Chapter 7 Sumeet K. Asrani1 and Patrick S. Kamath2 1Baylor University Medical Center Dallas Texas USA 2Division of Gastroenterology and Hepatology, Mayo Clinic College of Medicine Rochester MN, USA Liver-related mortality is the eighth leading cause of death in the United States and is responsible for a significant proportion of premature mortality; among persons aged 45–64 years, it is the third leading cause of death [1]. Unfortunately, the overall prognosis of patients with cirrhosis remains poor in the recent era. Patients with cirrhosis can have compensated disease for several years or have evidence of decompensation, manifested by complications of portal hypertension. Compared to the general population, persons with compensated cirrhosis have a fivefold (hazard ratio, HR, 4.7; 95% confidence interval, CI, 4.4–5.0) and persons with decompensated cirrhosis have a 10-fold (HR 9.7; 95% CI 8.9–10.6) increased risk of death [2]. However, there is wide variability in rates of morbidity and mortality among patients with cirrhosis. Early identification of factors that portend poor outcomes is important to initiate relevant measures early on in the disease course. This chapter addresses the natural history of cirrhosis, discusses prognosis, and evaluates several models that identify cirrhotic patients at highest risk for poor outcomes. The natural history of cirrhosis has been well described. Mortality estimates vary based on the setting (inpatient, intensive care unit, community), stage (compensated or decompensated), and etiology of disease. A large systemic review of heterogeneous prognostic studies showed that the median survival for cirrhotic patients was 2.8 years. Median survival was approximately 12 years and 2 years for persons with compensated and decompensated cirrhosis, respectively [3]. The 1-year cumulative survival was 78% (95% for compensated cirrhosis and 61% for decompensated cirrhosis). As expected, lower survival was seen in hospital-based studies. Using data from a Danish nationwide population-based hospital registry, subjects diagnosed with cirrhosis (1995–2006) were identified. The overall 1-year survival probability was 66% after 1 year, 38% after 5 years, and 22% after 10 years [4]. The majority of the deaths were cirrhosis-related rather than due to the presence of medical comorbidities. In another population-based study, the overall survival for persons with compensated cirrhosis was 87% (versus 75%) at 1 year and 67% (versus 45%) at 5 years [2]. Most of the deaths among subjects with compensated cirrhosis occurred due to a transition to a decompensated state and subsequent consequences of the complications. To refine prognosis of persons with cirrhosis, four stages of cirrhosis have been proposed, with stages 1 and 2 representing compensated cirrhosis [3]. Stage 1 is characterized by absence of ascites or varices. Stage 2 is characterized by the presence of nonbleeding varices but absence of ascites. Stage 3 is characterized by ascites with or without esophageal varices in a patient that has never bled. Stage 4 is characterized by variceal bleeding with or without ascites. Based on this initial classification, in a large systematic review the 1-year survival was 99%, 96.6%, 80%, and 43%, respectively. However, recent studies have not shown a markedly higher mortality with stage 4 than stage 3. Better management of variceal bleeding may have potentially led to improved survival in patients with variceal bleed and relatively lower survival in patients with ascites regardless of their varices status. Development of hepatic encephalopathy (HE) is an emerging negative prognostic indicator. Among alcoholics studied in the Danish National Health registry (1993–2005), the median survival in patients without complications was 48 months. The 1-year survival rate was 83% (absence of complications), 80% (variceal bleeding), 71% (ascites), 51% (ascites and variceal bleeding), and 36% (HE, alone or in combination) [5]. Infection and renal failure are common causes of mortality in patients with cirrhosis. Compared with uninfected patients, cirrhotic patients with infections have almost a fourfold increase in mortality (odds ratio, OR, 3.8; 95% CI 2.1–4.2). Infections may be spontaneous or related to complications of portal hypertension such as gastrointestinal bleeding or ascites. In a recent meta-analysis, the overall median mortality of infected patients was 38%: 30.3% at 1 month and 63% at 12 months (1-year survival 37%). In a subset of studies with spontaneous bacterial peritonitis (SBP), the median mortality was 44% (1-year survival was 34%) [6]. Renal failure is also common in patients with cirrhosis awaiting liver transplant and is often multifactorial [7,8]. When compared with patients without renal failure, cirrhotic patients with renal dysfunction have a significantly increased risk of death (OR 7.6; 95% CI 5.4–10.8) [9]. Among patients with cirrhosis, the median mortality rate for those with renal failure is 58% at 1 month and 63% at 1 year [9]. Hence, given that the median survival at 1 year is 37% with either renal dysfunction and/or infection (and refractory ascites), presence of one of these factors may represent a more advanced stage in addition to the four stages proposed above. Given that the majority of deaths in patients with compensated cirrhosis is due to progression to a decompensated state and the development of its ensuing complications, knowledge of the risk of progression is important. The 10-year probability of risk of decompensation from a compensated state in a single-center study (1968–1980, median follow-up 63 months) was 58% [10]. Progression of cirrhosis from a compensated state (D’Amico stage 1 and 2) to a decompensated state (stage 3 and 4) has been examined in population-based studies. In an analysis of a large population-based United Kingdom General Practice Research Database (1987–2002), the age and gender adjusted rate of decompensation from compensated state was 12% per year [11]. This rate was faster during the first year after diagnosis (31%) and 5–7% in subsequent years. Other studies suggest a uniform rate of progression of 6–9% per year [3]. Yearly rates of decompensation likely vary by underlying disease with reported annual progression rates of 4% for viral hepatitis C, 6–10% for alcoholic cirrhosis with higher rates in patients with active alcohol use or development of alcoholic hepatitis, and 10% in persons with hepatitis B [12]. Besides assigning prognosis by clinical stage of disease, several models have been proposed to assist further in prognostication. This includes models to predict mortality or capture transition from a compensated to decompensated state. The Child’s score was initially designed to predict the outcome after surgery for portal hypertension (portocaval shunting and transection of the esophagus) in cirrhotic patients (Table 7.1). Based on the variables shown in Table 7.1, cirrhotic patients are stratified into three categories: Child–Pugh grades A (5–6 points), B (7–9 points), or C (10–15 points). The factors, in addition to an evaluation of liver dysfunction, are also a gauge of organ dysfunction [13]. The median 1-year survival is 95% for Child A, 80% for Child B, and 45% for Child C cirrhosis. Correspondingly, the 2-year survival is 90%, 70%, and 38%, respectively [3,14]. Child–Pugh score is an independent predictor of mortality in compensated or decompensated liver disease (e.g., ascites, bleeding esophageal varices) and within etiologies of liver disease (e.g., chronic hepatitis C, cholestatic liver disease, or alcoholic liver disease). Table 7.1 Prognostic scores in assessment of patients with cirrhosis. Several limitations of the Child–Pugh score are noted. This includes equal weight assigned to all components with lack of statistical validity (e.g., ascites and coagulopathy get the same numerical weight), categorization of continuous variable, subjective nature of assessment for some of the variables (ascites, encephalopathy), possible redundancy (inclusion both international normalized ratio, INR, and albumin), influence of interlaboratory variability (e.g., prothrombin time, albumin), empiric cutoff values for variables, ceiling effect of variables (bilirubin 5 mg/dL and 25 mg/dL get the same points), lack of inclusion of cause of liver disease, and inability to account for reversibility of treating the underlying cause (e.g., cessation of alcohol abuse). The model for end-stage liver disease (MELD) score, akin to the Child–Pugh score, was devised as an objective predictor of early mortality in patients undergoing elective placement of transjugular intrahepatic portosystemic shunts (TIPS) [15]. The model contains three objective variables: serum creatinine, bilirubin, and INR (Table 7.1). The MELD score has high concordance (c-statistic 0.83) to predict risk of death within 3 months. This implies that 83 out of a 100 times, in a pair of randomly drawn patients, the model correctly predicts which patient will die first [16]. The model has been validated in several independent cohorts of patients with varying levels of liver disease severity (e.g., hospitalized and ambulatory patients), as well as patients of geographically and temporally diverse origin [17]. It is a good predictor of long-term survival (e.g., c-statistic 0.75 for 1-year survival), across several different populations (e.g., c-statistic 0.83 for alcoholic hepatitis, acute liver failure) as well as a good prognostic marker in decompensated liver disease (e.g., variceal bleeding, infections), and surgery [18–22]. The etiology of liver disease and individual complications of portal hypertension do not significantly enhance the prognostic information captured by the MELD score [16,17]. Overall, the MELD score is used a common metric of underlying disease severity [16]. The benefits of the MELD score lie in its simplicity, use of a continuous scale, and incorporation of easily available objective laboratory parameters. It has been shown to be superior to clinical judgment in identifying mortality risk [23]. Variables are ranked based on their importance and no ceiling effect is observed [24]. There are obvious limitations. The MELD score was created in a select population without acute reversible causes of decompensation (e.g., infection). Further, it does not capture prognosis in certain conditions (e.g., portopulmonary hypertension) or certain complications (e.g., hepatocellular carcinoma, HCC) [25]. The total bilirubin rather than direct bilirubin is used. However, the former is not a superior predictor than the latter (unpublished data). Serum creatinine is highly weighted in patients with liver disease. However, it is known that inclusion of serum creatinine is suboptimal among cirrhotic patients [26–28]. Measured glomerular filtration rate (GFR, e.g., by iothalamate clearance measurement) is better at assessing prognosis [26,27]. A multivariable model that incorporates calculated GFR and/or serum sodium is superior to the MELD score [28]. Serum creatinine measured is not standardized and may be overestimated in patients with high serum bilirubin [29]. Serum creatinine may underestimate the severity of renal dysfunction among women as a function of less muscle mass [30]. The prothrombin time, as a reflection of synthetic dysfunction, may be manipulated by persons who have artificially higher INR when on warfarin-based anticoagulation [31,32]. There is interlaboratory variation in assays that measure INR based on measurement techniques [33,34]. Though a liver-specific INR is more accurate, practical implementation across centers may be hard to achieve [32,35]. Several models have been proposed to refine and improve the prognostic ability of the MELD score. The primary driving force has been estimating the prognosis of patients listed for liver transplantation and an assessment of their short-term mortality. This includes measurement of serial MELD scores, addition of variables (e.g., serum sodium), or reweighting components of the MELD score. A change in MELD scores, for example in patients with decompensated liver disease, may be associated with worse outcomes than other subjects with stable MELD score. However, the change in MELD (delta MELD) is not a significant predictor in multivariable analysis after adjusting for baseline MELD, which by definition is inherently higher, and repeated measurements of MELD score, which are more frequent in sicker patients [36,37]. Serum sodium concentration, a reflection of more advanced liver disease, is as an important prognostic factor [38–41]. Hyponatremia is associated with neurologic dysfunction, refractory ascites, hepatorenal syndrome, and death from liver disease [8,42]. Hyponatremia is an independent predictor of survival at 3 and 12 months [43,44]. Further, patients with hyponatremia are more likely to drop out while awaiting liver transplantation [45]. Hyponatremia is likely a marker for advanced renal dysfunction that is not accurately captured by creatinine-based measures. Even after adjusting for the MELD scores, a 1 mmol/L decrease in serum sodium concentration (125–140 mmol/L) is associated with a 5–10% increase in mortality [43,44]. This is particularly evident in patients with a low MELD score (Table 7.1). Serum sodium assessment is widely measured objective and easily available. Limitations of the serum sodium include potential for manipulation. Recently, attempts have been made to reweigh the components of the MELD score using a contemporary dataset [46,47] which improves the predictive ability of MELD based score to (c-statistic >0.85). Further validation is awaited (see Table 7.1) As mentioned above, the role of INR in the MELD score is contentious. Heuman et al. [48] examined a model without INR (MELD-XI) for liver transplant candidates on stable oral anticoagulation. The model was still less accurate than MELD, suggesting that even in those patients, INR somehow carries prognostic information. The INR can be elevated in patients receiving warfarin. Hence, an elevated INR may not accurately capture disease severity, especially because calibration for deriving INR is based on samples from persons on oral anticoagulation [33,34]. Less variability in INR is observed if calibration is done using samples from persons with liver disease (ISIliver). Though incorporation of ISIliver may improve MELD-based prediction models, it is practically cumbersome to incorporate [35]. But, despite the valid concerns, derivation of the MELD score using updated data suggests that the INR in its current form continues to be an excellent predictor of survival with a c-statistic upwards of 0.85 [47]. The incremental improvement in the predictive ability of a model that incorporates ISIliver is unknown. The Child–Pugh score provides a global prognostic scale for cirrhotic patients at large, in patients with both compensated and decompensated cirrhosis. Studies show that the MELD score may be better suited for patients with decompensated liver disease. The MELD score was designed to predict short-term mortality; for longer term mortality prediction, the MELD score may not be superior to the Child–Pugh score [49–51]. Most deaths in persons with compensated cirrhosis occur as a result of transition to a decompensated state. Hence, prediction of development of decompensation as well as mortality specifically due to portal hypertension-related complications is important. This transition is not entirely explained by the prognostic models noted in previous sections. By definition, portal hypertension is a pathologically abnormal portal pressure of 6 mmHg or above. The hepatic venous pressure gradient (HVPG) is an indirect reflection of the portal pressure across the liver [52]. For example, varices form only when HVPG reaches 10 mmHg with a clinically significant risk of bleeding occurring at an HVPG greater than 12 mmHg. Serum albumin, MELD score, and HVPG are independent predictors of development of decompensation from a compensated state [53,54]. An HVPG <10 mmHg has a 90% negative predictive value of development of clinical decompensation over 4 years [53]. Elevated HVPG is an independent predictor of mortality with a purported 3% increase in mortality per 1 mmHg increase in HVPG [55]. A single HVPG measurement greater than 20 mmHg is associated with worse probability of survival (1-year mortality 64% vs. 20%; P <0.01) and early rebleeding from varices [56,57]. Other factors that encapsulate advanced liver disease, such as serum sodium, are also important. A combination of both of hyponatremia (serum sodium <130 mmol/L) and HPVG >20 mmHg is associated with a significantly worse prognosis than either alone. However, it is unclear whether addition of HVPG to MELD of Child–Pugh stage based prediction significantly improves the predictive capabilities of a prognostic model [57].
Evaluating Prognosis1
Introduction
Natural History
Child–Pugh Score
Child–Pugh Score
1 point
2 points
3 points
Total bilirubin (mg/dL)
≤2
2–3
>3
Serum albumin (g/dL)
>3.5
2.8–3.5
<2.8
INR
<1.7
1.7–2.3
>2.3
Ascites
None
Mild
Moderate to severe
Hepatic encephalopathy
None
Grade I–II
Grade III–IV
Points
Stage
5–6
A
7–9
B
10–15
C
MELD score [17]
MELD Na [43]
where the serum sodium concentration (Na) is bound between 125 and 140 mmol/L
ReFit MELD [47]
where bilirubin is bilirubin bounded below by 1 mg/dL; creatinine is creatinine capped by 0.8 mg/dL below and 3 mg/dL above, and; INR is INR bounded by 1 below and 3 above. Renal replacement therapy = 3 mg/dL
MELD Score
MELD Modifications
Delta MELD
Serum Sodium and MELD-Na
Re Weighting Components of MELD
MELD XI
Child–Pugh Score Versus MELD Score
Hepatic Venous Pressure Gradient
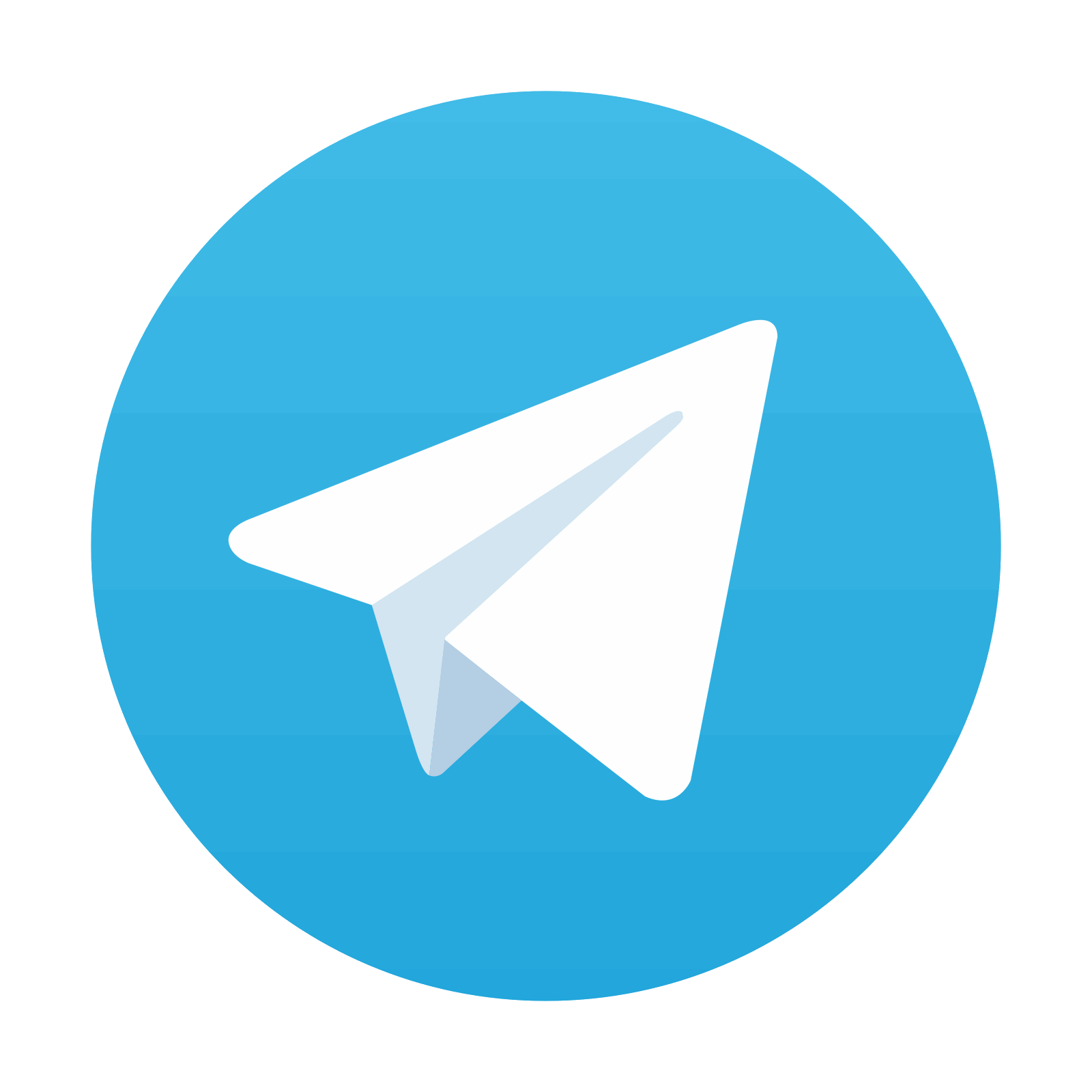
Stay updated, free articles. Join our Telegram channel

Full access? Get Clinical Tree
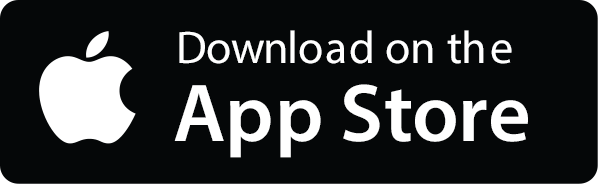
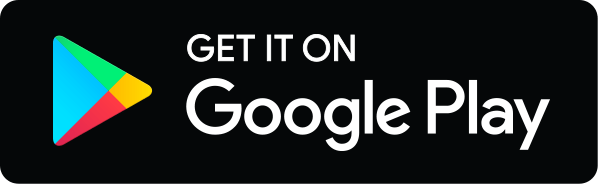