectomy. In tertiary hyperparathyroidism, the parathyroid gland becomes autonomous, nodular in composition, and unresponsive to feedback inhibition by vitamin D analogues or cinacalcet owing to a reduction in VRD and CaSR. For more than six decades, many patients with advanced CKD have undergone surgical parathyroidectomy (PTX) for severe SHPT mainly according to historical clinical practice patterns. Mugurel Apetrii et al. conducted a meta-analysis to evaluate the benefits and hazards of PTX in patients with SHPT [74]. The final analysis included 15 cohort studies, comprising 24,048 participants. Compared with standard therapy, PTX significantly reduced all-cause mortality (RR 0.74 [95% CI, 0.66 to 0.83]) in patients with end-stage renal disease who had biochemical and/or clinical evidence of SHPT. In six observational studies involving nearly 10,000 patients, PTX was also associated with reduced cardiovascular mortality (RR 0.59 [95% CI, 0.46 to 0.76]). In conclusion, the results of this meta-analysis suggest that PTX has a significant clinical benefit in all-cause and cardiovascular mortality in CKD patients with SHPT. Our study showed that total parathyroidectomy without autotransplantation could improve BMD at the L1–L4 level and the hip in SHPT patients [75]. However, in view of the observational nature of the studies included, there is a strong demand for appropriately performed, independent randomized controlled trials to compare surgery with medical treatment and featuring many different outcomes from mortality to quality of life.
Due to the complexity of basic pathophysiology, the lack of evidence certainty, and multiple morbidities in the patient population, clinical providers in the kidney care setting often encounter patients with CKD-MBD and strive to find treatment methods. The ambiguity and lack of clear and actionable recommendations highlight potential challenges in implementation, remind us that clinical practice guidelines should be used in conjunction with clinical judgments, and emphasize the need for future research in this vital area.
Key Messages
CKD-MBD is a systemic disorder manifested by one or more of the following: (1) abnormalities of calcium, phosphorous, PTH, or vitamin D metabolism, (2) abnormalities in bone pathology and histomorphometry marked by changes in bone turnover, mineralization, volume, linear growth, or strength (TMV system), and (3) the presence of vascular or other soft tissue calcification.
The 2017 KDIGO clinical practice guideline recommends monitoring the serum calcium, phosphate, and PTH levels and alkaline phosphatase activity beginning in stage G3a CKD.
Treatments of CKD-MBD should be based on serial assessments of phosphate, calcium, and PTH levels, considered altogether.
In patients with stage G3a–G5D CKD with severe hyperparathyroidism who fail to respond to medical or pharmacological therapy, we suggest parathyroidectomy.
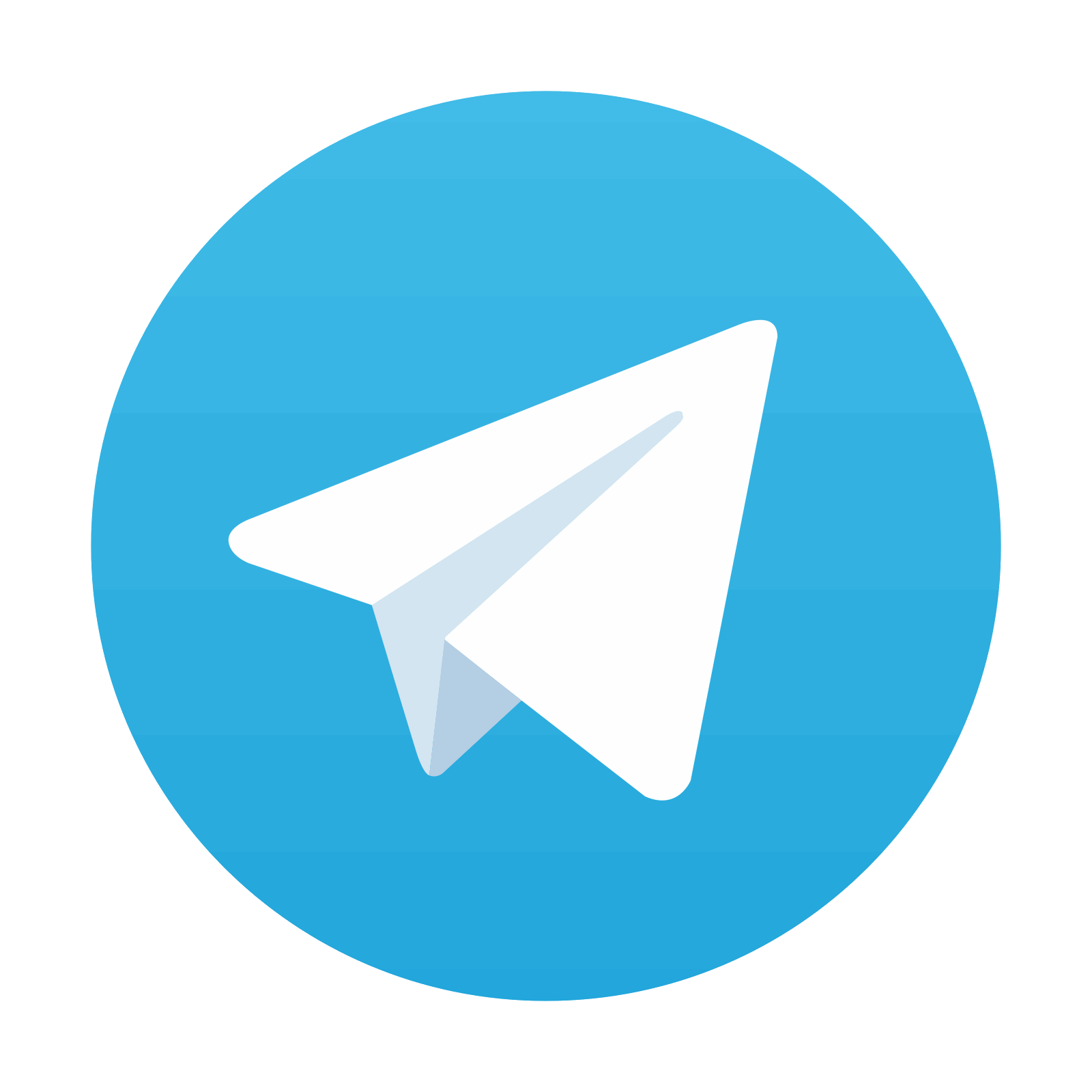
Stay updated, free articles. Join our Telegram channel

Full access? Get Clinical Tree
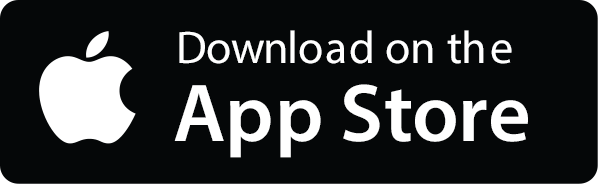
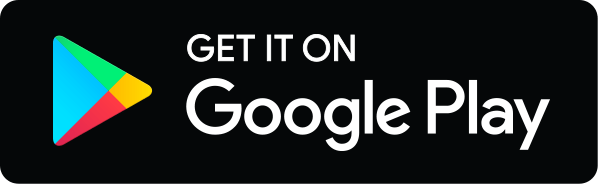