Fig. 14.1
Proposed pathogenesis of hypervolemic hyponatremia in cirrhosis. Portal hypertension causes splanchnic vasodilatation with subsequent activation of the renin–angiotensin–aldosterone system (RAAS) and sympathetic nervous system (SNS), and a nonosmotic hypersecretion of arginine vasopressin (AVP) is due to decreased effective arterial blood volume that activates baroreceptors and stimulates the hypothalamic release of AVP causing renal solute-free water retention through the action of V2 receptors and hypervolemic hyponatremia
Located on the basolateral (capillary) membrane of renal collecting duct principal cells, V2 receptors are responsible for the AVP-induced solute-free water reabsorption [3, 16–19]. The binding of AVP to the V2 receptor stimulates adenyl cyclase via a stimulatory G protein. The resulting intracellular cyclic AMP (cAMP) binds to a regulatory subunit of protein kinase A and activates it, which in turn phosphorylates aquaporin 2 (AQP2). AQP2 is then translocated from cytosolic vesicles to the luminal (apical) plasma membrane of the principle cell where it increases water permeability facilitating water reabsorption down a gradient [3]. The water entering the cell by the luminal plasma membrane leaves the cell through the basolateral membrane and enters the capillaries in contact with the tubular cells. Data from patients with cirrhosis and hypervolemic hyponatremia in whom V2 receptor antagonists of AVP (vaptans) were administered indicate that hypersecretion of AVP plays a major role in the development of hyponatremia because these drugs increase the serum sodium concentration in a large proportion (60–70 %) of patients [20]. However, there are a number of patients in whom serum sodium levels do not increase with vaptans which suggests that other mechansims involved in solute-free water retetion play an important role in the pathogenesis of hypervolemic hyponatremia in cirrhosis.
Clinical Features
The specific clinical consequences of hypervolemic hyponatremia for patients with cirrhosis are largely obscure. Given that hyponatremia occurs in the setting of advanced liver failure, the symptoms with which patients with hyponatremia may present are often attributable to concomitant cirrhotic complications, including encephalopathy, renal failure, and infection. Furthermore, the lack of effective treatments for hyponatremia does not permit a controlled evaluation of symptoms in the presence and absence of hyponatremia.
Neurological Features
When it develops in the absence of liver disease, hyponatremia is primarily associated with a wide range of neurological manifestations. Owing to compensatory intracellular water shifts, these manifestations are related to the development of brain edema, such as headache, confusion, focal neurological deficits, seizures, and, in some cases, death due to cerebral herniation [2]. The severity of neurological symptoms in patients with hyponatremia without liver disease correlates reliably with serum osmolality and sodium. Given the existence of homeostatic compensatory mechanisms, rather than the absolute reduction in serum sodium levels, the most important determinant of the severity of neurological symptoms is the rate of fall in serum sodium levels [2]. Patients with acute hyponatremia have a much higher incidence of neurological symptoms than those with chronic hyponatremia.
No study has specifically evaluated neurological symptoms in patients with cirrhosis and hyponatremia. Clinical experience, however, suggests that neurological manifestations such as headache, focal deficits, seizures, and cerebral herniation are very uncommon. The relatively low incidence of neurological manifestations in patients with cirrhosis and dilutional hyponatremia is likely related to timescale over which their sodium concentration falls, allowing sufficient time for adaptation. In most patients with cirrhosis, hyponatremia is asymptomatic but may be associated with a higher risk of hepatic encephalopathy (HE) [21–23]. The underlying pathogenesis of HE is partially based on ammonia and other toxins inducing low-grade cerebral edema due to astrocyte swelling. When portosystemic shunting results in elevated ammonia levels, the astrocyte begins metabolizing excess ammonia via intracellular glutamine synthetase. The result is increased intra-astrocyte levels of glutamine that both acts as an osmolyte and alters astrocyte function [24]. Accordingly, the low serum osmolality reflective of hyponatremia likely contributes to HE by exacerbating astrocyte swelling as the brain takes on water to compensate for serum changes (Fig. 14.2). Consequences that attend astrocyte swelling include alterations in gene expression and oxidative stress that alter glioneuronal communication and disturb neurological function, leading to encephalopathy [24, 25]. Hyponatremia in combination with hyperammonemia lead to astrocyte swelling and may increase the risk of HE.


Fig. 14.2
Proposed interaction between hyperammonemia and hyponatremia on brain astrocytes and possible pathogenic relationship with hepatic encephalopathy
Complications of Cirrhosis
Beyond HE, hyponatremia is also associated with other complications of cirrhosis. As discussed above, in the majority of patients, hyponatremia is a marker of illness and advanced liver failure, often occurring in close association with renal failure and correlates with poor prognosis [15, 26]. Patients with ascites and hyponatremia constitute a population at a very high risk of developing HRS [27]. On the other hand, low serum sodium levels are a very common finding in patients with HRS. Hyponatremia is also a frequent finding in patients with cirrhosis and bacterial infections. Furthermore, owing both to the illness it reflects, its toxic effects and the results of the fluid intake restrictions imposed to prevent its progression, hyponatremia is an independent predictive factor of the impaired health-related quality of life [28].
Management of Hyponatremia
The first step in the management of hyponatremia in cirrhosis is to determine the patient’s volume status. The management of hypovolemic hyponatremia is fundamentally different from that of hypervolemic hyponatremia. Regardless, diuretic treatment—for its effect of intravascular volume and natriuresis, should be stopped in all patients with hyponatremia [4, 5]. Thereafter, hypovolemic hyponatremia treatment consists of the identification and treatment of the cause of sodium loss together with the aim of repleting extracellular sodium (either intravenous saline or a diet with normal sodium content) .
The management of hypervolemic hyponatremia, on the other hand, aims to reduce total body water and requires maneuvers to increase renal solute-free water excretion. The available therapeutic methods for the management of hypervolemic hyponatremia are summarized below.
Fluid and Water Restriction
Fluid restriction (1.5 L/day) is still considered the first step in the management of hypervolemic hyponatremia [5]. There are no studies specifically assessing the effectiveness of fluid restriction in this setting but it is likely necessary to prevent a progressive decrease in serum sodium levels. Fluid restriction rarely increases serum sodium concentration in a significant manner, largely because the volume restriction required to effect significant changes—generally 500 mL—are profoundly less than typically prescribed by physicians or tolerated by patients [29].
Sodium Chloride
The use of intravenous hypertonic sodium chloride is neither advisable nor previously investigated in randomized studies of patients with cirrhosis. There are three reasons. First, the effect of hypertonic sodium is short-lived. Second, it has no effect on renal solute-free water excretion. While cardiac underfilling in patients with cirrhosis trigged significant AVP-mediated free water retention, it also stimulates sodium avidity via the renin–angiotensin system. Accordingly, third, hypertonic saline will invariably increase the patient’s ascites and edema.
Albumin
Two short-term studies, one in 1990 and the other only published in abstract form, including a low number of patients suggest that the administration of albumin could improve serum sodium concentration in patients with hypervolemic hyponatremia [30, 31]. By improving circulatory function, albumin likely suppresses the sodium and water-retaining systems, including nonosmotic AVP release. Unfortunately, the effects of albumin infusion were studied over only 1 week. As the half-life of infused albumin is short, the changes it can bring are fundamentally short lived. It is also a costly therapy. Accordingly, further studies should focus on the subset of high risk patients that would benefit from a short-term therapy, namely, those with profound hyponatremia awaiting liver transplantation.
AVP Antagonists: The Vaptans
The pharmacological approach to the treatment of hypervolemic hyponatremia appeared revamped with the introduction of vaptans. These drugs cause a selective blockade of the V2 receptors of AVP in the principal cells of the collecting ducts [32]. Vaptans are aquaretics. In healthy subjects, vaptan treatment induces a marked and dose-dependent increase in urine volume with low urine osmolality due to a marked increase in solute-free water excretion, but without an increase in urinary sodium excretion. Randomized, double-blind, comparative studies indicate that treatment with oral vaptans for a short period of time (up to 1 month), including tolvaptan, lixivaptan, and satavaptan, improves serum sodium concentration in patients with cirrhosis and hypervolemic hyponatremia [33–37]. A small study suggests that intravenous conivaptan, a vaptan that is not only an antagonist of the V2 receptors but also of the V1 receptors of AVP, is also effective in patients with cirrhosis and hyponatremia [38]. The increase in serum sodium concentration occurs within the first 7 days of treatment and normalization of serum sodium concentration has been observed in up to 80 % of patients [33–37]. A specific analysis in patients with cirrhosis and hyponatremia revealed a significant increase in free water clearance associated with weight loss without renal impairment and normalization of serum sodium to > 135 mEq/L in 41 % of patients at day 4 and 33 % at day 30 [37] (Fig. 14.3). A secondary analysis also found a significant improvement in health-related quality of life scores in patients treated with tolvaptan [37]. Hyponatremia reliably recurs upon treatment discontinuation. Unfortunately, for some patients, as many as one third in some of the studies above, serum sodium increases more than 5 mEq/L but does not reach values > 130 mEq/L. Therefore, vaptans are potentially effective in the short-term treatment of hypervolemic hyponatremia in patients with cirrhosis.


Fig. 14.3
Observed serum sodium concentration in patients with hyponatremia that received tolvaptan or placebo for 30 days and 7 days after stopping (day 37) Error bars are ± SE. *P < 0.001, tolvaptan versus placebo; † P < 0.01, tolvaptan versus placebo; ‡ P < 0.05, tolvaptan versus placebo. (From Ref. [37])
Overall, the vaptans do not seem to improve major outcomes in cirrhosis. A meta-analysis evaluated outcomes in 2266 patients from 12 randomized trials of tolvaptan, satavaptan, and lixivaptan. The primary outcome measure was mortality and secondary outcomes included, but were not limited to complications of cirrhosis and mobilization of ascites [39]. While the vaptans increased serum sodium, reduced mean body weight (mean difference of—1.82 kg) and increased time to first large volume paracentesis (RR = 0.76; 0.60–0.83), there was no mortality benefit (RR = 1.06; 0.90–1.26). There was a significant increase in thirst (RR = 3.97; 1.78–8.83) and excessive urine volume of > 5 L/day (RR = 9.96; 1.38–71.68). These adverse effects are important particularly in a patient population that is predisposed to encephalopathy limiting access to water and physical deconditioning limiting mobility.
Therefore, future of this class of medications for patients with cirrhosis is currently in question. First, the effect of vaptans on hyponatremia has only been proven in the short term and they may be associated with severe adverse events in the long term. Unfortunately, phase 3 long-term treatment studies in three different populations of patients with cirrhosis and ascites demonstrated a lack of effect [40]. Moreover, use of satavaptan was associated with increased mortality. Though the reason for this signal could not be determined, it was withdrawn from development. Finally, while, a small study in 18 patients with cirrhosis and ascites without hyponatremia showed that the administration of tolvaptan dose dependently decreased body weight and improved ascites and edema, long-term studies with tolvaptan in other populations were associated with liver injury [41, 42]. Indeed, the Tolvaptan Efficacy and Safety in Management of Autosomal Dominant Polycystic Kidney Disease and its Outcomes (TEMPO) 3:4 study evaluated the efficacy and safety of tolvaptan in a population with polycystic kidney disease and saw a 23 % rate of serious hepatic adverse events [41].
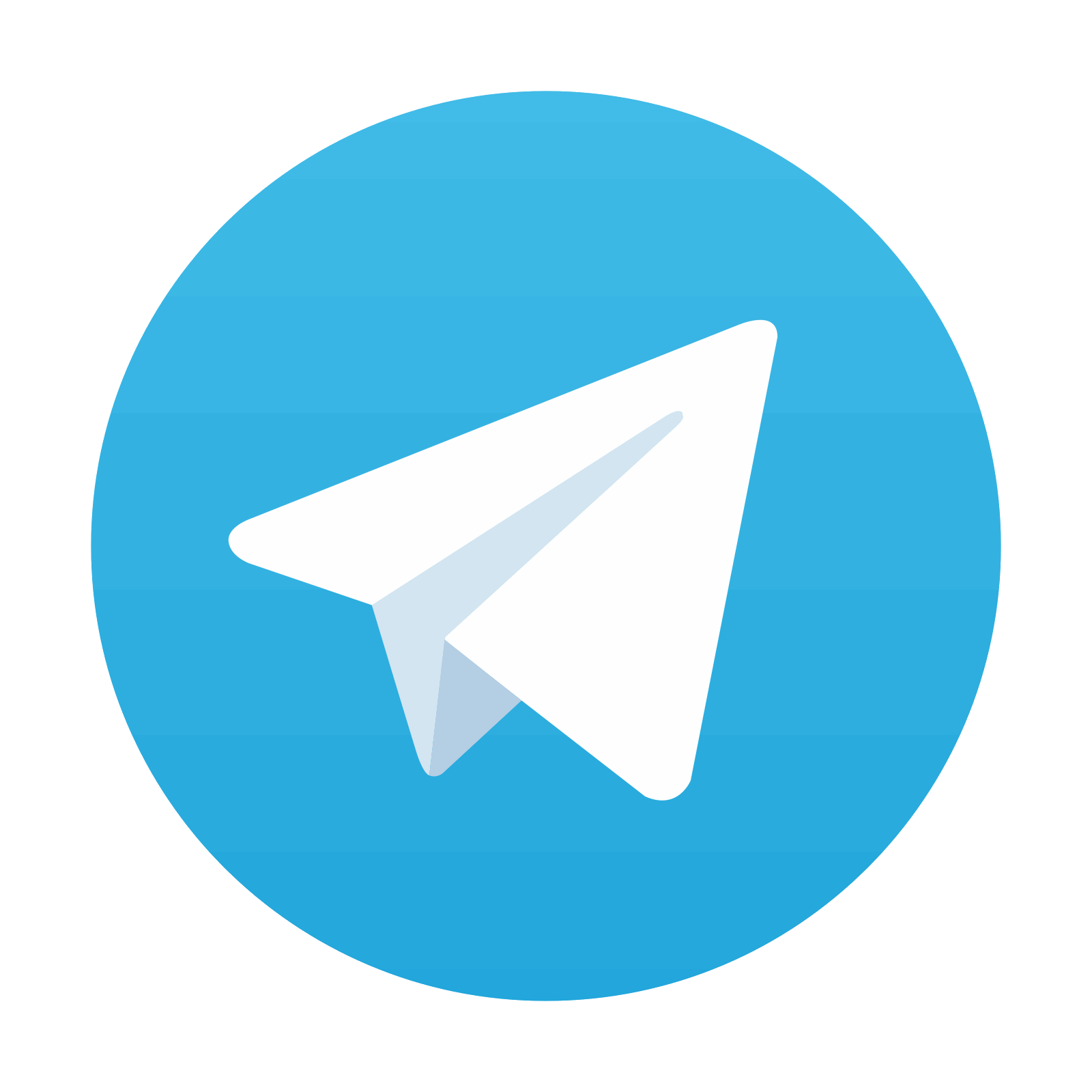
Stay updated, free articles. Join our Telegram channel

Full access? Get Clinical Tree
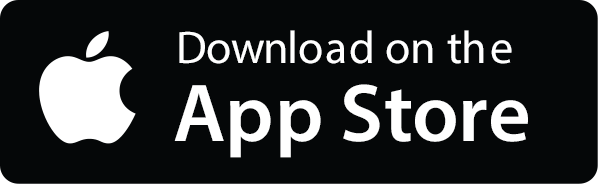
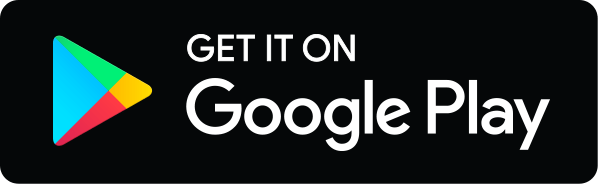