The skeleton has a variety of functions, including mechanical support, protection of soft tissues, locomotion, hematopoiesis, acid–base buffering, and serving as the largest reservoir for calcium and phosphate in the body. Bone mass, the most important determinant of bone strength, is regulated by the activities of bone-forming cells (osteoblasts), bone-resorbing cells (osteoclasts), and osteocytes embedded in the calcified bone matrix. Bone loss and structural deterioration, which are characteristic of menopause, occur when osteoclast activity predominates over bone formation. However, in children with chronic digestive and liver diseases, bone mass is predominantly affected not by increased bone resorption but by deceleration of linear growth, loss of skeletal muscle (which normally applies anabolic mechanical tension to bone), and endocrine disturbances (e.g., delayed puberty, growth hormone resistance). Therefore, there are fundamental differences between postmenopausal osteoporosis (net bone loss and architectural deterioration) and bone formation deficits in children with chronic gastrointestinal and liver diseases brought about by growth stunting, inflammation, and sarcopenia. Because growing children have actively remodeling bones, they may be particularly vulnerable to the effects of disease on the skeleton. However, restoration of health in children offers the prospect of full skeletal reconstitution, a characteristic that may be unique to pediatric patients with growth potential.
This chapter summarizes current knowledge on how digestive and liver diseases affect bone in children and offers suggestions for treatment of gastrointestinal and liver disorders to optimize the chances of achieving peak bone mass in growing children. To achieve this goal, we first review basic bone biology, and then the assessment of bone mass and bone metabolic activity; we then review current knowledge on the effects of digestive and liver diseases on bone metabolism and bone mass in children, and available therapies to enhance bone mass.
Accretion of Bone Mass and Maintenance of Bone Mass
Bone Modeling
Bone size, shape, and architecture evolve continuously in growing children (“bone modeling”) ( Figure 91-1 ). Although linear growth ceases after epiphyses fuse in late puberty, bone continues to acquire mineral content until the early part of the third decade of life. This normal physiologic process is regulated by an array of systemic endocrine and bone paracrine factors. These systems act on osteoblasts, cells derived from mesenchymal precursors, and osteoclasts, which develop from hematologic precursors. Osteoblasts go through a sequence of developmental events controlled by hormones, microRNAs, and transcriptional factors that ensure the proper expression of their mature phenotype and functional properties. The main function of osteoblasts is to form a protein matrix that is rich in type I collagen and contains bioactive factors such as transforming growth factor β (TGF-β), osteonectin, and osteopontin. Under normal circumstances, the collagen matrix becomes mineralized with calcium phosphate crystals to form mature bone tissue. Osteoblasts can respond to a variety of cytokines that are produced in the inflamed intestine and liver and may reach the bone microenvironment.

Most osteoblasts die by apoptosis, but some osteoblasts survive and become embedded in the mineralized matrix and become osteocytes. Osteocytes develop radiating processes that form a network that senses mechanical stress. Osteocytes are the most abundant bone cell type and are increasingly recognized as master directors of both osteoblasts and osteoclasts.
Osteoclasts require receptor activator of nuclear factor κΒ ligand (RANKL) to differentiate and become active. RANKL is produced by osteoblasts and other cells, such as stromal cells and activated T cells, and serves as the final common mediator for osteoclast development. RANKL can be bound to the cell membrane, or released into the extracellular fluid in a soluble form. RANKL binds to its receptor RANK on the osteoclast precursor, stimulating its proliferation, differentiation, and activity. Mice lacking RANKL or RANK have abnormally dense bones because of lack of osteoclasts. These mice also fail to develop lymph nodes, functionally linking the immune system and bone cell biology. Of interest, cytokines associated with inflammation such as tumor necrosis factor α (TNFα) can increase osteoclast formation directly and in synergy with RANKL, and interleukin 17 (IL-17) stimulates osteoclastogenesis directly and via RANKL. On the other hand, interferon γ (IFNγ), IL-4, and IL-12 are potent inhibitors of osteoclast formation by inhibiting RANKL function. Osteocytes secrete RANKL to stimulate osteoclast differentiation and sclerostin, an inhibitor of osteoblast development, thereby holding ultimate control over bone remodeling.
Bone Remodeling
Unlike bone modeling, where bone grows rapidly and changes in shape, bone remodeling eliminates and replaces small quanta of damaged bone over a period of several months. During bone remodeling, the activities of osteoblasts and osteoclasts are coupled. Therefore, increased osteoclast activity leads to osteoblastogenesis and vice versa. Consequently, diseases that decrease osteoblast activity eventually result in decreased bone resorption, a state referred to as low bone turnover. In this state, bone mass is lost primarily because of a decrease in osteoblast function. Conversely, when bone resorption is increased, osteoblast activity is induced. However, because bone formation is much slower than bone resorption, osteoblasts cannot keep up with bone resorptive activity, bone mass is lost, and bone microarchitecture is compromised. This remodeling state is known as high bone turnover (which occurs after menopause, for example). In some diseases, the function of osteoblasts and osteoclasts can be uncoupled; for example, in adults with Crohn’s disease, decreased bone formation can be associated with increased bone resorption. Digestive and liver diseases in children, in general, arrest growth, delay puberty, and reduce muscle mass, leading to a decrease in bone modeling and low bone turnover. This has important implications for the approaches used in children to foster skeletal reconstitution in the setting of chronic gastrointestinal and liver diseases.
Bone Structure
Structurally, bone tissue can either be compact or trabecular. Compact bone, which forms the shafts of long bones, constitutes approximately 80% of the bone mass. It is formed by an array of tightly packed mineralized cylinders, each with an axis formed by a nourishing blood vessel that runs parallel to the long axis of the bone. Each cylinder is made of concentric layers of mineralized matrix in which there are embedded osteocytes (Haversian system). On the other hand, trabecular bone is made by interconnecting mineralized struts and plates (trabeculae), resembling the structure of a sponge, and is located in the epiphyses of long bones, vertebral bodies, and ribs. Each trabecula is made of mineralized matrix containing osteocytes, and lined by osteoblasts and osteoclasts. Trabecular bone is intimately associated with the bone marrow. In adults, trabecular bone is the most metabolically active bone tissue, accounting for 80% of its metabolic activity. Both trabecular bone and cortical bone may be affected in chronic pediatric diseases.
Peak Bone Mass
Peak bone mass is the major determinant of bone strength over the life of the individual. Peak bone mass is determined primarily by heredity. Therefore, a family history of osteoporosis and fractures is a major risk factor for fragility fractures. Other factors that influence bone mass include body weight, muscle mass, physical activity, diet, and ethnicity. Rapid gain of bone mass occurs throughout childhood, especially during puberty when bones grow rapidly in length, volume, and strength. All bone matrix eventually becomes fully mineralized, signaling the end of bone mass accretion, usually early in the third decade of life. At this time, an individual has finished bone modeling (except under special circumstances) and has gained the maximum of bone tissue, called peak bone mass . Peak bone mass is achieved earlier in women than in men because women complete their sexual maturation earlier. There may be a narrow, fixed window of opportunity during puberty and early adulthood when peak bone mass can be acquired. However, newer data suggest that healthy individuals with delayed puberty eventually catch up and show normal bone mass in early adulthood. This has important practical implications for children with gastrointestinal and liver diseases in whom delayed puberty is common.
After late adolescence (around 20 years of age), bone remodeling maintains bone mass and structure, but later in life, bone formation can no longer keep up with bone resorption and bone mass is lost at a steady rate, predominantly at the expense of trabecular bone. Eventually, in some individuals, bone weakens to a point where it may be unable to sustain the stresses of daily living, fails structurally, and fractures with ordinary physical activity or minor trauma.
Every effort should be made to minimize the impact of the underlying disease on nutrition, pubertal maturation, muscle mass, and physical activity to give the best chance to achieve peak bone mass.
Measurement of Bone Mass in Children
Dual X-ray Absorptiometry (DXA)
The most widely available method to measure bone mass is dual x-ray absorptiometry (DXA). In DXA, an X-ray source generates fan beams of two different energies, which are differentially attenuated by bone and soft tissues ( Figure 91-2 ). After traversing the body, the residual X-ray energy is captured by an array of detectors that scan the patient from head to toe in a C-arm configuration. DXA scanners measure bone mineral content (BMC, in grams) and bone area (in cm 2 ). Bone mineral density (BMD) is a calculated value (BMC divided by bone area, g/cm 2 ) and is therefore a projection of the three-dimensional skeleton, not a true volumetric density (in g/cm 3 ). In addition, DXA obtains percent fat and lean tissue. Newer instruments can obtain an image of the lateral spine and analyze vertebral bodies for compression fractures (vertebral fracture analysis, or VFA), but VFA has limitations in children due to the incomplete vertebral mineralization, which can be erroneously interpreted as a vertebral compression fracture.

DXA involves minimal radiation and time and is reproducible; its use has been validated in children, and there are excellent reference data for various DXA instruments. DXA in growing children should only be performed in the total body (minus head) and lumbar spine, and not in the hip or femoral neck. In children with contractures or other issues that preclude proper positioning for DXA, BMD can be measured in lower extremities and results extrapolated based on established normative data. DXA scanners are not interchangeable, so longitudinal measurements should be performed in the same instrument whenever possible, using the same software version.
DXA does not measure the dimension of depth of bone, only bone area. Therefore, bone density by DXA will appear to increase in growing bones, even if the true volumetric density remains constant. Special caution needs to be taken to properly interpret DXA measurements in children with growth retardation and/or delayed puberty, a common complication of gastrointestinal and liver diseases in children. DXA tends to underestimate BMD in children who are small for age, whereas it overestimates BMD in children with larger skeletons. To overcome this limitation, volumetric bone density can be calculated using geometrical assumptions to generate a value called the bone apparent mineral density (BAMD), or can be measured with peripheral quantitative computed tomography (see subsequent text). Alternatively, BMD can be adjusted to height Z score to adjust for short stature.
In children, BMD should always be expressed as a Z score, which measures the deviation of the observed BMD from normative values (Z score = Observed BMD − BMD for age, sex, and race/standard deviation). T scores, which are used in older adults, should never be used in children, because they grossly underestimate their BMD.
As with any ancillary test, DXA should be performed if its results are anticipated to influence the medical management of the patient. Children with chronic gastrointestinal disorders who have risk factors for low BMD, such as continuous use of corticosteroids for more than 3 months, malnutrition, amenorrhea (in girls), and chronic active inflammation, or who have a history of fragility fractures (vertebral compression, extremity fractures with low trauma, or more than two fractures at any site) should have DXA at diagnosis. In children with low BMD (Z score ≤2), DXA may be repeated to assess the result of medical interventions in as early as 3 months if there is active linear growth, or more commonly every 6 to 12 months.
Peripheral Quantitative Computed Tomography
Peripheral quantitative computed tomography (pQCT) of the radius and tibia can determine bone mass, bone architecture, and certain mechanical properties of bone. It involves minimal radiation exposure (comparable to that of DXA). It has several advantages over DXA: pQCT measures volumetric bone density, differentiates compact from trabecular bone, and directly visualizes limb muscle and fat compartments. Parameters of bone strength, stiffness, and bone fragility can be inferred from pQCT measurements. pQCT is currently used primarily in research centers but may be adopted more widely with standardization of measurements at different skeletal sites, improved precision, and generation of normative data.
Bone Biopsy
Bone biopsy can be used to assess bone remodeling and diagnose bone metabolic disease. Transiliac bone biopsy is performed after timed administration of oral tetracycline to label the mineralizing front in the bone so that bone formation rates can be calculated. There are normative data to interpret bone biopsies in growing children. Although it is considered the “gold standard” to assess bone metabolic activity, a major disadvantage of bone biopsy for routine pediatric use is its invasive nature.
Biomarkers of Bone Metabolic Activity
Several indirect markers of bone metabolism that can be measured in serum and urine are used to assess bone formation and bone resorption. However, their interpretation in children is difficult because they depend on multiple factors including linear growth, age, pubertal stage, nutritional status, circadian variation, day-to-day variation, and specificity for bone tissue. For bone formation, available biomarkers include serum osteocalcin, a protein secreted by mature osteoblasts; bone-derived alkaline phosphatase, a marker of osteoblast activity; and procollagen I extension peptides. For bone resorption, the products of type I collagen degradation N- and C-terminal telopeptides (NTx and CTx), and collagen crosslinks (urinary deoxypyridinoline) are measured. At this point, these markers are most often used in the research setting.
Definition of Osteoporosis in Children
Low BMD alone is not sufficient to diagnose pediatric osteoporosis. Instead, the International Society for Clinical Densitometry (ISCD) suggests that in children, low BMD be described as mild or severe depending on the BMD Z score of the total body (minus head) and/or the lumbar spine. A BMD Z score of 2 or less at any site should be reported as “low bone density for chronological age,” without using the terms osteopenia or osteoporosis as in adults. Pediatric osteoporosis is defined by ISCD as bone density Z score of 2 or less, in combination with a fracture.
In healthy children, a relationship between low BMD and fracture risk is emerging from longitudinal studies. A study in preadolescent children (average age of 9 years) showed that bone size relative to body size and humeral volumetric BMD are inversely correlated with fracture risk, irrespective of whether fractures followed slight or moderate/severe trauma. A childhood fracture in men is associated with low BMD and smaller bone size in young adulthood but the same is not true for women. In addition, there appears to be a period of relative bone fragility during the pubertal growth spurt, when long bone area expands more rapidly than it can mineralize. The implications of these findings in children with chronic digestive disorders are not known.
Common Mechanisms by Which Digestive Diseases Affect Bone Mass
Endocrine Dysfunction
A number of normal physiologic processes that are involved in bone modeling and remodeling can be disrupted by chronic gastrointestinal and liver diseases. An important adverse effect of these diseases is pubertal delay. Puberty is characterized by rapid longitudinal bone growth, volumetric expansion, and bone mineralization, and pubertal retardation can undermine normal bone development. Abnormally delayed puberty can permanently affect the attainment of peak bone mass. Estrogen is critical in linear growth in both males and females. A relative estrogen deficiency, as seen in children with delayed sexual maturation, may therefore affect linear bone growth in growing children. During puberty, there is a marked increase in the magnitude and frequency of growth hormone release. This induces the expression of insulin-like growth factor (IGF)-1 in the liver and other tissues, including the skeleton. IGF-1 is a potent growth factor for bone. It stimulates longitudinal growth by chondrocytes and expansion of the outer cortical layer by periosteal osteoblasts. In the trabecular bone compartment, IGF-1 promotes the recruitment of undifferentiated stromal cells into cells of the osteoblast lineage. IGF-1 also is essential for the activation of 1,25-dihydroxyvitamin D. Active chronic diseases in childhood are frequently associated with decreased serum IGF-1, probably as a result of a combination of cytokine effects and nutritional deficiencies (e.g., protein and zinc) that result in inhibition of IGF-1 synthesis in the liver. Serum IGF-1 tends to increase in parallel with clinical improvement and nutritional rehabilitation, which helps to reestablish normal skeletal homeostasis.
Nutritional Factors
Malnutrition due to lack of food intake and/or nutrient malabsorption is common in children with gastrointestinal and liver diseases. Protein and calorie malnutrition is associated with suboptimal bone development. Luminal fatty acids malabsorbed in cholestatic states or enteropathies can bind calcium and prevent its absorption. In addition, it is possible that the inflamed intestine may leak calcium into the lumen and be lost in the stool. Enteral loss of calcium can affect the pool of calcium available to mineralize bone. Moreover, malabsorption of fat-soluble vitamins such as vitamin D and vitamin K can adversely affect bone metabolism. Vitamin D deficiency can result in calcium malabsorption and rickets and vitamin K deficiency can affect the γ-carboxylation of osteocalcin. Children who are ill may spend more time indoors and limit their exposure to direct sunlight, thereby decreasing the synthesis of vitamin D in the skin. Children with gastrointestinal diseases may also limit their intake of vitamin D–fortified dairy products because of primary or secondary lactose intolerance, which will also decrease their calcium intake.
In general, vitamin D bolsters innate immune function while it attenuates the activity of effector immune cells, which may have therapeutic effects in inflammatory bowel disease (IBD) and other gastrointestinal disorders. Specifically, in rodent models of colitis, vitamin D supplementation ameliorates inflammation and the lack of vitamin D receptor exacerbates it. However, it is not known if vitamin D supplementation changes clinical outcomes of IBD in humans. Vitamin K may play a role in normal bone mineralization, as a cofactor in the γ-carboxylation of glutamic acid to form γ-carboxyglutamic acid residues in osteocalcin that can bind calcium. Elevated serum uncarboxylated osteocalcin is a sensitive indicator of vitamin K deficiency, before changes in prothrombin time occur. Deficient osteocalcin carboxylation due to vitamin K deficiency leads to markedly decreased affinity of osteocalcin for calcium. Moreover, osteocalcin plays a role in the control of glucose metabolism, which has important implications for the diabetogenic effects of corticosteroids, which inhibit osteocalcin synthesis by osteoblasts. Subclinical vitamin K deficiency has been reported in patients with Crohn’s disease and cystic fibrosis, and clinical vitamin K deficiency (along with other fat-soluble vitamin deficiencies) occurs in cholestatic liver diseases and may be difficult to correct with oral supplementation.
Decreased Physical Activity
Normal bone development is stimulated by weight-bearing exercise. Children with chronic disease may have limitations in their endurance and prefer activities that are more sedentary. This can potentially decrease bone strength over time. In addition, lean body mass (composed primarily of skeletal muscle) deficits are prevalent in children with chronic gastrointestinal disorders, and lean body mass is an important determinant of bone mass.
Cytokines
Cytokines and other factors released by inflamed tissues can influence the function of bone cells, and bone cells can secrete cytokines conventionally associated with inflammation. For example, IL-6 is an acute-phase reactant commonly elevated systemically in inflammatory diseases. IL-6 can activate bone resorption by osteoclasts and can also inhibit some aspects of osteoblast function but activate others. IFNγ, a product of activated T helper type 1 (Th1) CD4+ cells found in inflamed tissues, can inhibit both osteoclastogenesis and osteoblast function in vitro but also is involved in maintenance of bone mass in vivo. TNFα inhibits osteoblast differentiation and can directly (but modestly) induce osteoclast formation. The specific role of these and other factors in the regulation of bone mass in gastrointestinal and liver diseases requires further elucidation.
RANKL and Osteoprotegerin
RANKL, a member of the TNF family and a potent stimulus for osteoclast differentiation and activity, is synthesized by activated T cells. Osteoprotegerin (OPG) is a soluble decoy receptor for RANKL. OPG is a potent inhibitor of osteoclast formation and activity. Of interest, OPG is widely expressed in many tissues in addition to bone, including and in the gastrointestinal tract. The function of OPG and RANKL in the intestine is incompletely understood. High expression of OPG in the colon is associated with resistance to treatment with intravenous corticosteroids and infliximab in patients with ulcerative colitis, respectively. RANKL is necessary for normal development and functional M cells in Peyer’s patches. RANKL binds to its receptor RANK in dendritic cells (DCs) and increases their survival and activity. However, the effect of RANKL on DCs depends on the body compartment from which DCs are harvested. Whereas DCs from spleen are activated by RANKL, DCs from Peyer’s patches adopt a phenotype of tolerance. Moreover, RANKL induces oral tolerance to an experimental antigen and RANKL suppresses cytokine responsiveness to lipopolysaccharide (LPS) in vivo. OPG neutralization of RANKL inhibits DC activity. Taken together, it is possible that intestinal OPG may be proinflammatory, whereas RANKL induces tolerance to luminal antigens that is mediated by DCs. These intriguing osteoimmune connections require further study.
Medications
Medications used to treat digestive disorders can also affect bone cell function. Corticosteroids decrease BMD and increase the risk of fractures in adults, an effect that occurs early in the course of therapy and can be observed even with small doses. A large case-controlled study involving children who received four or more courses of oral corticosteroids (mean duration 6.4 days), found an increased risk of fracture among children who received the medication as compared with controls. Corticosteroids affect both osteoblasts and osteoclasts, although their initial effect is primarily on osteoblasts. Glucocorticoids directly inhibit osteoblast function and decrease osteoblast number. In addition, corticosteroids can affect osteoblasts indirectly by decreasing the synthesis of anabolic sex steroids. Corticosteroids can also inhibit the activity of vitamin D and stimulate parathyroid hormone (PTH) activity, thus stimulating bone resorption. Increased PTH level promotes renal elimination of calcium and phosphate. However, it is difficult to dissect the effect of corticosteroids on skeletal mass from the underlying disease for which they are prescribed. In addition, corticosteroids may have effects that promote bone mass accrual, including increased food intake, higher activity level, and suppression of inflammation. Therefore, the net effect of corticosteroids on bone mass in children may be positive or negative in the individual patient. The calcium-binding phosphatase calcineurin regulates osteoblast function, and its substrate nuclear factor of activated T cells (NFAT) is critically important in osteoblast and osteoclast differentiation. Calcineurin inhibitors such as cyclosporine and tacrolimus may therefore have significant effects on bone cells, but as with corticosteroids, their net effect in the human skeleton is difficult to separate from the condition for which they are used. Limited information on IFNα suggests that it increases RANKL production by osteoblasts and therefore enhances osteoclast formation and activity. Treatment of chronic hepatitis C with IFNα and ribavirin may result in bone loss. Ribavirin in vitro reduces osteoblast formation. These effects have not been confirmed in vivo. Cholestyramine used to treat pruritus from cholestasis or diarrhea due to unconjugated and malabsorbed acids can adsorb vitamin D and prevent its absorption from the intestinal lumen. Loop diuretics for treatment of ascites can increase urinary calcium loss. Recently concerns have been raised in older adults about a possible increase of fractures in chronic users of proton pump inhibitors (PPIs). However, the risk of fracture in children who use chronic PPI is currently not known.
Selected Gastrointestinal and Liver Diseases Associated with Decreased Bone Mass
Inflammatory Bowel Diseases
Both Crohn’s disease and ulcerative colitis are associated with decreased bone mass. Children with Crohn’s disease more commonly exhibit deficits in bone mass and bone architecture than children with ulcerative colitis, probably due to the negative effects of Crohn’s disease on linear growth, pubertal progression, and lean body mass, which are less pronounced in ulcerative colitis. Nontraumatic fractures have been reported in children with IBD, especially in vertebral bodies. On the other hand, extremity fractures do not appear to be more common in children with IBD than in age-matched controls, based on the interrogation of a large administrative database and a study using a recall questionnaire of parents and caregivers. Additional studies are needed to determine the prevalence of vertebral fractures in children with IBD, however, because compression fractures of the vertebrae can be asymptomatic and not come to clinical attention.
The pathogenesis of bone loss in IBD, as in other digestive disorders, is multifactorial, with roles for protein-calorie malnutrition, vitamin deficiencies (e.g., vitamin D and possibly vitamin K ), malabsorption, inactivity, hypogonadism, corticosteroid use, and systemic inflammation. In addition, alterations in klotho associated with intestinal inflammation may produce renal calcium losses and disturbances in phosphate metabolism that lead to decreased bone mass.
Biochemical markers of bone metabolism suggest that bone formation is primarily impaired in pediatric IBD. Although bone-formation markers can be reduced in children with impaired growth (which is commonly associated with IBD), transiliac bone biopsies in children newly diagnosed with Crohn’s disease suggest decreased bone formation as well. Moreover, in an in vitro model of intact bone, serum from newly diagnosed children with Crohn’s disease decreased bone weight and calcium incorporation, whereas serum from children with ulcerative colitis had no effect. Similar studies using primary osteoblast cultures showed that osteoblast function is impaired by serum from newly diagnosed, untreated children with Crohn’s disease. Therefore, soluble factors present in Crohn’s disease that may reach bone through the systemic circulation may affect osteoblast function and decrease bone formation.
Medical therapies that spare children with Crohn’s disease of corticosteroid exposure and effectively treat intestinal inflammation appear promising to reconstitute skeletal health. One such therapy is infliximab, an anti-TNFα antibody, which in the short term is associated with a sharp increase in biomarkers of bone turnover, especially bone formation. Exclusive enteral nutrition also offers the benefit of improved bone health in children with Crohn’s disease, but the effect may not be sustained. However, conventional induction of remission with corticosteroids followed by maintenance with a thiopurine in Crohn’s disease is associated with persistent deficits in height, bone mass, and skeletal muscle mass.
A rare skeletal complication associated with IBD is chronic recurrent multifocal osteomyelitis. This condition is associated with sterile inflammation of the clavicles, vertebrae, and long bones, and it usually appears years before the onset of gastrointestinal symptoms. It is not specific to IBD, as it can occur in other chronic inflammatory diseases. It responds to treatment of the underlying disease, including infliximab for Crohn’s disease.
Celiac Disease
Loss of bone mass can occur in patients with celiac disease, even in patients who do not present with classic symptoms of malnutrition and malabsorption. The risk of hip fracture in children with celiac disease may be increased, but children who strictly follow a gluten-free diet have an excellent chance of full skeletal repair postdiagnosis.
The pathogenesis of bone loss in celiac disease is multifactorial. Studies in children suggest that bone formation is reduced at the time of diagnosis, as judged by decreased serum osteocalcin and carboxy-terminal peptide of type I collagen (PICP). Bone remodeling becomes significantly more active after the initiation of a gluten-free diet, as determined by biochemical indices of bone turnover. Bone turnover may be inhibited by inflammation in untreated children with celiac disease. For example, elevated serum IL-6, a marker of active inflammation, and reduced IL-1ra, an anti-inflammatory factor, correlate with decreased BMD in celiac disease at diagnosis. Malnutrition may also adversely affect bone metabolism in celiac disease, but bone mineral deficits occur in well-nourished individuals with celiac disease. Dietary calcium may bind to unabsorbed fatty acids and form indigestible soaps, but this has not been proven in celiac disease. Malabsorption of vitamin D can further impair intestinal calcium absorption and lead to secondary hyperparathyroidism and phosphaturia. Clinical vitamin K deficiency with prolongation of prothrombin time has been observed in patients with celiac disease. Vitamin K deficiency may also affect the posttranslational γ-carboxylation of osteocalcin, a matrix protein that plays a role in bone calcification and affect bone mass accrual. Magnesium deficiency has been detected by sensitive methods in patients with celiac disease, but others have reported normal levels. Magnesium deficiency can decrease bone turnover.
DXA should not be performed in most children with celiac disease, because the result of DXA would not help with their management and it is likely that their bone mass will recover if they adhere to a gluten-free diet. DXA may be justified in children who strictly avoid gluten and sustain a lower extremity or vertebral fracture, or more than two fractures at any site.
Cholestatic Liver Disease
Cholestatic diseases such as extrahepatic biliary atresia and Alagille syndrome are frequently associated with reduced bone mass, as judged by DXA, even when adjusted for reduced body size. Fragility fractures commonly occur in children with chronic cholestasis, but the prevalence of fractures in children with cholestasis is not known. In a rat model of cholestasis (bile duct ligation), bone histomorphometry shows signs of reduced bone volume and bone formation, a decreased osteoblast pool, and increased bone fragility. The mechanisms by which cholestasis affects bone in children are complex. Malnutrition is common despite caloric supplementation due to abnormal nutrient utilization. Decreased muscle mass is prevalent and may affect bone modeling (and the outcome of liver transplantation). Puberty may be delayed, resulting in relative hypogonadism. Biochemical markers of bone metabolism suggest decreased bone formation. The precursor for the formation of vitamin D in the presence of UV light (7-dehydrocholesterol) is present in normal concentration in the skin of cholestatic patients, so skin photoconversion of vitamin D is probably normal. However, vitamin D malabsorption is common and serum concentration of 25-hydroxyvitamin D, which reflects vitamin D stores, needs to be followed carefully, even when seemingly adequate fat-soluble vitamin supplementation is provided. Nevertheless, 25-OHD levels might be falsely low due to reduced vitamin D–binding protein, which carries vitamin D metabolites, while free 25-OHD remains normal. Radiologic changes of rickets secondary to vitamin D deficiency may be present in children with cholestasis.
Plasma protein induced in vitamin K absence II (PIVKA-II), an indicator of vitamin K stores, is often reduced in children with cholestasis. This potentially can affect the γ -carboxylation of osteocalcin, which is important for bone mineralization. Calcium absorption is normal in cholestatic patients who have normal serum vitamin D, but may be decreased in children with vitamin D deficiency. IGF-1 is a potent anabolic agent for bone growth. Of interest, plasma growth hormone (GH) concentration is elevated in children with cholestasis, while plasma IGF-1 and IGF-binding protein 3 are both low. This suggests that in chronic cholestasis, there is peripheral resistance to GH, decreased production of IGF-1 in the liver and other tissues, and IGF-1 may be cleared faster from plasma. Unconjugated bilirubin has direct negative effects on osteoblast proliferation and function in vitro, whereas lithocholic acid inhibits responses to vitamin D in osteoblasts. The negative effects of cholestasis on osteoblast function, however, may be reversible at least in part by treatment with ursodeoxycholic acid. In addition, specific genetic defects associated with Alagille syndrome in the Notch pathway may directly affect osteoblast differentiation and skeletal maturation.
Children with cholestatic liver disease who are receiving parenteral nutrition can develop hypercalcemia of unknown cause. Intravenous pamidronate, a bisphosphonate, is used to control this metabolic abnormality.
Liver Transplantation
Hepatic osteodystrophy is common in children with end-stage liver disease. However, hepatic osteodystrophy may be reversible in children post-liver transplantation. Bone biomarkers may normalize post-transplantation, and BMD and BMC of the total body may improve.
Liver transplantation has an anabolic effect on linear growth and bone mass, and bone mineral content may become normal 1-year posttransplantation. Newer anti-rejection regimens that limit exposure to corticosteroids may help the skeletal recovery and catch-up growth. Restoration of normal liver function, with consequent improvement in nutrient intake, absorption, linear growth, muscle mass, pubertal progression in adolescents, and physical activity may outweigh the negative effects of immunosuppressive therapy on bone metabolism in these children. However, continuous monitoring of physical and sexual development is important, especially for children with pretransplantation growth retardation, re-transplantation, and a diagnosis other than biliary atresia, and in non-whites.
Although fragility fractures occur relatively frequently in children after liver transplantation, more studies are needed to examine their precise risk, especially in the context of more refined posttransplantation medical therapy that reduces total steroid exposure. In addition, some patients receive liver grafts from living-related donors, which allow for earlier surgery and may prevent long-term complications from end-stage liver disease such as bone loss. Nevertheless, vertebral compression fractures (which may be asymptomatic) are more common than long bone fractures post-liver transplantation and may occur in patients with normal BMD by DXA.
Cystic Fibrosis
Bone loss and fractures have been reported in both adults and children with cystic fibrosis. Risk factors for cystic fibrosis–related bone disease include poor nutritional status, lung infection and chronic inflammation, severity of lung disease, pancreatic insufficiency, vitamin D and K deficiencies, negative calcium balance, hypogonadism, delayed puberty, cystic fibrosis–related diabetes (CFRD), glucocorticoid treatment, reduced weight-bearing activity, organ transplantation, and immunosuppressive therapy.
Cystic fibrosis–related bone disease can be progressive and result in significant kyphosis due to vertebral fractures, with consequent restriction of lung function. Fractured ribs can make it more difficult to cough effectively and to perform chest physiotherapy. Approximately one-third of patients with cystic fibrosis have a significant decrease in bone mineral mass. Low BMD by DXA correlates with decreased lung function and mortality.
The precise prevalence of osteopenia in children with cystic fibrosis is debated, because of methodologic limitations of available studies (small sample sizes, mixed age groups, inclusion of severely ill adults) and because patients with cystic fibrosis can be short for their age, so their bone density can be underestimated by DXA. There may be regional differences in BMD in patients with cystic fibrosis, depending on their content of trabecular or cortical bone. Fractures tend to occur in skeletal sites that are rich in trabecular bone in cystic fibrosis. Louis et al. reported normal radius BMD measured by pQCT in well-nourished young adults with cystic fibrosis, but reduced cortical thickness, whereas Gronowitz et al. using similar methodology in well-nourished patients with cystic fibrosis observed an increase in endosteal surface. This could affect the biomechanical properties of long bones such as resistance to bending and make them more prone to fracture. Increased longevity in patients with cystic fibrosis may convey a higher risk of bone loss.
The mechanisms by which cystic fibrosis affects bone are complex and not fully elucidated. There are both disease and treatment factors involved. In the F508del-CFTR mice, bone formation rate is lower than in sex- and age-matched control mice. Bone histomorphometry data are limited in humans with cystic fibrosis. A 25-year-old man with cystic fibrosis who sustained a fragility fracture of the femoral neck and was treated with an oral bisphosphonate had severe cortical and trabecular osteopenia with no osteomalacia. A study primarily in posttransplantation patients with cystic fibrosis revealed evidence of decreased bone formation, increased osteoclast number, and areas of bone resorption in postmortem bone biopsies. Serum osteocalcin is low in young patients with cystic fibrosis, suggesting decreased osteoblast activity and bone formation. A longitudinal study of patients with cystic fibrosis that included both children and adults showed failure to gain bone mineral at the expected rate. As judged by balance studies using stable isotopes of calcium, rates of calcium deposition into bone are decreased despite normal calcium absorption in clinically stable females with cystic fibrosis. Based on this evidence, it appears that cystic fibrosis can decrease bone formation and activate bone resorption.
Recent calcium balance data suggest that excessive fecal losses of calcium occur in girls with cystic fibrosis, despite normal calcium absorption, suggesting that calcium is abnormally leaked back into the intestinal lumen. Hardin et al. have reported that recombinant growth hormone improves general health in patients with cystic fibrosis and bone mineral content, but there are concerns about the diabetogenic effects of GH in patients with cystic fibrosis and the theoretical potential of inducing malignancy.
Optimizing bone health in children, adolescents, and adults with cystic fibrosis involves a multifaceted approach. General approaches include nutritional interventions, vitamin K, vitamin D, and calcium supplementation, if necessary, as well weight-bearing exercise. European Cystic Fibrosis Society guidelines are available concerning desired serum vitamin D concentration and supplementation regimens for patients with cystic fibrosis. Bisphosphonate therapy can increase bone mass in patients with cystic fibrosis, and the European Cystic Fibrosis Society has recently proposed clear guidelines for their use in children and adults with cystic fibrosis. Intravenous bisphosphonates can, however, cause bone pain in cystic fibrosis. Therefore, pediatric endocrinologists with expertise in bone active agents should be involved when bisphosphonates are being considered in patients with cystic fibrosis.
Hypertrophic Pulmonary Osteoarthropathy
Patients with cystic fibrosis with advanced lung disease may develop joint swelling, pain, and morning stiffness. The etiology of this complication is not understood. It is usually controlled with nonsteroidal anti-inflammatory drugs, physiotherapy, and sometimes corticosteroids. Intravenous pamidronate, a bisphosphonate that inhibits bone resorption, has also been used.
Treatment
In the absence of pediatric data, it is common to extrapolate indications and results obtained with therapeutic agents in adults. This approach has risks when it comes to increasing bone mass and preventing fractures in children, given important physiologic differences between children and adult bone biology.
Reduced BMD alone should not be used as the sole criterion for starting bone active agents such as bisphosphonates in a child. On the other hand, measures aimed at preventing loss of bone mass and deterioration of bone architecture should be considered in any child with chronic digestive and liver diseases.
In children in whom a fracture(s) have occurred, risk factors for osteoporosis should be identified and, if possible, corrected. These children should be considered for pharmacologic therapy for preventing future fractures and alleviating bone pain, in consultation with a pediatric endocrinologist with expertise in bisphosphonates.
Bone-active agents besides bisphosphonates for children with fractures are limited. Teriparatide, a recombinant human parathyroid hormone, hPTH (1-34), is approved by the U.S. Food and Drug Administration (FDA) for treatment of osteoporosis in adults, but its use is contraindicated in individuals whose growth plate is not fused because of the theoretical concern of promoting the development of osteosarcoma. Teriparatide was used in a newborn with refractory hypocalcemia. Denosumab, an anti-RANKL antibody, decreases bone resorption, increases bone mass, and prevents fractures in postmenopausal women with osteoporosis and in men receiving androgen-deprivation therapy for nonmetastatic prostate cancer. Denosumab has been used in individual children with rare primary metabolic bone diseases associated with increased bone resorption. Romosozumab, an antibody directed at sclerostin (an inhibitor of osteoblast function), increases bone mass in postmenopausal women with osteopenia, increases bone formation, and reduces bone resorption. There are no reports of pediatric use of romosozumab at the time of this writing. Intranasal calcitonin does not increase BMD in children with Crohn’s disease.
General Measures
Appropriate treatment of the underlying gastrointestinal and liver disease in children with growth potential offers the best hope for catch up growth, improved muscle mass, and reaching genetically programmed peak bone mass. Attention should be paid to minimizing exposure to corticosteroids, increasing weight-bearing physical activity, and optimizing nutrition, including ensuring adequate calcium and vitamin D.
Vitamin D
Vitamin D is essential for normal calcium absorption. Vitamin D is found naturally in a small number of foods (e.g., oily fish, cod liver oil, irradiated shiitake mushrooms) and in foods supplemented with vitamin D (milk and cereals). Vitamin D is synthesized in humans, with the first step involving unprotected skin exposure to UVB light. Due to the angle of incidence of sunlight during autumn and winter, individuals living in high northern or low southern latitudes are at risk for vitamin D deficiency. In addition, proper use of sun block minimizes the cutaneous synthesis of vitamin D precursors.
Vitamin D deficiency (serum 25-OHD <20 ng/mL or <50 nmol/L) is common in diseases that affect the gastrointestinal tract and liver. In healthy individuals, the Institute of Medicine (IOM) recommended dietary allowance (RDA) is 600 IU vitamin D daily to maintain skeletal health, with an upper level of safe intake set at 4,000 IU of vitamin D per day in adolescents. These recommendations have generated considerable debate, with the Endocrine Society defining vitamin D insufficiency as 25-OHD level of less than 30 ng/mL or less than 75 nmol/L and advocating at least 1,000 IU vitamin D daily for high-risk children and adolescents. Vitamin D3 (cholecalciferol, derived from animal sources) is more effective than vitamin D2 (ergocalciferol, from plant sources) to increase vitamin D levels. The bioavailability of vitamin D may be affected by the plasma concentration of vitamin D–binding protein in individuals of different race.
In sick children, doses required to correct vitamin D deficiency or maintain adequate vitamin D levels may be higher than in healthy children. In addition, overweight and obese children may require higher doses of vitamin D, because it is thought that vitamin D is sequestered in adipose tissue.
There is compelling epidemiologic and experimental evidence that vitamin D may have important extraskeletal effects. Vitamin D receptor is ubiquitous in cells of the immune system, and vitamin D in general enhances the activity of the innate immune system while it reduces adaptive immune function, which may be beneficial in Crohn’s disease, for example. However, direct evidence is still lacking that vitamin D supplementation to achieve serum 25-OHD 20 ng/mL or greater is clinically beneficial for extraskeletal sites.
Calcium
Calcium and phosphate are essential for normal bone mineralization. Calcium is essential for many cellular functions. Bone is the largest calcium reservoir. Phosphate is ubiquitous in the diet. However, calcium is present in a more restricted list of foods, including milk (276 mg/8 oz whole milk), yogurt (415 mg/8 oz serving of low fat, plain yogurt), cheese (~310 mg/45 g), and bones of canned fish (325 mg/90 g sardines). Calcium is present in some plant sources (broccoli, bok choy, kale, turnip) but phytates and oxalates that bind calcium limit its bioavailability. Calcium is also added to juices (261 mg/6 oz of fortified orange juice). Therefore, children with diseases of the digestive tract and liver may need calcium supplementation.
The RDA for calcium increases with age, from 200 mg in newborns to 1,300 mg in adolescents up to 18 years of age, with tolerable upper limits of 1000 mg in newborns and 3000 mg in adolescents. Children catching up in growth may need additional calcium, however. If the RDA cannot be met with dietary intake, calcium supplements can be used. Both calcium carbonate (40% calcium by weight) and calcium citrate (21% calcium) are widely available. Calcium carbonate is cheaper. It should be taken with food for optimal absorption. Calcium citrate on the other hand can be taken with or without food. The dose of a calcium supplement should be divided to increase the absorption of elemental calcium.
Bone-Active Agents
Bisphosphonates are derivatives of pyrophosphate that have been shown to increase BMD and decrease the risk of new fractures in postmenopausal women and in patients receiving long-term corticosteroids. These drugs are potent antiresorptive agents. Although oral forms are poorly absorbed, they accumulate in bone tissue where they can remain for several years. There is very limited published experience with these agents in children with digestive diseases and decreased bone mass. Most experience is with intravenous pamidronate in children with osteogenesis imperfecta (OI), followed by oral risedronate in children with OI (based on a randomized, double-blind, placebo-controlled trial [DBPCT]). In children with OI, bisphosphonates increase BMD, decrease bone resorption, and do not affect fracture healing. A small DBPCT in 19 nonambulatory children and young adults showed a significant increase in lumbar spine BMD in patients treated with oral risedronate for 2 years.
Before treatment with bisphosphonates can be recommended, it is important to remember that no trials have been conducted in children with digestive and liver diseases using reduced fracture risk as an endpoint. Instead, primary outcomes are increased in BMD. Increases in BMD associated with bisphosphonate use may not necessarily result in decreased fracture risk, however. In addition, one needs to consider that digestive and liver diseases in children appear in general to retard bone formation rather than increase bone resorption, suggesting that bisphosphonates may have a limited role in the absence of fractures. The administration of bisphosphonates should always be done in conjunction with a pediatric endocrinologist with experience in bone diseases.
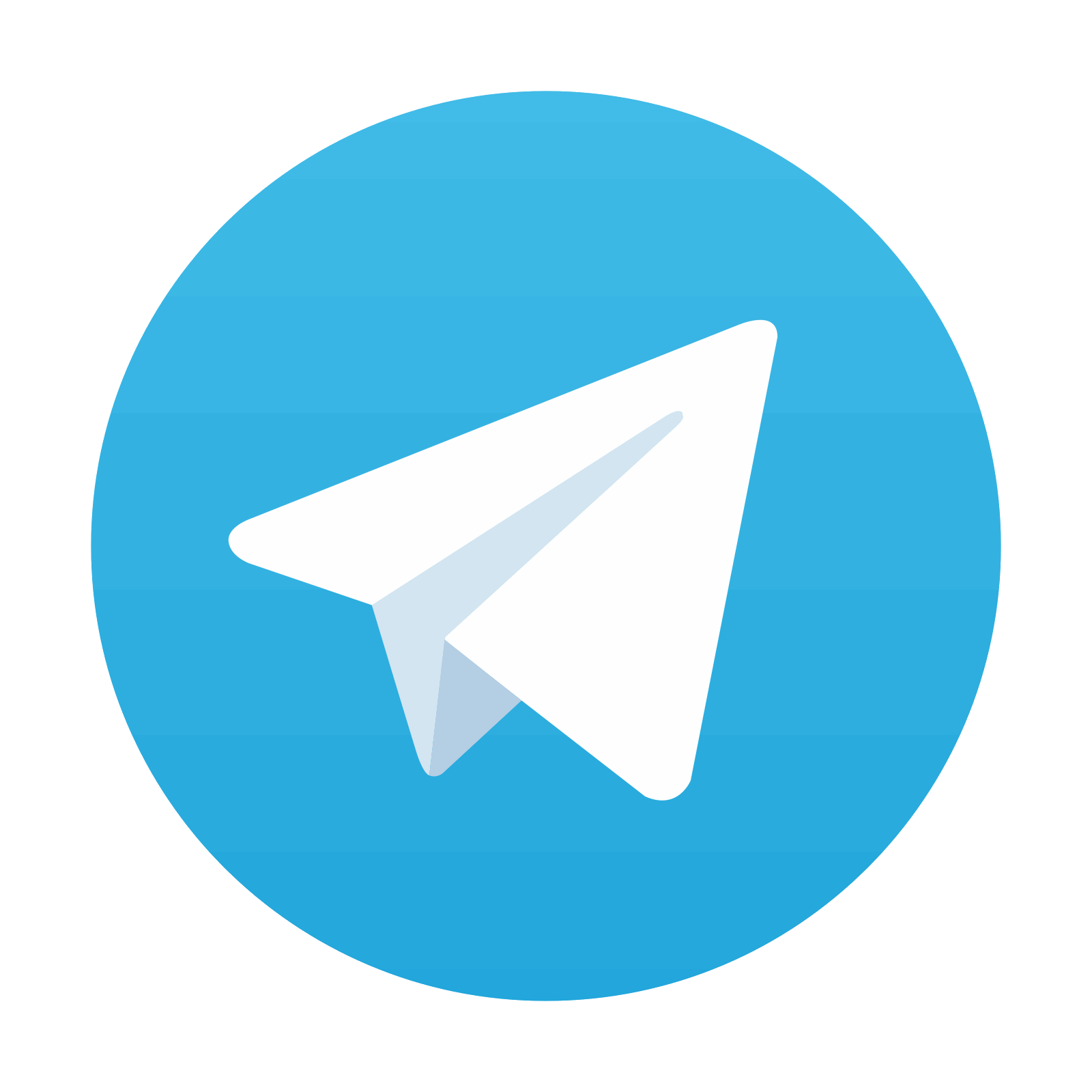
Stay updated, free articles. Join our Telegram channel

Full access? Get Clinical Tree
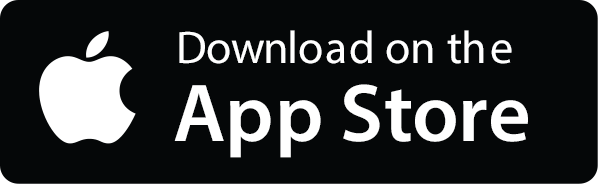
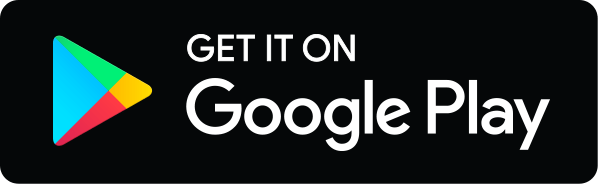