INTRODUCTION
HTN as defined by current standards afflicts almost 70 million Americans, and is thus—not surprisingly—the most common reason for a physician visit in this country. The magnitude of the problem has generated multiple public health efforts in the past 35 years, leading to the present levels of awareness (81%) and treatment (73%). Although still not optimal, the rates of BP control have improved from 27% to 50% in the last decade. Nevertheless, it is imperative that we focus continued attention on education of the public and medical professionals. The many reports of the Joint National Committee on Prevention, Detection, Evaluation and Treatment of High Blood Pressure are the most prominent representatives of this educational effort, and its eighth report (The Eighth Report of the Joint National Committee on Prevention, Detection, Evaluation, and Treatment of High Blood Pressure [JNC 8]) is forthcoming in 2013. We urge everyone interested in HTN to read the full report after its publication. This chapter reviews general aspects of HTN related to epidemiology, mechanisms, diagnostic evaluation, complications, and therapy.
EPIDEMIOLOGY
Current estimates of the worldwide prevalence of HTN are as high as 1 billion individuals (–68 million in the United States). Its prevalence increases with age (Table 20.1), and the BP rise is steeper in men than in premenopausal women. Women show a greater rise in BP following menopause, and the absolute prevalence of HTN is higher in women than men. HTN is a more pervasive problem in the Western world, and there is a relationship between average populational sodium intake and the prevalence of HTN. Other factors associated with a greater prevalence of HTN include ethnicity, lower socioeconomic status, lower dietary potassium intake, higher body mass index, and larger amounts of habitual alcohol use. The prevalence is greater in African Americans and nonblack Hispanics than in whites. These 2 subgroups also have poorer control rates than whites, which further amplify the cardiovascular burden of BP. Another important point is the fact that migration from a rural to an urban setting or from a nonindustrialized to an industrialized country increases the risk of HTN. These effects are primarily mediated by changes in dietary and psychosocial factors.
TABLE 20-1. Prevalence of Hypertension in U.S. Adults According to Age and Gender
KEY POINTS
PATHOPHYSIOLOGY
Essential HTN is the term used to describe elevated BP without a readily detectable cause. The term was coined at a time when high BP was thought to be required or “essential” to surmount the established vascular disease in order to achieve target–organ perfusion. In the past, vascular disease was thought to precede HTN, and not be a result of it. Therefore, most experts discouraged physicians from treating high BP. It was not until the 1960s that it became clear that HTN was itself a major risk factor for vascular disease, and that its treatment resulted in improved outcomes. Only then was it determined that the need for higher BPs was not really “essential.”
The operative mechanisms in essential HTN are multiple, intersecting, and represent an attempt at a balance between vasopressor and vasodilator mechanisms. The formula
BP = cardiac output × vascular resistance
provides a valuable guide to the understanding of the pathophysiology of HTN. Changes in cardiac output usually result only in transient changes in BP, therefore, most of the chronic changes in BP control are dependent on the relationship between 1 of the determinants of cardiac output—blood volume (BV) (the content)—and systemic vascular resistance (SVR, the container). For the sake of this discussion, BV is referred to here as a surrogate for extracellular volume (ECV), even though an increase in ECV does not always result in increased BV, and vice versa. Because the vasculature has a great ability to accommodate BV because of its large capacitance bed (veins and venules), an inappropriate increase in vascular tone is necessary to result in HTN when BV is increased. Therefore, abnormalities in vascular resistance, either as a net increase or an insufficient decrease, are an essential part of HTN in almost all patients. Figure 20.1 is an incomplete list of relevant mechanisms that impact on BP regulation and vascular function. These systems are affected to different degrees in different individuals. Discrepancies are the result of the genetic heterogeneity of the population, and different degrees of exposure to environmental factors (sodium and potassium intake, alcohol use, psychosocial stressors, and so on).
FIGURE 20-1. Relevant mechanisms involved in the genesis of hypertension. Abbreviations: RAS, renin-angiotensin system; SNS, sympathetic nervous system; VC, vasoconstrictor; VD, vasodilator.
It is estimated that heredity accounts for approximately 20% to 25% of one’s BP and the determinants of this effect are polygenic and highly variable. Certain gene polymorphisms affecting the function of certain key mechanisms, especially the renin-angiotensin-aldosterone system (RAAS) (eg, the angiotensinogen, angiotensin-converting enzyme [ACE], aldosterone synthase, and 11β-hydroxysteroid dehydrogenase genes) or salt sensitivity (eg, the α-adducin gene) have been linked with the presence of HTN, but the relative importance of these polymorphisms is small. Recent large genome-wide association studies have identified high-risk loci for the presence of HTN. Some of these involve known pathways related to BP regulation, but not all. Again, the overall effect of each of these loci is quite small (~1 mmHg for systolic blood pressure [SBP] per locus). Perhaps of greater relevance is the approach to monogenic disorders. Although rare, the understanding of the mechanisms related to HTN in these conditions leads to a better understanding of essential HTN in general. Examples of these disorders are listed in Table 20.2, and are discussed in greater detail in Chapter 21. The findings related to their different mechanisms indicate that single-gene mutations altering renal sodium handling are able to produce sustained, severe HTN.
TABLE 20-2. Causes of Monogenic Hypertension and Their Respective Pathophysiologic Mechanisms, All with a Common Link to Increase Sodium Reabsorption
The aforementioned genetic diseases lend support to a much older postulate related to the central role of the kidneys in the genesis of HTN. Multiple experimental and clinical models reveal that the development of HTN always depends on an abnormality in renal sodium handling. Even if the primary change is related to increased cardiac output or peripheral resistance, these abnormalities result only in transient increases in BP unless a change in the renal pressure–volume relationship occurs, which will result in the need for a higher BP to guarantee sodium balance.
Abnormalities in renal sodium handling commonly result in elevated BP through interactions that were first championed by Guyton (the Guyton hypothesis). In this now widely accepted hypothesis, the most relevant mechanism used by the body to regulate BP is to alter renal sodium handling, thereby controlling ECV and cardiac output. In the normal state, increased sodium intake causes an increase in ECV and BP. Because of a steep relationship between volume and pressure, small increases in BP produce natriuresis, which restores sodium balance and returns BP to normal. This response becomes abnormal whenever there is impediment to sodium excretion, such as in states of reduced renal function or high levels of angiotensin II. In such case, the BP rise necessary to restore sodium balance is greater, resulting in a state of increased sensitivity to dietary salt wherein the ability to excrete sodium becomes pressure-dependent. In this situation, sodium balance is only achieved at higher BP levels that are required to excrete the ingested sodium load, a process called pressure natriuresis (Figure 20.2 ). This chronic state of high BP generated by sodium retention is not related to increased BV, which is only minimally increased (if at all) in most hypertensive patients, but to sodium-related increases in SVR. The mechanisms underlying this vascular effect are not completely understood, but we know that sodium overload leads to increased sympathetic outflow and abnormalities in cation flux, especially calcium. Volume expansion decreases extracellular calcium and stimulates the production of parathyroid hormone (PTH), 1,25-dihydroxy vitamin D, and ouabain-like factors that lead to an intracellular calcium shift, increased intracellular calcium, and thus elevated vascular resistance. Thus, abnormalities in pressure–volume relationships lie at the center of essential HTN, and also occur as an important part of the maintenance phase of most other causes of HTN (such as hyperaldosteronism, renal artery stenosis, Cushing syndrome, coarctation of the aorta, and even pheochromocytoma).
FIGURE 20-2. The concept of pressure natriuresis. If appropriate renal and vascular mechanisms exist, BP increases minimally and the excess volume is excreted completely, rapidly returning BP to normal. If adaptation is not normal, sodium retention occurs resulting in substantially increased BP, which then induces a pressure-natriuresis in order to achieve sodium balance (at the cost of chronic HTN).
The current understanding of the interplay between renal sodium retention and HTN involves changes in sodium handling throughout the nephron. The inciting event is an increase in arteriolar tone in the renal vasculature (e.g., from increased activity of the RAAS or the sympathetic nervous system), subtle renal injury of any type, aging, or the effects of inherited or environmental factors that lead to a sodium retentive phenotype. An interesting theory with substantial experimental support proposes that this increased renal vasoconstriction leads to a preglomerular (afferent) arteriolopathy that results in impaired sodium filtration. In addition, renal vasoconstriction results in tubular ischemia, which in turn results in increased sodium avidity. The combined result of these processes is salt-sensitive hypertension. The sensitivity of an individual to salt/volume overload and the BP response observed with changes in sodium intake can be improved or corrected by modifying some of the factors that modulate salt sensitivity, especially the RAAS. Obviously, in any individual who has increased sensitivity to salt (30% to 50% of the hypertensive population), sodium restriction can decrease BP effectively.
The sympathetic nervous system is important in BP control, and its activation may be an important early step in the process of increased renovascular resistance (increased arteriolar tone) that leads to sodium retention. Multiple strategies are available to block sympathetic overactivity in HTN, both at the central level, to limit central nervous system (CNS) sympathetic outflow, and at the effector level, with direct α– or β-receptor antagonism. Decreased sympathetic activity can also be achieved clinically by direct electric stimulation of the carotid baroreceptors or by radiofrequency ablation of renal sympathetic afferents.
The balance between vasopressor and vasodilator mechanisms is difficult to interpret in any individual patient. Table 20.3 summarizes the humoral systems that can be abnormally increased or decreased in HTN. Their relative role in the pathogenesis of HTN varies substantially, and a detailed discussion is beyond the scope of this text. The vasculature is not only abnormal in its responses related to vascular tone, but also in its structure. Hypertensive subjects have diffuse capillary rarefaction, as well as a progressive decrease in the lumen of small arteries and arterioles. These structural changes limit organ perfusion (especially important in the kidney), and also impair vascular responses to vasodilatory substances.
TABLE 20-3. Humoral and Cellular Factors Related to Vascular Function in Hypertension
An important pathophysiologic mechanism is increased arterial stiffness, a problem that is particularly relevant to older individuals (and isolated systolic HTN [ISH]). Arterial stiffening is caused by loss of elastic fibers of large arteries, and is strongly associated with aging (especially after the sixth decade of life), smoking, diabetes mellitus, and kidney disease. As shown in Figure 20.3, this process leads to increased pulse wave velocity (PWV), which, in turn, results in faster reflection of the incident pulse wave. Faster reflection implies that the reflected wave returns to the heart before the end of systole, resulting in augmentation of central BP and increased SBP. This abnormality is relevant to left ventricular (LV) performance, as increased impedance to LV ejection is an important factor in generating LV hypertrophy (LVH) and subendocardial myocardial ischemia, 2 common complications of HTN. Abnormalities in arterial structure also alter the shape of decay of the diastolic BP (DBP) curve resulting in a decrease in DBP and wider pulse pressure.
FIGURE 20-3. Effect of age and arterial stiffening on systolic blood pressure. Aging is associated with increased arterial stiffness, which results in faster PWV and wave reflection. Faster wave reflection augments the reflected pulse wave, which returns to the central circulation before the end of systole, thus increasing SBP and decreasing the diastolic BP. The relative magnitude of this effect is greater in the central blood vessels (aorta).
KEY POINTS
PATHOPHYSIOLOGY OF THE CLINICAL CONSEQUENCES OF HYPERTENSION
HTN is marked by diffuse vascular injury. If left untreated, elevated BP results in cardiovascular complications in as many as 50% of patients. The risk is directly related to BP levels; in a metaanalysis that included more than 1 million patients, the risk of cardiovascular mortality doubled with each BP increase of 20/10 mmHg, starting with levels above 115/75 mmHg. Progressive damage affects several vascular territories, with a particular predilection for the cerebral vasculature, retinal vessels, coronary arteries, renal circulation, and arteries of the extremities. The heart is not only affected by way of coronary disease, but also from pressure overload that leads to LVH.
Cerebrovascular disease is a frequent complication of HTN. At any given age, the risk of developing a stroke is increased by the presence of HTN, and the magnitude of this risk is directly related to the degree of BP rise. Vessels supplying the basal ganglia, brainstem, and cerebellum are exposed to higher BP levels, and there is a large drop of BP over a short distance in these short resistance vessels. Thus, these vessels sustain most of the damage in HTN, which develop as arterial hyalinosis and/or microaneurysms of the perforating branches. Occlusion of hyalinized vessels results in the small lacunar infarcts due to focal ischemia, and rupture of microaneurysms leads to the classic hypertensive hemorrhagic strokes of any of these sites, particularly the basal ganglia (more than half of all hypertensive cerebral hemorrhages are putaminal). In the neocortex, longer arteries with many branches act as a stepdown transformer, protecting the cortex from more extensive HTN damage.
Damage to retinal vessels is extensive, and examination of these changes with an ophthalmoscope provides valuable information on the state of the microvasculature in HTN (see the section “Diagnostic Evaluation”). Although hypertensive retinopathy is an infrequent cause of visual problems, there is an increased risk of central retinal vein occlusion in HTN, and high BP accelerates the progression of other eye diseases, especially diabetic retinopathy.
Cardiac involvement in HTN is extensive and complex. On the one hand, HTN leads to accelerated coronary atherosclerosis, a process mediated by shear stress, oxidative stress, and the coexistence of the metabolic syndrome (obesity, insulin resistance with or without diabetes mellitus, dyslipidemia, and HTN). This leads to clinical coronary disease and loss of myocardial mass because of ischemia and infarction. Additionally, the pressure overload state results in concentric LVH, which is the most common clinically relevant targetorgan complication of HTN, and is associated with worse outcomes in HTN. LVH and changes in the shape of the diastolic decay of the central BP curve (see above) lead to relative subendocardial ischemia, amplifying the effects induced by atherosclerotic changes. Long-term pressure overload and LVH are maladaptive, and chamber dilation and systolic dysfunction ultimately result, especially in patients with associated coronary disease and myocardial infarction. This course is responsible for the increased occurrence of congestive heart failure in HTN.
The kidneys are commonly affected by untreated HTN. Hypertensive nephrosclerosis is the result of progressive parenchymal ischemia as a consequence of narrowing and hyaline sclerosis of arterioles and small arteries. In addition, the larger interlobular arteries develop marked thickening of the media from a reduplication of the elastic lamina (fibroelastic hyperplasia). This abnormality also results in areas of parenchymal ischemia and interstitial fibrosis. Nephrosclerosis causes a decline of glomerular filtration rate in as many as 5% of patients with HTN, and is most common in patients with long-standing uncontrolled BP, especially in African Americans.
Atherosclerosis of the peripheral vasculature is accelerated by HTN, though other factors seem more relevant, such as smoking, diabetes, and hyperlipidemia. Nevertheless, HTN is a participant in the development of atherosclerotic plaques and its control is associated with small decreases in the incidence of peripheral arterial disease.
In patients who develop “malignant phase HTN,” a process in which BP is very high and there is evidence of target-organ dysfunction, diffuse endothelial damage leads to a microangiopathic picture (intravascular hemolysis, consumptive thrombocytopenia) and acute loss of renal function. Endothelial damage is caused by shear trauma as well as toxicity induced by the RAAS (angiotensin II is a major pathogenetic factor). Histologically, there is extensive arteriolar damage and occlusion, a process named arteriolar fibrinoid necrosis.
KEY POINTS
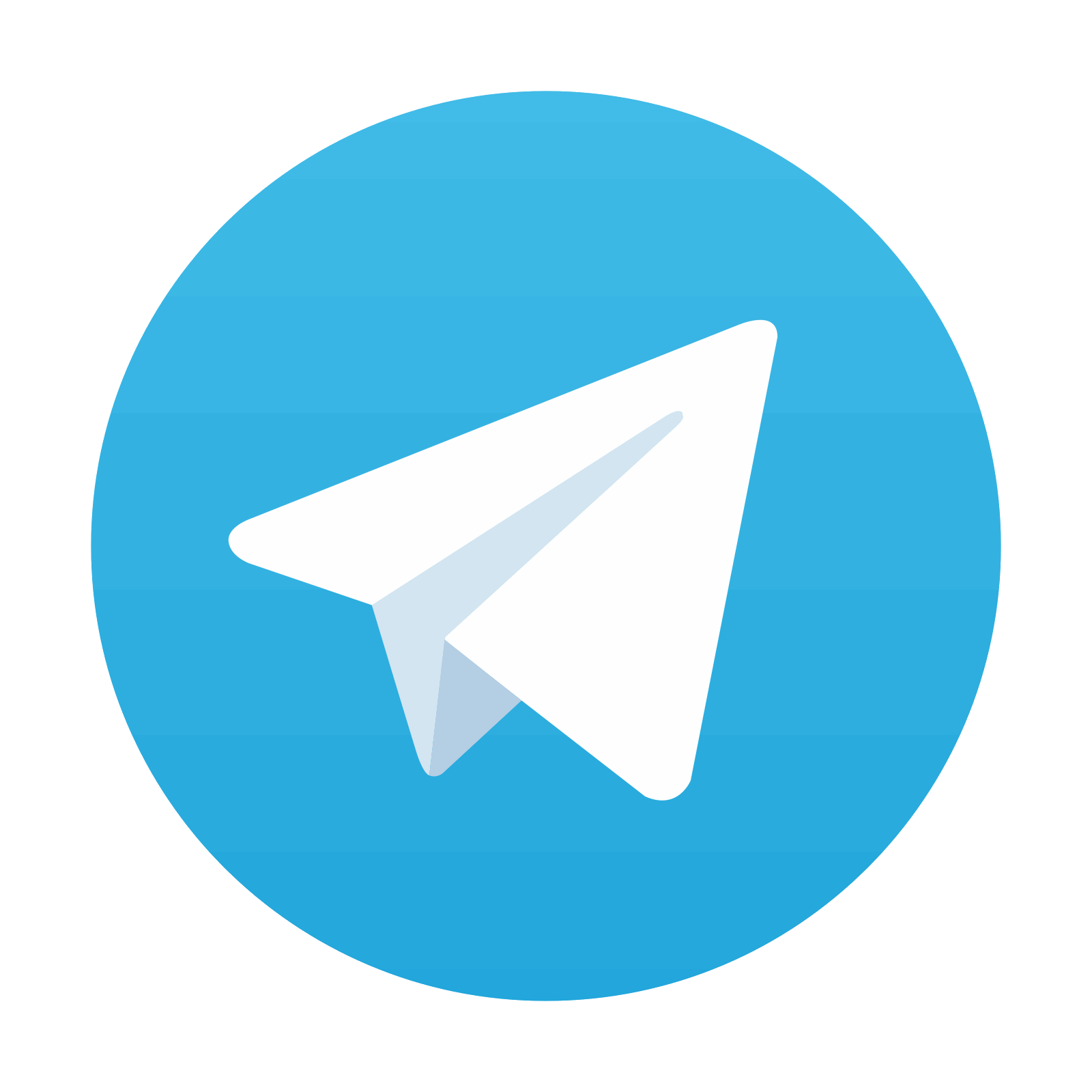
Stay updated, free articles. Join our Telegram channel

Full access? Get Clinical Tree
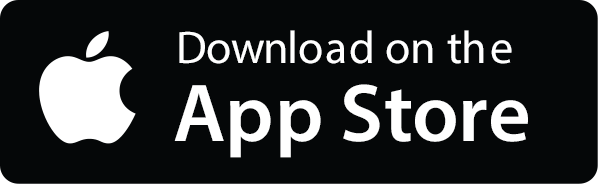
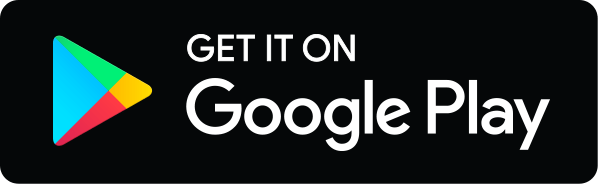