Fig. 3.1
Anatomical and functional regions of the stomach . The anatomical division of the stomach consists of the fundus, antrum, and corpus (body) that reflects differences in motor function. Functionally speaking, the stomach has two major regions: an exocrine or glandular portion that consists of the fundus and body or acid-secreting area, and an endocrine portion that is located in the antrum or gastrin -secreting area
Acid-Secreting (Exocrine) Area
The mucosa of the exocrine portion of the stomach consists of simple columnar epithelial cells that line the luminal surface. These cells secrete mucus and an alkaline fluid, both necessary for protecting the stomach against its own potentially harmful juices. Opening into the mucosal surface are numerous gastric pits that serve as conduits for the secretions of 3–7 oxyntic gastric glands (Fig. 3.2a) into the gastric lumen. The area occupied by the gastric pits is at least 50 % of the total luminal surface area. As shown in Fig. 3.2b, the remainder of the gastric or oxyntic gland can be further divided into two regions. The neck of the oxyntic gland contains parietal and mucous neck cells, the latter resembling intestinal goblet cells and secreting mucus. The neck region is also the site of germinal cell proliferation and differentiation, giving rise to surface cells that migrate up to the surface or glandular cells that move downward toward the base. Cells progressively mature as they reach their final destinations and then are continuously replaced by new cells, the turnover of various cell types ranging from days to weeks. The base of the gland contains chief cells in addition to some parietal and mucous neck cells. The oxyntic gland also contains a number of endocrine-type cells dispersed among chief and parietal cells that play a role regulating their secretory functions.
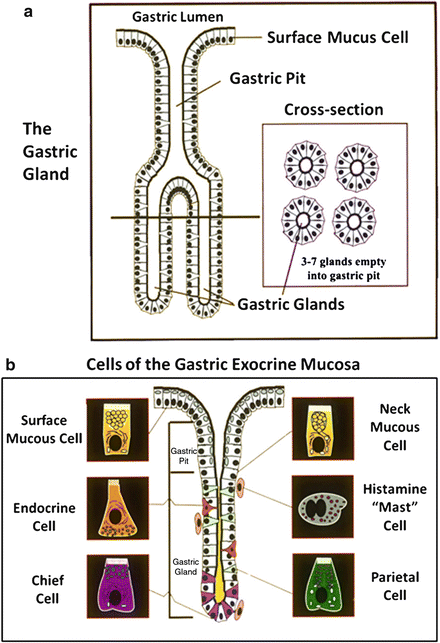
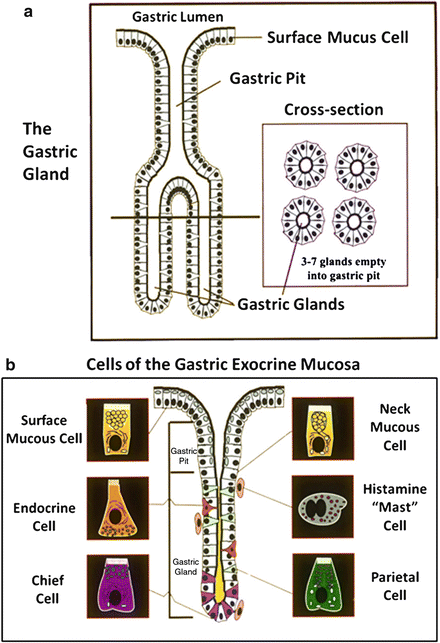
Fig. 3.2
The structure of gastric gland. (a) Side and cross-sectional views of gastric pits. (b) Various types of cells that are found in the gastric exocrine mucosa
Each cell type has different functions. Parietal cells are the acid-secreting cells, and their cellular physiology will be covered in greater detail later in the chapter. Chief cells make and secrete pepsinogen , which is converted by gastric acid to the active form pepsin . Mucous cells make mucus, an essential component that lubricates and protects the gastric mucosa , and those at surface and in gastric pits also secrete bicarbonate. Interspersed among these cells of the oxyntic gland are enterochromaffin-like (ECL) cells that regulate their functions by secretion of bioactive amines and peptides. Finally, a number of mast-like cells found in close apposition to epithelial cells of the gastric gland secrete histamine . In humans, they are believed to be important physiological regulators of gastric acid and pepsin secretion, an effect mediated through a paracrine action.
Hormone-Secreting (Endocrine) Area
The antral-pyloric or hormone-secreting area contains mucus-secreting cells similar to those seen in oxyntic glands, as well as a limited number of parietal and pepsinogen -secreting cells. However, this region of the stomach is the major source of gastrin secreted by the endocrine G cells of the mucosa . These cells are pyramidal in shape and have a narrow apical surface with long microvilli (Fig. 3.3). Secretory granules are located at the base of the cell, and the release of gastrin occurs by fusion and exocytosis with the basolateral membrane. Endocrine D cells , also found in the antral-pyloric region, elaborate somatostatin , an important physiological regulator of gastrin release and gastric acid secretion. D cells appear to have dendritic-like extensions of their cell membranes that span to adjacent G cells, consistent with a paracrine regulation of mucosal function. These processes make the delivery of somatostatin to G cells rapid and highly selective. At least seven additional types of enteroendocrine cells can be found in the gastric mucosa; however, their physiological role in the regulation of gastric function is not as well-defined.
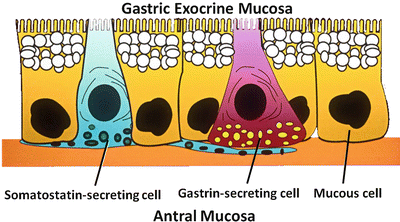
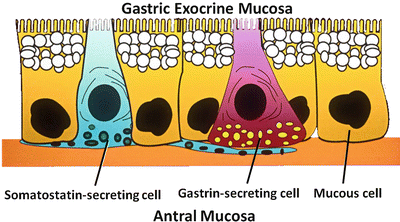
Fig. 3.3
Cells of the gastric endocrine antral mucosa . The two major endocrine cells of the antral mucosa are somatostatin -secreting D cells and gastrin -secreting G cells . The D cells are believed to have dendritic-like extensions of their cell membranes that span to adjacent G cells, which are consistent with the paracrine regulation of mucosal function
2 Gastric Acid Secretion
2.1 Neural and Hormonal Regulation of Gastric Acid Secretion
Acid secretion is regulated by nerves and GI hormones. Although the understanding of their mechanisms of actions is still incomplete, accumulated evidence has been learned about the regulation and cell biology of acid secretion to have had a major impact on the treatment of patients with peptic ulcer disease .
There are three major stimulators of acid secretion: (1) gastrin (predominantly secreted by antral G cells ), (2) histamine (in humans probably arising from mast-like cells in the lamina propria subjacent to the gastric epithelium), and (3) acetylcholine (secreted from postsynaptic vagal fibers innervating the gastric mucosa ). On the other hand Somatostatin , secreted from antral and oxyntic gland D cells as well as pancreatic islet cells , and prostaglandins from mucosal cells, are the major paracrine inhibitors of gastric acid secretion.
The interaction of these regulators is extremely complex, and several issues remain controversial. For instance, it is still not clear at what level certain agents regulate acid secretion. Specific receptors on parietal cells for histamine , gastrin , and acetylcholine have been demonstrated, and their stimulation results in acid secretion (Fig. 3.4). Histamine stimulates H 2 -type receptors and activates adenylate cyclase to increase cellular content of cyclic adenosine monophosphate (cAMP). The increase in cAMP, in turn, activates cAMP-dependent protein kinase, which appears to be an important mediator in initiating acid secretion. However, there is evidence that histamine may also increase cytosolic Ca2+ concentrations, through coupling with another signal transduction pathway such as phospholipase C (mediating phosphatidylinositol metabolism). Gastrin and acetylcholine appear to activate the phosphatidylinositol (PI) pathway, gastrin through the stimulation of a specific gastrin receptor (CCK B ) and acetylcholine through activation of a M 3 -type muscarinic receptor. These agonists both increase intracellular free calcium concentrations. However, there are some differences in the activation pathways. Acid secretion stimulated by acetylcholine is dependent on the presence of extracellular calcium, whereas the effects of gastrin are only partially affected by this manipulation. Thus, the cellular mechanisms mediating these responses are clearly more diverse and complex than previously thought. The physiological role of protein kinase C , which is activated by the generation of diacylglycerol from phosphatidylinositol metabolism, is unclear. Some evidence suggests that it has a minor role in stimulation of acid secretion and may be more important in feedback inhibition of parietal cell function. Finally, cAMP and calcium may interact in a variety of ways to modulate cell activation. For example, potentiation can be seen between agents that increase cytosolic calcium (gastrin and acetylcholine) and cAMP (histamine), an observation that may have considerable clinical and therapeutic relevance. The nature of this interaction remains undetermined.
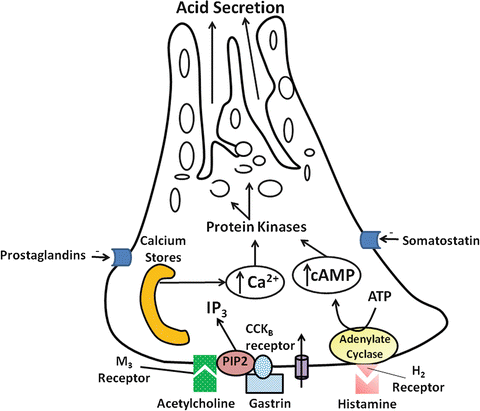
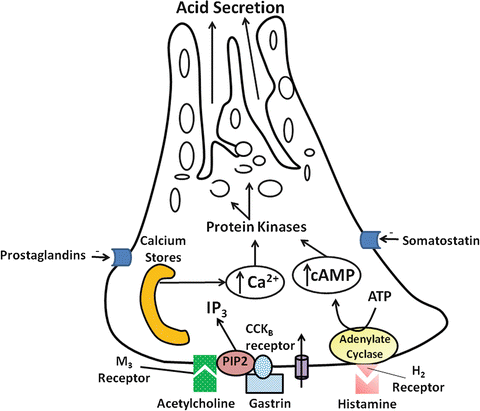
Fig. 3.4
Cellular mechanism of acid secretion by the parietal cell. There are three basic acid stimulants involved in the regulation of acid secretion, i.e. the neurocrine, acetylcholine ; the endocrine, gastrin ; the paracrine, histamine . PIP 2 phosphatidyl-4,5-bisphosphate, IP 3 inositol 1,4,5-triphosphate
In addition to their direct effects on parietal cell function, these agents appear to affect other target cells involved in the regulation of acid secretion (Fig. 3.5). For example, gastrin from antral G cells and acetylcholine from cholinergic neurons stimulate specific receptors on mast or ECL cells, promoting the secretion of histamine . Histamine potentiates the effects of each of these agents on parietal cell secretion. Noradrenergic nerves inhibit histamine release from mast cells through activation of β receptors, the net effect being the inhibition of acid secretion.
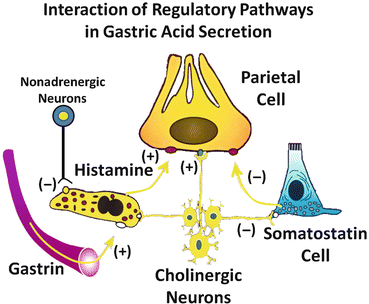
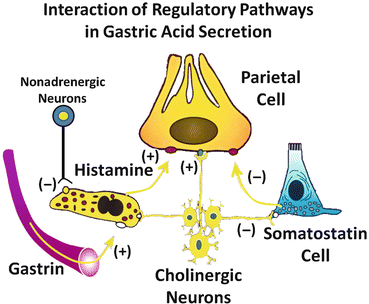
Fig. 3.5
Interaction of regulatory pathways in gastric acid secretion. Apart from the direct effects of the three basic acid stimulants of histamine, gastrin and acetylcholine on parietal cell functions, various regulatory agents also affect other target cells for modulating acid secretion such as the inhibitory action of somatostatin
The role of somatostatin -secreting D cells in both the antral and acid-secreting regions of the stomach also appears to be a major component of the regulatory systems. In the antrum, somatostatin inhibits G-cell secretion of gastrin and is released by the presence of luminal acid and gastrin (serving as negative feedback mechanisms) and by stimulation from enteric neurons containing acetylcholine , vasoactive intestinal peptide (VIP), and gastrin-releasing peptide (GRP). In the exocrine portion of the stomach, D-cell release of somatostatin directly inhibits parietal cell acid secretion. Alternatively, somatostatin also inhibits acid secretion by decreasing histamine release by mast-like and ECL cells. Gastrin and cholecystokinin (CCK) stimulate somatostatin secretion, whereas cholinergic neurons inhibit it.
The relative contribution of each of the aforementioned pathways in regulating gastric acid secretion is highly variable among mammalian species and not entirely elucidated in humans. However, it does appear that histamine plays a major role in stimulating gastric acid secretion. Specific inhibitors of the histamine H2 receptor, such as cimetidine and ranitidine, are clinically and experimentally very effective in reducing acid secretion, in spite of the fact that the stimulatory effects of gastrin and acetylcholine are still present. How can this be accounted for? A possible explanation is that in the absence of histamine stimulation of parietal cells, there is no potentiation of the gastrin or acetylcholine effects, thus diminishing acid secretion. In addition, gastrin stimulation of somatostatin release would continue unabated, further rendering a decrease in acid secretion.
2.2 Cellular Mechanisms Mediating Gastric Acid Secretion
Cellular Events
When the parietal cell is stimulated, it undergoes rapid, dramatic morphologic changes that are now known to be important in setting up the acid-secretory apparatus. The resting, or non-secreting, parietal cell has a specialized network of narrow channels that extend from the luminal surface through much of the cell body (Fig. 3.6a). These are secretory canaliculi , lined by short, stubby microvilli. There is also an elaborate system of tubular and vesicular membranes (tubulovesicles) in the luminal aspect of the cell. Large mitochondria, characteristically seen in these cells, are essential for providing a high oxidative capacity during acid secretion.
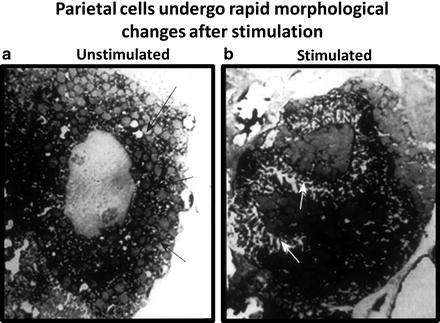
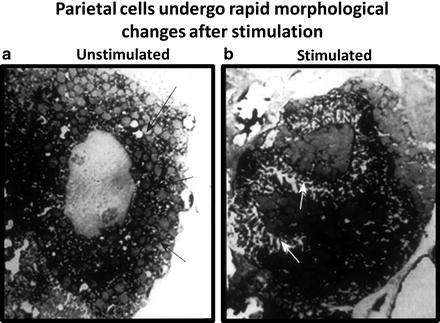
Fig. 3.6
Morphological changes associated with parietal cell activation and secretion. (a) The resting, or nonsecreting, parietal cell has many mitochondria and an elaborate system of tubular and vesicular membranes near the luminal aspect of the cell. (b) With stimulation, the luminal surface area increases six to tenfold, with a concomitant disappearance of tubulovesicle membranes. These changes are caused by the fusion of the tubulovesicle membranes with the plasma membrane, increasing the number and density of proton pumps in the apical surface (Courtesy of Dr. George Sachs)
Within a few minutes of stimulation, microvilli lining the canaliculi become longer and more elaborate. It is estimated that the luminal surface area increases to 6–10 times that of the resting state (Fig. 3.6b). Concomitant with these changes is the disappearance of tubulovesicle membranes. Withdrawal of the stimulating agents leads to a collapse of the canaliculi spaces and a return of the tubulovesicle forms in the cytoplasm. These observations are consistent with the membrane recycling that occurs during the stimulated and resting states of the parietal cell. Tubulovesicles contain large amounts of the gastric proton-pump enzyme H + /K + -ATPase . Following stimulation of the parietal cell, tubulovesicle membranes fuse with the plasma membrane, increasing the number and density of proton pumps in the apical surface. Conversely, withdrawal of the stimulus leads to endocytosis of these membranes, a decrease in the density of proton pumps in the apical membrane, and decreased acid secretion. The proton pump is only active when inserted in the luminal membrane; it is inactive when re-sequestered into tubulovesicle membranes.
Electrolyte Transport
The mechanism for proton secretion into the lumen is fairly well understood and summarized in Fig. 3.7. The essential element here is a membrane-bound enzyme that actively extrudes protons into the lumen in exchange for potassium ions. This enzyme requires adenosine triphosphate (ATP) and hence is referred to as the H + /K + -ATPase , or proton pump. Gastric H+/K+-ATPase is a member of the phosphorylating, ion-motive ATPase gene family, which includes Na+/K+-ATPase and Ca2+-ATPase. It is a heterodimer consisting of a larger catalytic subunit (α) and a smaller (β) glycosylated subunit. The role of the β subunit is unknown, but it appears to be required for the proton to work as a functional unit. The proton pump is electrically neural; that is, there is a one-for-one exchange of H+ and K+, with no net transfer of charge. Essential to the proton pump and acid secretion is the presence of an exit pathway for K+, which is then recycled in exchange for H+. This occurs through the coupled transport of K+ and Cl− out of the cell via respective conductive channels. The Cl− channel is distinct from the cystic fibrosis transmembrane regulator (CFTR) channel . These exit pathways operate in tandem with the proton pump, the net effect being the rapid secretion of hydrochloride into the canalicular lumen. The K + and Cl − exit pathways may be the major rate-determining factors in acid secretion, as both are regulated by cAMP-dependent protein kinase. In addition, being voltage-gated channels, they are active only when inserted into the luminal membrane where a favorable membrane potential exists. To date, there is no evidence that supports direct kinase regulation of the proton pump.
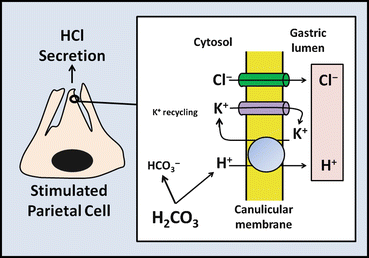
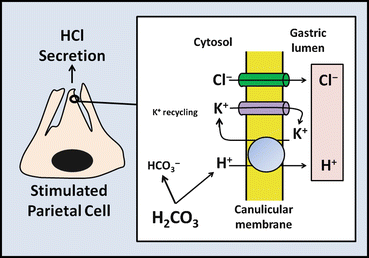
Fig. 3.7
Electrolyte transport and acid secretion at the luminal membrane of a stimulated parietal cell. The major component of acid secretion is the H+/K+-ATPase, or proton pump, which actively exchanges H+ for K+. For vectorial H+ secretion to occur, conductance pathways for K+ and Cl− must exit for recycling of K+ ions and extrusion of Cl−, respectively
The proton pump is somewhat unique in its actions. It has an enormous active secretory capacity, capable of secreting protons against large electrochemical gradients. As a consequence, luminal pH can approach the 1–2 range, representing a 5–6 log unit difference from intracellular pH values of approximately 7. No other part of the body can approach this level of acidification. Recently, several highly specific and clinically useful inhibitors of the proton pimp have been developed, specifically substituted-benzimidazole compounds such as omeprazole and lansoprazole . Being weak acids with pKa values of approximately 4, these agents are selectively (if not exclusively) taken up and concentrated in the acidified secretory canaliculi of gastric parietal cells. In this acid environment, they are converted to sulfonamides that are reactive with cysteine SH-thiol, but not cysteine disulfides. Thus, these agents bind to specific sites in the extracellular (luminal) domain of the α subunit of the H+/K+-ATPase . As a result, ATPase activity and proton transport cease. Because proton pump inhibitors block the final pathway, acid secretion can be virtually shut down, and patients may become a state, called achlorhydria . Omerprazole has therefore become a major part of the medical armamentarium for treating peptic ulcer diseases.
Because of the voluminous secretion of protons at the apical surface, equivalent amounts of base must be delivered by the cell into blood plasma to maintain normal intracellular pH. This is accomplished through a Cl − /HCO 3 − exchange mechanism located in the basolateral membrane of parietal cells. This pathway serves a dual purpose because it provides an entry pathway for chloride ions that are then vectorially secreted with protons. Coupled with Cl−/HCO3 − exchange is Na + /H + exchanger , which is important for providing intracellular sodium for recycling out of the cells via the sodium pump. This allows the sodium pump to operate efficiently at high capacity to maintain the electrochemical gradients required for proton secretion and to provide an entry pathway for potassium ions (since gastric juices typically contain potassium concentrations that exceed plasma potassium concentrations by twofold or more).
< div class='tao-gold-member'>
Only gold members can continue reading. Log In or Register a > to continue
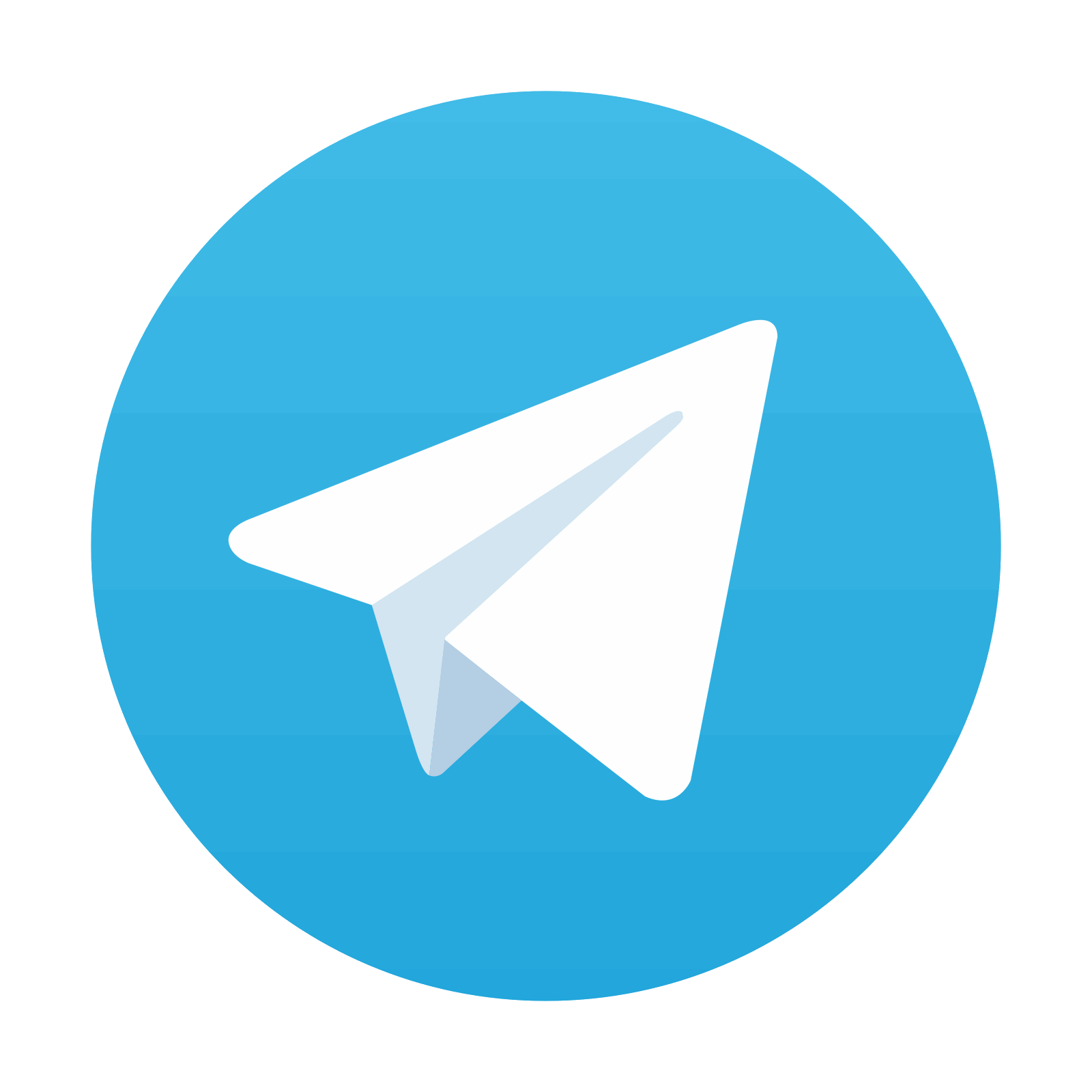
Stay updated, free articles. Join our Telegram channel

Full access? Get Clinical Tree
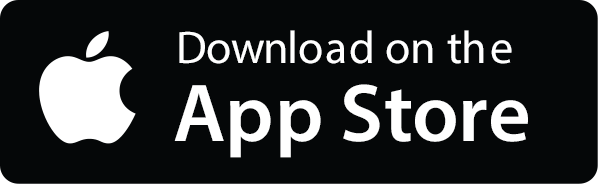
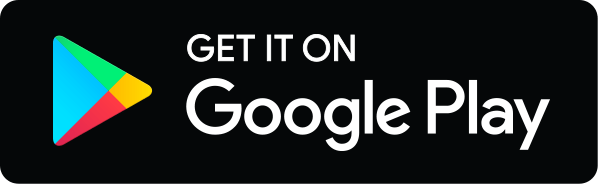