Computer-aided surgical navigation systems with fusion or overlaying capability, coupled with newer position tracking systems, can provide new opportunities to improve the precision of minimally invasive urology. Three-dimensional feedback of spatial position of surgical targets, and the predictive ability to guide laparoscopic dissection along the ideal surgical plane, with built-in safety checks and balances may help to lay the foundation for automated surgery in the future.
Precise intraoperative decision-making requires extensive understanding of the three-dimensional topography of the surgical target, the three-dimensional course of the surrounding vasculature, and the proximity of vital anatomic structures in advance of embarking on the actual surgical procedure. Such information is typically obtained from evaluation of high-resolution radiographic images, such as CT or MRI; the surgeon’s knowledge of the relevant surgical anatomy; and the surgeon’s prior experience and expertise. The emergence of laparoscopic and robotic minimally invasive surgery has served to increase the surgeon’s dependence on preoperative imaging. Although the magnification or three-dimensional picture capabilities of current endoscopes provides unparalleled visualization of the surgical field during laparoscopic and robot-assisted surgery, tactile feedback is somewhat diminished, increasing reliance on visual cues. As such, the novice surgeon’s ability to be knowledgeable of anatomic structures beyond the endoscopic view may be somewhat decreased. Conventional intraoperative imaging for surgical navigation, such as two-dimensional real-time ultrasonography (US), has the considerable issue of being operator-dependent as regards expertise in performing the procedure, and in data interpretation. Intraoperative capability to visualize typically invisible surgical anatomy would be a considerable advance.
The recent revolution in high-speed computing, digital imaging software, and position-tracking technologies can provide new opportunities for real-time intraoperative surgical navigation. Fused-image or overlaid three-dimensional imaging for surgical navigation could be constructed from any imaging modality, such as preoperative CT, MRI, or positron emission tomography scanning, or intraoperative US, and serve to enhance the surgical capabilities of the surgeon.
“Virtual reality” implies surgical simulation of the three-dimensional world, for the purposes of educational training or preoperative surgical planning. “Augmented reality” (AR) involves integrating radiologic images of anatomy with the real intraoperative view of the patient’s anatomy to create computerized three-dimensional images of real surgical targets for the purpose of intraoperative decision-making.
The authors initially reported the application of a US and CT-MRI fusion system to facilitate US-guided intervention. They also first used AR technology in various urologic-oncologic laparoscopic procedures. Their ongoing aims are to develop a predictive surgical navigation system to guide the surgeon toward the ideal surgical direction or surgical plane before actually performing the surgical dissection to enhance intraoperative precision.
Computer-aided image-guided surgery
Every imaging modality has inherent advantages and disadvantages. Because most current imaging systems acquire and store data in a digital format they can be synchronized and fused using specialized software to create a system that combines advantages of two imaging modalities. Image-fusion systems provide a new opportunity to enhance the ability of an imaging modality by compensating for its shortcomings by fusing an additional imaging modality. Real-time virtual sonography (RVS) is the image-fusion system of real-time US with preoperative CT or MRI. Herein, US provides real-time percutaneous or transrectal guidance; superimposition of preoperative CT or MRI images enhances the precision of the US-guided intervention. In RVS, images of the same section of the body are dynamically fused from its US plus its CT-MRI iterations, both images being synchronously shown in a side-by-side display. The unique advantages of each imaging modality are taken advantage of: the real-time images of US and the high-precision anatomic images of CT-MRI.
AR uses computerized digital imaging technology to project superimposed three-dimensional images onto the real-time endoscopic view. The authors used AR in a series of laparoscopic urologic surgical cases, mainly laparoscopic partial nephrectomy and laparoscopic radical prostatectomy. During renal surgery, they primarily used preoperative CT data, whereas during prostate surgery they used preoperative MRI data and intraoperative transrectal US.
Real-time virtual sonography
During percutaneous US-guided intervention, US allows real-time monitoring of the insertion of a needle into the target. The predicted puncture line is demonstrated on a display. For the novice practitioner, however, difficulty is often encountered during continuous visualization of the needle tip, the target organ, and adjacent anatomic structures, all of which have to be spatially located in the plane of the projected US beam. Difficulties include not only the technical visualization of the advancing needle, but also real-time interpretation of the US image. The learning curve with US-guided interventions can often be significant. The reflected US echoes make it difficult to capture precise images through stone, bone, gas, or air. Urologists typically prefer CT or MRI as the imaging modality of choice because of the higher resolution and availability of volume data acquisition. Taken together, the disadvantages of US-guided intervention include the relatively inferior image quality of US, especially for deep lesions located distant from the US probe, and operator dependence for technical aspects and interpretation.
For energy-based needle ablation of renal tumor (cryoablation, radiofrequency ablation), reliable real-time image-guidance is necessary for (1) precise needle placement, (2) monitoring the entire extent of the ablative lesion, and (3) evaluation of ablation outcome. US can monitor ice-ball formation, yet visualization of the deep edge of the lesion is difficult, because US energy cannot penetrate through the body of the ice-ball. CT-guidance provides accurate images for cryoablation of renal tumors. Repeated CT-guided procedures confer the hazard of radiation-induced morbidity. Clearly, in children or young patients, US guidance is the safer choice.
Fundamentally, the RVS system constructs a tomogram from a previously acquired CT-MRI volume data-set and displays it in a synchronized manner along with the real-time US image. The physical position of the US transducer is tracked with an attached magnetic position sensor ( Fig. 1 ).
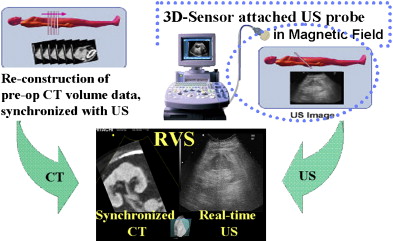
The RVS system is comprised of (1) an US machine and display panel, (2) a computer work-station, (3) a magnetic field generator, and (4) an US probe with attached magnetic sensor. First, the Digital Imaging and Communication in Medicine formatted volume data of preoperative CT-MRI is obtained in the workstation of the RVS system before intervention. Second, this CT-MRI volume data is point-registered with the actual surgical target using fiducial or cross-section registration. Because a magnetic sensor is attached on the US probe in the RVS system, the angle and spatial position of the US probe is tracked in real-time, and the information is fed into the computer workstation. Movements of the magnetic sensor–attached US probe within the generated magnetic field around the body can be precisely tracked. The CT-MRI image can be automatically determined and reconstructed in real-time from the volume data, according to the real-time positional information of the US probe, which consists of both angle (to the x-, y-, or z-axis) and spatial position (x, y, z) of the US probe. The reconstructed “virtual” image of CT-MRI can be displayed in parallel with the US image for the same tomogram cut of the body in real-time ( Fig. 2 ).
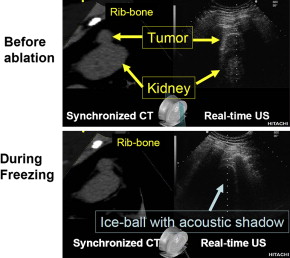
The most important advantage of the RVS is its presentation of enhanced high-resolution volume data. Any CT image (arterial phase, venous phase, delayed enhanced phase) or enhanced or functional MRI image can be selected from the preoperatively acquired volume data. Enhanced imaging, such as that provided by CT-MRI, can now be used for real-time navigation, while decreasing radiation exposure and costs.
The attractive feature of RVS is its side-by-side presentation of the real-time US and preoperative CT-MRI images (see Fig. 2 ). In monitoring the changing target lesion during ablative treatment, the display of the preoperative status, at the same tomogram level as the real-time US, can be important for confirming precision and safety of the ablation zone. Availability of only the real-time US image after the formation of an ice-ball requires the surgeon to maintain a perfect mental picture of the spatial position of the pretreatment target lesion (whose shape may have become distorted by the ice-ball). Parallel displays of the initial and current status of the targeted tumor allow decision-making regarding the adequacy of the current treatment, and the possible necessity of an additional session to ablate completely the entire target.
For prostate applications, RVS is now also available for transrectal US-navigation fused with MRI. The high resolution of functional dynamic contrast-enhanced magnetic resonance imaging (DCE-MRI) could provide accurate diagnosis of prostate cancer in the future. Fusion of real-time images of needle ablation by transrectal RVS combined with high-resolution imaging of the prostate cancer nodule by functional DCE-MRI would enhance localization and targeted focal destruction. The authors’ experience with RVS fusion of real-time transrectal US with high-resolution 3-T MRI volume data allows precise needle placement into the suspected lesion.
Real-time virtual sonography
During percutaneous US-guided intervention, US allows real-time monitoring of the insertion of a needle into the target. The predicted puncture line is demonstrated on a display. For the novice practitioner, however, difficulty is often encountered during continuous visualization of the needle tip, the target organ, and adjacent anatomic structures, all of which have to be spatially located in the plane of the projected US beam. Difficulties include not only the technical visualization of the advancing needle, but also real-time interpretation of the US image. The learning curve with US-guided interventions can often be significant. The reflected US echoes make it difficult to capture precise images through stone, bone, gas, or air. Urologists typically prefer CT or MRI as the imaging modality of choice because of the higher resolution and availability of volume data acquisition. Taken together, the disadvantages of US-guided intervention include the relatively inferior image quality of US, especially for deep lesions located distant from the US probe, and operator dependence for technical aspects and interpretation.
For energy-based needle ablation of renal tumor (cryoablation, radiofrequency ablation), reliable real-time image-guidance is necessary for (1) precise needle placement, (2) monitoring the entire extent of the ablative lesion, and (3) evaluation of ablation outcome. US can monitor ice-ball formation, yet visualization of the deep edge of the lesion is difficult, because US energy cannot penetrate through the body of the ice-ball. CT-guidance provides accurate images for cryoablation of renal tumors. Repeated CT-guided procedures confer the hazard of radiation-induced morbidity. Clearly, in children or young patients, US guidance is the safer choice.
Fundamentally, the RVS system constructs a tomogram from a previously acquired CT-MRI volume data-set and displays it in a synchronized manner along with the real-time US image. The physical position of the US transducer is tracked with an attached magnetic position sensor ( Fig. 1 ).
