Disorders of Calcium Homeostasis
The extracellular fluid (ECF) calcium concentration in the human body is tightly regulated by a complex process. Three organs—skeleton, kidney, and intestine—are involved in this process through their direct or indirect interaction with parathyroid hormone (PTH), parathyroid hormone–related peptide (PTHrP), vitamin D, and calcitonin. Phosphatonins such as fibroblast growth factor 23 (FGF-23), although they participate in phosphate and vitamin D homeostasis, do not directly modify extracellular calcium.
This homeostatic system is modulated by dietary and environmental factors, including vitamins, hormones, medications, and mobility. Disorders of extracellular calcium homeostasis may be regarded as perturbations of this homeostatic system, either at the level of the genes controlling this system (e.g., as in familial hypocalciuric hypercalcemia, pseudohypoparathyroidism, or vitamin D–dependent rickets) or perturbations of this system induced by nongenetic means (e.g., as in lithium toxicity or postsurgical hypoparathyroidism).
Calcium fluxes between the ECF and one of the organs (skeleton, kidney, and intestine) or their combination, as well as abnormal binding of the calcium to serum protein, can cause hypercalcemia. PTH directly protects against hypocalcemia by augmenting calcium mobilization from bone, increasing renal tubular reabsorption of calcium, and enhancing intestinal absorption of calcium. PTH indirectly protects against hypocalcemia through its effect on vitamin D metabolism. States with excess PTH may cause hypercalcemia, whereas PTH deficiency is associated with hypocalcemia. Similarly, PTHrP promotes bone resorption, enhances renal reabsorption of calcium, and decreases renal tubular reabsorption of phosphate. Excess of this hormone is responsible for the hypercalcemia of malignancy. Vitamin D and its metabolites increase intestinal absorption of calcium and cause bone resorption; therefore, excess vitamin D would induce hypercalcemia. Calcitonin inhibits bone resorption, but its physiologic role in the protection against hypercalcemia in humans is not proven.
Whole-Body Calcium Homeostasis
An adult human body contains approximately 1000 to 1300 g of calcium, with 99.3% in bone and teeth as hydroxyapatite crystal, 0.6% in soft tissues, and 0.1% in ECF, including 0.03% in plasma. Maintenance of normal calcium balance and serum calcium levels depends on the integrated regulation of calcium absorption and secretion by the intestinal tract, excretion of calcium by the kidney, and calcium release from and deposition into bone. In young adults, calcium balance is neutral. Approximately 1000 mg of calcium is ingested per day, 200 mg absorbed by gut, mainly duodenum and 800 mg excreted via the gut. Out of 10 g of calcium filtered by the kidney daily, only approximately 200 mg is excreted in the urine. At the same time, 0.5 g of calcium is released from bone, and the same amount is deposited with new bone formation. PTH, by stimulating bone resorption and distal tubular calcium reabsorption in the kidney, and activating renal hydroxylation of 25(OH)D 3 to 1,25(OH)D 3 , increases serum calcium levels. Depression in serum levels of calcium, by itself, stimulates, through the calcium-sensing receptor (CaSR) in the parathyroid gland, the secretion of preformed PTH from the parathyroid gland within seconds. Subsequently, PTH biosynthesis by the parathyroid gland increases over 24 to 48 hours and is followed by parathyroid gland hypertrophy and hyperplasia. Vitamin D metabolites, serum phosphorus, and FGF-23 levels also regulate PTH levels in blood.
The values for total serum calcium concentration in adults vary among clinical laboratories, depending on the methods of measurement, with the normal range being between 8.6 and 10.3 mg/dL (2.15 to 2.57 mmol/L). Variation in serum calcium levels occur, depending on age and gender, with a general trend for lower serum calcium level with aging.
Calcium in blood exists in three distinct fractions—protein-bound calcium (40%), free (ionized) calcium (48%), and calcium to complexed various anions, such as phosphate, lactate, citrate, and bicarbonate (12%). The latter two forms, complexed calcium and free calcium ion, together comprise the fraction of plasma calcium that can be filtered. Plasma albumin is responsible for 90% and globulins for 10% of protein-bound calcium. Free calcium is the physiologically active component of extracellular calcium with regard to cardiac myocyte contractility, neuromuscular activity, bone mineralization, and other calcium-dependent processes. It is measured in most hospitals using ion-selective electrodes; values in adults range from 4.65 to 5.28 mg/dL (1.16 to 1.32 mmol/L). Total calcium reflects the levels of free calcium if plasma levels of protein, pH, and anions are normal.
The relationship between calcium ion and the concentration of protein in the serum is represented by a simple mass action expression:
( [ Ionized Ca 2 + ] × [ protein ] ) / [ calcium proteinate ] = K
In conventional units:
Adjusted total calcium ( mg / dL ) = total calcium ( mg / dL ) + 0.8 ( 4 − albumin [ g / dL ] )
In SI units:
Adjusted total calcium ( mmol / L ) = total calcium ( mmol / L ) + 0.02 [ 40 − albumin ( g / L ) ]
This formula was endorsed in 1977 by an editorial in the British Medical Journal ; the correction factor of 0.02 (in SI units) was chosen arbitrarily for simplicity from the range available in the literature at that time (0.018 to 0.025). This adjustment can also correct for errors in measurement of total calcium related to hemoconcentration of a blood sample because of the prolonged use of a tourniquet or because of hemodilution when blood is drawn in a supine position in hospitalized patients. Other formulas have been developed, particularly for chronic kidney disease (CKD) patients, that have a slightly better (although not statistically significant) discriminatory ability to make the diagnosis of hypocalcemia or hypercalcemia, as established from measurement of free calcium. Also, a fall in pH of 0.1 unit will cause approximately a 0.1 mEq/L rise in the concentration of ionized calcium, because hydrogen ion displaces calcium from albumin, whereas alkalosis decreases free calcium by enhancing the binding of calcium to albumin. There is no correction for this effect of pH in the above formula, which also limits its accuracy.
Calcium binding to globulin is small (1.0 g of globulin binds 0.2 to 0.3 mg of calcium), and it is unusual to see a change in the total concentration of serum calcium as a result of alterations in the levels of globulin in blood. However, in cases in which the globulin concentration in serum is extremely high (>8.0 g/dL), such as in multiple myeloma, a mild to moderate hypercalcemia may be seen because of an elevation of the globulin-bound calcium. In addition, immunoglobulin G (IgG) myeloma proteins may have increased calcium-binding properties, and an elevation in the total level of serum calcium could occur, even with a moderate increase in serum levels of globulins. In these cases, the ionized calcium in serum is normal; therefore, this type of hypercalcemia would not require treatment.
Unfortunately, calcium status will be incorrectly predicted by this formula in 20% to 30% of subjects, and the agreement between corrected and free calcium is only fair. Thus, free calcium should be assessed, particularly in critically ill patients with acid-base disturbances, in patients exposed to large amounts of citrated blood, and in those with severe blood protein disorders. Patients with CKD and those treated with dialysis may also benefit from free calcium measurements in an evaluation of their mineral bone metabolism status. It is important to recognize that free calcium results in blood are affected by factors related to the handling of specimens, including duration of cellular metabolism, loss of CO 2 , and use of anticoagulants.
Hypercalcemia
Hypercalcemia is relatively common and frequently overlooked, with an annual incidence estimated to be about 0.1% to 0.2% and a prevalence of 0.17% to 2.92% in a hospital population and 1.07% to 3.9% in a normal population.
Hypercalcemia results from an alteration in the net fluxes of calcium to and from four compartments—bone, gut, kidney, and serum binding proteins ( Table 19.1 ). Usually, the hypercalcemia is caused by net calcium movement from the skeleton into ECF through increased osteoclastic bone resorption, as in hyperparathyroidism (HPT) or excess PTHrP production in malignancy. PTH acts via the PTH receptor 1 on the osteoblasts. PTH receptor 1 is encoded by the PTH1R gene. It activates cyclic adenosine monophosphate (cAMP) signalling in osteoblasts and upregulates their expression of receptor activator for nuclear factor-kappaB (RANK) ligand (RANKL). RANKL binds to RANK on osteoclasts. RANK activation on osteoclasts through the RANK/RANKL interaction causes recruitment, proliferation, and activation of osteoclasts for bone resorption. Increase in bone resorption rate without increase in bone formation rate will cause hypercalcemia.
|
Myeloma cells may induce multiple osteoclastogenic factors such as RANKL in nonosteoblastic stromal cells or decrease production of osteoprotegerin, a decoy receptor for RANKL. Excess circulating 1,25-dihydroxyvitamin D (1,25[OH] 2 D) from various causes also activates osteoclastic bone resorption indirectly through osteoblasts. Increased intestinal calcium absorption may lead to the development of hypercalcemia, as in vitamin D overdose or milk-alkali syndrome. In general, the kidney does not contribute to hypercalcemia; rather, it defends against the development of hypercalcemia. Typically, hypercalciuria precedes hypercalcemia. Extracellular calcium itself appears to have a calciuric effect on the renal tubule by its direct action on the CaSR of the thick ascending limb (TAL). Thus, in most hypercalcemic states, renal calcium handling is subject to competing influences; excess PTH or PTHrP acts on the PTH/PTHrP receptor to promote renal calcium reabsorption, and excess calcium acts on the calcium receptor to promote calcium excretion.
In rare cases, the kidney can actively contribute to the development of hypercalcemia. As opposed to primary HPT and humoral hypercalcemia of malignancy, in which increases in renal calcium excretion are observed, renal calcium excretion is not elevated in familial hypocalciuric hypercalcemia because of a defective renal response to calcium itself. The hypercalcemia associated with thiazide use is also mediated by the kidney: in both thiazide use and its genetic counterpart, Gitelman’s syndrome, renal calcium excretion is decreased.
Signs and Symptoms
Hypercalcemia adversely affects the function of almost all organ systems, but in particular the kidney, central nervous system, and cardiovascular system. The clinical manifestations of hypercalcemia relate more to the degree of hypercalcemia and rate of increase than the underlying cause. Hypercalcemia may be classified based on the level of total serum calcium :
Mild: [Ca] = 10.4 to 11.9 mg/dL
Moderate: [Ca] = 12.0 to 13.9 mg/dL
Severe (hypercalcemic crisis): [Ca] = 14.0 to 16 mg/dL
(Much higher levels are occasionally observed.)
General |
Malaise, tiredness, weakness |
Neuropsychiatric |
Impaired concentration, loss of memory, headache, drowsiness, lethargy, disorientation, confusion, irritability, depression, paranoia, hallucinations, ataxia, speech defects, visual disturbances, deafness (calcification of eardrum), pruritus, mental retardation (infants), stupor, coma |
Neuromuscular |
Muscle weakness, hyporeflexia or absent reflexes, hypotonia, myalgia, arthralgia, bone pain, joint effusion, chondrocalcinosis, dwarfism (infants) |
Gastrointestinal |
Loss of appetite, dry mouth, thirst, polydipsia, nausea, vomiting, constipation, abdominal pain, weight loss, acute pancreatitis (calcifying), peptic ulcer, acute gastric dilation |
Renal |
Polyuria, nocturia, nephrocalcinosis, nephrolithiasis, interstitial nephritis, acute and chronic renal failure |
Cardiovascular |
Arrhythmia, bradycardia, first-degree heart block, short Q-T interval, bundle branch block, arrest (rare), hypertension, vascular calcification |
Metastatic Calcification |
Band keratopathy, red eye syndrome, conjunctival calcification nephrocalcinosis, vascular calcification, pruritus |
As many as 10% of patients with elevated levels of serum calcium are detected by a routine screening test of blood chemistry and are considered to have so-called asymptomatic hypercalcemia. However, even very mild hypercalcemia may be of clinical significance inasmuch as some studies have suggested an increased cardiovascular risk from mild but prolonged calcium level elevations.
In symptomatic patients, the spectrum of the clinical presentation is varied and could be nonspecific. Mild hypercalcemia may present with malaise, weakness, minor joint pain, and other vague symptoms. In patients with severe hypercalcemia, the major symptoms are more likely to be nausea, vomiting, constipation, polyuria, and mental disturbances, ranging from headache and lethargy to coma. Recent loss of memory could be prominent and be a presenting symptom.
Hypercalciuria induced by hypercalcemia causes nephrogenic diabetes insipidus, with polyuria and polydipsia leading to ECF volume depletion, decreased glomerular filtration rate (GFR), and further increases in the serum calcium level. The effect of hypercalcemia on urinary concentration is mediated through CaSR activation, which decreases vasopressin-dependent aquaporin-2 (AQP2) water channels trafficking in the inner medullary collecting duct. Nephrolithiasis and nephrocalcinosis are common complications of hypercalcemia, seen in 15% to 20% of cases of primary HPT.
Laboratory Findings
Laboratory findings in patients with hypercalcemia include abnormalities related to the underlying disease causing the hypercalcemia, which are beyond the scope of this chapter. Alterations in the electrocardiogram (ECG) and electroencephalogram (EEG) occur in hypercalcemic patients, independent of the cause of the hypercalcemia. The ECG shows a shortened ST segment and therefore a reduced QT interval as a result of an increased rate of cardiac repolarization. In patients with severe hypercalcemia (>16 mg/dL), there is a widening of the T waves, resulting in an increase in the QT interval. Bradycardia and first-degree heart block may be present in the ECGs of patients with acute and severe hypercalcemia. The EEG displays slowing and other nonspecific changes.
Diagnosis
A careful history, physical examination, and routine laboratory tests will, in most patients, lead to the correct diagnosis in hypercalcemia. A flow diagram for the evaluation of hypercalcemia is shown in Figure 19.1 . Primary HPT (PHPT) and malignancy-associated hypercalcemia together are responsible for 90% of cases of hypercalcemia, with malignancy being the most common cause in hospitalized patients and PHPT being the most common cause in the outpatient clinic.

It is generally easy to differentiate these two entities. Hypercalcemia is only rarely an early finding in occult malignancy. PTH levels are essential in the diagnosis of hypercalcemia. There are two types of assay for PTH, depending on which epitopes of 1-84 PTH are recognized by the antibodies in the assay. Second-generation assays, the immunoradiometric assay (IRMA) and immunochemiluminometric assay (IMCA), use antibodies against 7-34 and 39-84 epitopes of 1-84 PTH. Despite their being called intact PTH assays, implying that they measure only the biologically active PTH, they also detect large carboxy-terminal fragments of PTH, such as PTH (7-84). Thus, they may overestimate the amount of bioactive hormone in serum, especially in CKD patients. The third-generation, whole or biointact PTH assays, use antibodies against 1-5 and C-terminal epitopes and detect biologically active intact PTH. The normal levels of PTH measured by various assays range from 8 to 80 ng/L (1 to 9 pmol/L). Intraoperative PTH measurements are frequently used to assess the adequacy of parathyroidectomy. There is no cross reactivity between PTH and PTHrP assays. In PHPT, levels of PTH can be frankly elevated but can also be in the middle or upper range of normal, particularly in young individuals ( Figure 19.2 ).

The differential diagnosis of patients with hypercalcemia and elevated PTH level includes HPT due to thiazide diuretics or lithium, familial hypocalciuric hypercalcemia (FHH), and the tertiary HPT associated with renal failure and kidney transplantation. Patients with FHH have a positive family history, onset of hypercalcemia at a young age, very low urinary calcium excretion, and specific gene abnormalities. In malignancy-associated hypercalcemia and in hypercalcemia of most other causes, PTH levels are low. The diagnosis of humoral hypercalcemia of malignancy (HHM) frequently can be made on clinical grounds. In addition, PTHrP can now be assayed by commercial clinical laboratories to support HHM or when the cause of hypercalcemia is obscure.
Approximately 10% of cases of hypercalcemia are due to other causes. Of particular importance in the evaluation of a hypercalcemic patient are the family history (because of familial syndromes, including multiple endocrine neoplasia type 1 [MEN1], MEN2, and familial hypocalciuric hypercalcemia), medication history (because of the several medication-induced forms of hypercalcemia), and presence of other disease (e.g., granulomatous or malignant disease). Plasma 1,25(OH) 2 D levels should be measured when granulomatous disorders or 1,25(OH) 2 D lymphoma syndrome is considered. High 25(OH) 2 D levels may suggest vitamin D intoxication as a cause of hypercalcemia.
Causes
Primary Hyperparathyroidism
PHPT is caused by excessive and incompletely regulated secretion of PTH, with consequent hypercalcemia and hypophosphatemia (see Table 19.1 ). It is the underlying cause of approximately 50% of hypercalcemic cases in the general population. The estimated prevalence of PHPT is about 1%, but may be as high as 2% in postmenopausal women. The annual incidence is approximately 0.03% to 0.04%. A single enlarged parathyroid gland (adenoma) is the cause of PHPT in 80% to 85% of cases. These adenomas are benign clonal neoplasms of parathyroid chief cells, which lose their normal sensitivity to calcium. In about 15% to 20% of patients with PHPT, all four parathyroid glands are hyperplastic. This occurs in sporadic PHPT or in conjunction with MEN1 or MEN2. In diffuse hyperplasia, the set point for calcium is not changed in any given parathyroid cell, but the increased number of cells causes excess PTH production and hypercalcemia. Parathyroid carcinoma is seen in no more than 0.5% to 1% of patients with PHPT.
PHPT occurs at all ages but is most common in older individuals; peak incidence is in the sixth decade of life. After age 50 years, women are about three times more frequently affected than men. Sporadic PHPT is the most common. External neck irradiation during childhood is recognized as a risk factor for PHPT. The genetic alterations underlying parathyroid adenomas are being partially elucidated. Rearrangements and overexpression of the PRAD-1/cyclin D1 oncogene have been observed in about 20% of parathyroid adenomas. The MEN1 tumor suppressor gene is inactivated in about 15% of adenomas. Other chromosomal regions may also harbor parathyroid tumor suppressor genes.
PHPT typically presents in one of three ways. In 60% to 80% of cases, there are minimal or no symptoms, and mild hypercalcemia is usually discovered during routine laboratory examination. Another 20% to 25% of patients have a chronic course manifested by mild or intermittent hypercalcemia, recurrent renal stones, and complications of nephrolithiasis; in these patients, the parathyroid tumor is small (<1.0 g) and slow-growing. In 5% to 10% of patients, there is severe and symptomatic hypercalcemia and overt osteitis fibrosa cystica; in these patients, the parathyroid tumor is usually large (>5.0 g). Patients with parathyroid carcinoma typically have severe hypercalcemia, with classic renal and bone involvement.
The diagnosis of PHPT is now usually suggested by the incidental finding of hypercalcemia rather than by any of the sequelae of PTH excess, such as skeletal and renal complications or symptomatic hypercalcemia. Hypercalcemia may be mild and intermittent. Hypercalciuria was noted in 40% of PHPT, nephrolithiasis in 19%, and classic bone disease and osteitis fibrosa cystica only in 2% of studies performed between 1984 and 2000 in the United States. However, even in individuals with mild PHPT, there is also progressive bone loss as measured by bone mineral densitometry over 15 years of observation.
The diagnosis of PHPT is established by laboratory tests showing hypercalcemia, inappropriately normal or elevated blood levels of PTH, hypercalciuria, hypophosphatemia, phosphaturia, and increased urinary excretion of cAMP. Hyperchloremic acidosis may be present, and the ratio of serum chloride to phosphorus is elevated. The serum levels of alkaline phosphatase and of uric acid may be also elevated. The serum concentration of magnesium is usually normal but may be low or high.
Some controversy surrounds the potential relationship between primary HPT and increased mortality. A number of studies have shown that primary HPT may be associated with hypertension, dyslipidemia, diabetes, increased thickness of the carotid artery, and increased mortality, primarily from cardiovascular disease. The morbidity from primary HPT can also be substantial, especially in symptomatic patients with severe hypercalcemia and a late diagnosis.
The classic bone lesion in primary HPT, osteitis fibrosa cystica, is now rarely seen. Diffuse osteopenia is more common. Even in asymptomatic patients, increased rates of bone turnover are always present.
Surgery is still standard therapy for PHPT. It is generally agreed that parathyroidectomy is indicated in all patients with biochemically confirmed PHPT who have specific symptoms or signs of disease, such as a history of life-threatening hypercalcemia, renal insufficiency, and/or kidney stones. In 2008, a Third International Workshop on Hyperparathyroidism updated 2002 National Institutes of Health guidelines for the management of asymptomatic HPT. Surgery is advised for asymptomatic disease in patients with serum calcium levels greater than 1 mg/dL above normal, reduced bone mass (T-score < −2.5 at any site), GFR less than 60 mL/min, or age younger 50 years. Hypercalciuria (>400 mg calcium/24 hours) is no longer regarded as an indication for parathyroid surgery because hypercalciuria in PHPT has not been established as a risk factor for stone formation. Patients older than 50 years with no obvious symptoms should receive close follow-up, including measurements of bone density every 1 to 2 years and serum creatinine and calcium levels annually. All monitored patients should be repleted with vitamin D to achieve a 25-hydroxyvitamin D (25[OH])D level above 20 ng/dL and should maintain calcium intake the same as individuals without PHPT.
Preoperative localization of the parathyroid glands has generally been considered unnecessary in uncomplicated patients undergoing surgery for the first time with bilateral neck exploration. However, imaging studies are recommended to be used in complicated cases and if minimally invasive surgery is planned. Sestamibi scanning is the most popular and sensitive technique to localize PTH glands, with accuracy rates up to 94%, followed by ultrasound of the neck. If a single adenoma is visualized, minimally invasive parathyroidectomy may be an option, with a cure rate of 95% to 98%: this procedure requires the surgeon to visualize only one gland as long as resection results in a substantial intraoperative decline in PTH level. Otherwise, all four parathyroid glands should be surgically identified. Recurrence of HPT is rare after identification and removal of one enlarged gland. If the initial exploration fails, and hypercalcemia persists or recurs, more extensive preoperative parathyroid localization should be performed. Complications are greater with re-exploration of the neck than after the initial operation.
Although parathyroidectomy remains the definitive treatment of PHPT, patients refusing surgery, those with contraindications for surgery, or those who do not meet current operative guidelines can be treated pharmacologically. There are four classes of medications that can be useful—calcimimetics, bisphosphonates, estrogens, and selective estrogen receptor modulators. There are insufficient long-term data to recommend any of these medications as alternatives to surgery. The CaSR agonist, cinacalcet, is approved in some European countries for PHPT and in the United States for severe hypercalcemia in adult patients with PHPT who are unable to undergo parathyroidectomy. Cinacalcet therapy in PHPT patients reduced plasma PTH levels, normalized serum calcium levels in the short and long terms, and preserved bone mineral density (BMD). Bisphosphonates and hormone replacement therapy decreased bone turnover and increased BMD in PHPT patients without change in serum calcium levels.
Parathyroid carcinoma probably accounts for less than 1% of PHPT cases. The diagnosis of parathyroid carcinoma may be difficult to make in the absence of metastases because the histologic appearance may be similar to that of atypical adenomas. In general, parathyroid carcinomas are typically large (3 cm), irregular, hard tumors with a low degree of aggressive growth, and survival is common if the entire gland can be removed. Cinacalcet is approved for patients with inoperable parathyroid cancer to control hypercalcemia.
Malignancy
Hypercalcemia occurs in approximately 10% to 25% of patients with some cancer, especially during the last 4 to 6 weeks of their life. It can be classified into four categories—HHM, local osteolytic hypercalcemia (LOH), 1,25 (OH) 2 vitamin D−induced hypercalcemia, and ectopic secretion of authentic PTH.
HHM from secretion of PTHrP by a malignant tumor accounts for approximately 80% of cases. Numerous types of malignancies are associated with HHM, including squamous cell cancer (e.g., head and neck, esophagus, cervix, lung) and renal cell, breast, and ovarian carcinomas. Lymphomas associated with human T-lymphotropic virus type 1 (HTLV-1) infection may cause PTHrP-mediated HHM, and other non-Hodgkin lymphomas may also be associated with PTHrP-mediated hypercalcemia. PTHrP is a large protein encoded by a gene on chromosome 12; it is similar to PTH only at the NH 2 terminus, where the initial eight amino acids are identical. PTHrP is widely expressed in a variety of tissues, including keratinocytes, mammary gland, placenta, cartilage, nervous system, vascular smooth muscle, and various endocrine sites. Injection of PTHrP produces hypercalcemia in rats and essentially reproduces the entire clinical syndrome of HHM, but other circulating factors such as cytokines may also be important. Normal circulating levels of PTHrP are negligible; these are probably unimportant in normal calcium homeostasis. However, mice with a targeted disruption in the PTHrP gene have shown a lethal defect in bone development, thus demonstrating its importance in normal development.
Circulating PTHrP interacts with the PTH/PTHrP receptor in bone and the renal tubule. It activates bone resorption and suppresses osteoblastic bone formation, thus causing flux of calcium (up to 700 to 1000 mg/day) from bone into ECF. The reason for this uncoupling of bone formation from bone resorption remains unclear. One possible explanation is a difference in the affinity of PTH versus that of PTHrP for the PTH receptor on osteoblasts. PTHrP mimics the anticalciuric effect of PTH on the kidney, which exacerbates hypercalcemia. Other effects of PTHrP include phosphaturia, hypophosphatemia, and increased cAMP excretion by the kidney.
HHM is associated with a reduction in 1,25(OH) 2 D levels (in contrast to PHPT), which may limit intestinal calcium absorption. Patients are hypercalcemic and hypophosphatemic and demonstrate increased osteoclastic bone resorption, increased urinary cAMP, and hypercalciuria.
LOH accounts for 20% of patients with malignancy-associated hypercalcemia. LOH-producing tumors include breast and prostate cancers and hematologic neoplasms (e.g., multiple myeloma, lymphoma, leukemia). LOH is caused by locally produced osteoclast-activating cytokines, which include PTHrP, interleukin-1 (IL-1), IL-6, and IL-8. PTHrP increases RANKL expression on osteoblast and RANK-mediated osteoclast bone resorption. The resorbing bone releases transforming growth factor-β (TGF-β), which in turn stimulates PTHrP expression on tumor cells. The bone metastases can be classified as osteolytic, osteoblastic, or mixed. Osteolytic lesions are caused by osteoclast activation by malignant cells and appear as areas of increased radiolucency on radiographs. LOH leads to predictable pathophysiologic events, which include hypercalcemia, suppression of circulating PTH and 1,25(OH) 2 D, hyperphosphatemia, and hypercalciuria. Bone metastases may produce severe pain and pathologic fractures.
Hypercalcemia in breast cancer is associated with the presence of extensive osteolytic metastases and HHM. Extensive osteolytic bone destruction is seen in multiple myeloma. Although bone lesions develop in all patients with myeloma, hypercalcemia occurs only in 15% to 20% of patients in later stages of disease and with impaired kidney function. The degree of hypercalcemia and bone destruction is not well correlated. Treatment with bisphosphonates appears to protect against the development of skeletal complications (including hypercalcemia) in patients with myeloma and lytic bone lesions.
Hypercalcemia caused by 1,25 (OH) 2 vitamin D production by malignant lymphomas has been reported. All types of lymphoma can cause this syndrome. The malignant cells or adjacent cells overexpress the enzyme 1α-hydroxylase, which converts 25(OH)D to 1,25(OH) 2 D. Hypercalcemia is mainly secondary to increased intestinal calcium, although decreased renal clearance and bone resorption may also develop. In addition, increased osteoclastic activity mediated through activation of the RANKL pathway by 1,25(OH)2D augment hypercalcemia. Ectopic production of authentic PTH by a nonparathyroid tumor may occur, but is very rare.
Familial Primary Hyperparathyroidism Syndromes
Familial primary HPT syndromes are defined by a combination of hypercalcemia and elevated or nonsuppressed serum PTH levels.
Familial Hypocalciuric Hypercalcemia and Neonatal Severe Hyperparathyroidism.
FHH (benign) is a rare disease (estimated prevalence, 1/78,000), with autosomal dominant inheritance, high penetrance for hypercalcemia, and relative hypocalciuria. FHH was first described in 1966 by Jackson and Boonstra and in 1972 by Foley and colleagues. The hypercalcemia is typically mild to moderate (10.5 to 12 mg/dL), and affected patients do not exhibit the typical complications associated with elevated serum calcium concentrations. Both total and ionized calcium concentrations are elevated, but the PTH level is generally inappropriately normal, although mild elevations in approximately 15% to 20% of cases have been reported. Urinary calcium excretion is not elevated, as would be expected in hypercalcemia of other causes. The fractional excretion of calcium is usually less than 1%. The serum magnesium level is commonly mildly elevated, and the serum phosphate level is decreased. Bone mineral density is normal, as are vitamin D levels. The finding of a right-shifted set point for Ca 2+ -regulated PTH release in FHH has indicated the role of CaSR in FHH.
Most families have FHH type 1, which is caused by autosomal dominant loss-of-function mutations in the CaSR gene located on chromosome 3q, which encodes for the CaSR. FHH types 2 and 3 are much rarer and are caused by heterozygous mutations in the GNA11 (guanidine nucleotide-binding protein alpha-11) gene or the AP2S1 (adaptor-related protein complex 2, sigma 1 subunit) gene, respectively. Both genes localize on chromosome 19q13, and their mutations render the CaSR less sensitive to extracellular calcium.
The fact that relative hypocalciuria persists even after parathyroidectomy in FHH patients confirms the role of CaSR in regulating renal calcium handling. More than 257 mutations have been described for the CaSR, most of which are inactivating and missense and found throughout the large predicted structure of the CaSR protein. Expression studies of mutant CaSRs have shown great variability in their effect on calcium responsiveness. In some cases, CaSR mutations only slightly shift the set point for calcium; other mutations appear to render the receptor largely inactive. CaSR mutation analysis has an occasional role in the diagnosis of FHH in cases in which biochemical test results remain inconclusive, and the distinction of FHH from mild primary HPT is unclear. It is critically important to make an accurate diagnosis because the hypercalcemia in FHH is benign and does not respond to subtotal parathyroidectomy.
Hypercalcemia in FHH has a generally benign course and is resistant to medications, except it is successfully treated with cinacalcet in some patients. Potential benefit of calcimimetic agents in FHH has been supported by in vitro studies with human CaSR mutants, which have shown that the calcimimetic agent R-568 enhances the potency of extracellular calcium toward the mutants.
Patients who inherit two copies of CaSR alleles bearing inactivating mutations develop neonatal severe HPT (NSHPT). NSHPT is an extremely rare disorder that is often reported in the offspring of consanguineous FHH parents; it is characterized by severe hyperparathyroid hyperplasia, PTH elevation, severe hyperparathyroid bone disease, and elevated extracellular calcium levels. In a few affected infants, only one defective allele has been found, but it is unclear whether this finding is due to the presence of an undetected defect in the other CaSR allele. Treatment is total parathyroidectomy, followed by vitamin D and calcium supplementation. This disease is usually lethal without surgical intervention.
Multiple Endocrine Neoplasia.
MEN1 is a rare, autosomal dominant disorder with an estimated prevalence of two to three cases/100,000, characterized by endocrine tumors in at least two of three main tissues—parathyroid gland, pituitary gland, and enteropancreatic tissue. It is the most common form of familial PHPT. PHPT is present in 87% to 97% of patients, whereas pancreatic and pituitary tumors are more likely to be absent. The responsible gene, MEN1, encodes a nuclear protein, menin, of 610 amino acids, which functions in cell division, genome stability, and transcription regulation.
MEN2A is a syndrome of heritable predisposition to medullary thyroid carcinoma, pheochromocytoma, and PHPT. Mutations in the RET proto-oncogene, which encodes a tyrosine kinase receptor, are responsible for MEN2A. The biochemical diagnosis and indications for surgery for patients with PHPT in association with MEN1 or MEN2A are similar to those for sporadic PHPT.
Hyperparathyroidism–Jaw Tumor Syndrome.
Hyperparathyroidism–jaw tumor (HPT-JT) syndrome is a rare autosomal dominant disorder characterized by severe hypercalcemia, parathyroid adenoma, and fibro-osseous tumors of mandible or maxilla. Renal manifestations include cysts, hamartomas, and Wilms’ tumors. Mutations in the HRPT2 (hyperparathyroidism 2) gene, and elimination of its product, parafibromin, which has tumor suppressor activity, are responsible for HPT-JT. If biochemical changes consistent with PHPT are present, parathyroidectomy is indicated.
Nonparathyroid Endocrinopathies
Hypercalcemia may occur in patients with other endocrine diseases. Mild hypercalcemia is present in up to 20% of the patients with hyperthyroidism, but severe hypercalcemia is uncommon. Thyroid hormones (thyroxine, triiodothyronine) increase bone resorption and lead to hypercalcemia and/or hypercalciuria when bone resorption exceeds bone formation significantly. Because of a possible increased association between hyperthyroidism and parathyroid adenoma, the latter must be ruled out in patients with thyrotoxicosis and hypercalcemia. Furthermore, the hypercalcemia in a patient with thyrotoxicosis should be attributed to this disease only if it resolves after achieving an euthyroid state.
Pheochromocytoma may be associated with hypercalcemia ; it is usually caused by coincident PHPT and MEN2A. In some patients, hypercalcemia disappears after removal of the adrenal tumor, and some of these tumors produce PTHrP. Acute adrenal insufficiency is a rare cause of hypercalcemia. Because these patients may be dehydrated and have hemoconcentration, a rise in the serum albumin concentration and increased binding of calcium to serum albumin secondary to hyponatremia may contribute to the increase in serum calcium levels. In addition, isolated adrenocorticotropic hormone (ACTH) deficiency can result in hypercalcemia.
Growth hormone administration and acromegaly have both been associated with hypercalcemia. Acromegaly is often (15% to 20% of cases) accompanied by mild hypercalcemia, which results from enhanced intestinal calcium absorption and augmented bone resorption. In acromegalic patients with hypercalcemia, the serum levels of PTH are normal but may be inappropriately high for the levels of serum calcium.
Vitamin D–Mediated Hypercalcemia
Vitamin D is naturally generated in skin under exposure to ultraviolet B (UVB) light or is acquired from the diet and medical supplements. Excess of vitamin D or its metabolites can cause hypercalcemia and hypercalciuria. The mechanism of hypercalcemia is a combination of increased intestinal calcium absorption and bone resorption induced by vitamin D and decreased renal calcium clearance resulting from dehydration. The effect of toxic amounts of vitamin D is due to an increase in plasma total 25(OH)D, well in excess of 80 ng/mL, which exceeds the binding capacity of vitamin D–binding protein (DBP) for 25(OH)D. The resulting increase in free circulating 25(OH)D may activate the vitamin D nuclear receptor (VDR). Vitamin D metabolites may also displace 1α,25(OH) 2 D from DBP, increasing free 1α,25(OH) 2 D levels and thus increasing signal transduction.
Hypercalcemia has been reported in accidental overdoses of vitamin D from fortified cow’s milk, consumption by children of a fish oil with manufacturing error that caused an excess of vitamin D, and over-the-counter supplements. Serum 25(OH)D levels were elevated, 1,25(OH) 2 D levels were normal, and PTH levels were depressed or normal in these settings. However, vitamin D well in excess of the tolerable upper intake of 2000 IU/day is required for this form of hypercalcemia to develop. Polymorphism in genes that regulate vitamin D metabolism may predispose certain individuals to develop toxicity, even with exposure to small vitamin D doses. The diagnosis is made by the history and detection of elevated 25(OH)D levels. In the syndrome of idiopathic infantile hypercalcemia, a defect in degradation of 1,25(OH) 2 D to 24,25(OH) 2 D caused by a CYP24A1 loss-of-function mutation is responsible for extremely high levels of 1,25(OH) 2 D and hypercalcemia. Vitamin D analogues, including 1,25(OH) 2 D used in the treatment of HPT and metabolic bone disease in CKD patients, can also cause hypercalcemia.
Medications
Hypercalcemia and HPT is a long-recognized, well-described consequence of lithium therapy. The prevalence of lithium-associated hypercalcemia is estimated to be 4% to 6%. Lithium probably interferes with signal transduction elicited by the CaSR, which increases the set point for extracellular calcium to inhibit PTH secretion. This leads to parathyroid hyperplasia or adenoma. The spectrum of lithium-induced calcium disorders is wide and includes patients with overt HPT and mild or severe hypercalcemia, with or without elevated PTH levels. Hypocalciuria is common, although hypercalciuria was reported in a few case series. Hypercalcemia can be reversible after a few weeks of discontinuing lithium in most patients with short lithium treatment (<5 years). The CaSR agonist cinacalcet was also used with good results in those patients when cessation of lithium therapy was not an option. Symptomatic patients with HPT should be treated with parathyroidectomy.
Vitamin A intake in doses exceeding the recommended daily allowance over prolonged periods, especially in older adults and patients with impaired kidney function, may cause hypercalcemia, with increased alkaline phosphatase levels, presumably from increased osteoclast-mediated bone resorption. The hypercalcemia is accompanied by high retinol plasma levels, and discontinuation of vitamin A caused normalization of plasma calcium levels. Vitamin A analogues, used in the management of dermatologic and hematologic malignant diseases, have also been reported to cause hypercalcemia.
Estrogens and selective estrogen receptor modifiers (e.g., tamoxifen) used in the management of breast cancer may cause hypercalcemia early during treatment, even in the presence of bone metastasis.
Thiazide diuretic–associated hypercalcemia was noted in approximately 0.4% to 1.9% of treated subjects, with an annual incidence rate of 7.7/100,000 in the population of Olmsted County, Minnesota. A reduction in urinary calcium excretion, volume contraction, and metabolic alkalosis are the major reasons for thiazide-induced hypercalcemia. Also, thiazides may increase intestinal calcium absorption and reveal primary HPT. Hypercalcemia is usually mild, asymptomatic, and nonprogressive. Subjects with unsuppressed PTH levels, despite hypercalcemia or with severe and continued hypercalcemia, may have primary HPT.
Many other medications occasionally cause hypercalcemia, including theophylline, foscarnet, growth hormone, parenteral nutrition, manganese in toxic doses, and 8-chloro-cAMP.
Milk-Alkali Syndrome
Milk-alkali syndrome was originally described in patients with duodenal ulcers receiving therapy with sodium bicarbonate and large amounts of milk. These patients have hypercalcemia, hyperphosphatemia, hypocalciuria, and renal insufficiency, together with kidney and other soft tissue calcifications. During the last 20 years, calcium supplements in the form of calcium carbonate for the prevention and treatment of osteoporosis have become the main cause of this syndrome. In the most recent literature, the name calcium-alkali syndrome was proposed. In some studies, milk-alkali syndrome was the third most common cause of hypercalcemia in non–end-stage kidney disease (ESKD) hospitalized patients. The pathogenesis of milk-alkali syndrome can be divided into two phases, the generation of hypercalcemia by intake of calcium and the maintenance phase. Usually, oral intake of more than 4 g of elemental calcium/day has been reported, but even 2 g of calcium/day, especially if taken together with vitamin D, may induce this syndrome. Hypercalcemia activates the renal CaSR, causing natriuresis and water diuresis, with volume depletion and a decrease in the GFR. Increased tubular reabsorption of calcium as a result of metabolic alkalosis and volume depletion contribute to the maintenance of hypercalcemia. The diagnosis is made largely by the history and may not be obvious because of atypical dietary sources of calcium and alkali. Hypercalcemia can be corrected, but renal damage may be permanent.
Immobilization
Immobilization, especially in high bone turnover states (e.g., in young people, hyperparathyroidism, breast cancer with bone involvement, Paget’s disease), suppresses osteoblastic bone formation and increases osteoclastic bone resorption, leading to uncoupling of these two processes, with subsequent release of calcium from the bone and hypercalcemia. Typically, it takes from 10 days to a few weeks for the development of immobilization hypercalcemia. Increased sclerostin production by osteocytes during mechanical unloading and disuse of the bone is implicated in the pathogenesis of hypercalcemia. Sclerostin is a glycoprotein that inhibits Wnt/β catenin signaling in the osteoblast and decreases bone formation. It is of interest that antisclerostin antibodies are being studied for the treatment of osteoporosis. Bisphosphonates may help decrease hypercalcemia and osteopenia in the setting of immobilization-induced hypercalcemia. There are case reports showing that denosumab also can correct hypercalcemia of immobilization. Mobilization remains the ultimate cure for this condition.
Granulomatous Disease
A variety of granulomatous diseases are associated with hypercalcemia. The most common is sarcoidosis (prevalence of hypercalcemia and hypercalciuria of 10% and 20%, respectively), but tuberculosis, berylliosis, histoplasmosis, coccidioidomycosis, pneumocystosis, leprosy, histiocytosis X, eosinophilic granulomatosis, and inflammatory bowel disease may present with hypercalcemia. Hypercalcemia is more common in chronic and disseminated granulomatous diseases. Sun exposure, or even small doses of vitamin D supplementation, may precipitate or worsen this syndrome. The hypercalcemia, which has been best studied in sarcoidosis, is caused by inappropriate extrarenal production of 1,25(OH) 2 D by activated macrophages with increased 1α-hydroxylase activity. Elevated circulating 1,25(OH) 2 D levels have been described in most granulomatous diseases during hypercalcemia, except in coccidioidomycosis. The 1,25(OH) 2 D in turn leads to intestinal hyperabsorption of calcium, hypercalciuria, and hypercalcemia. Osteopontin, highly expressed by histiocytes in granulomas, may contribute to hypercalcemia via osteoclast activation and bone resorption. Bone mineral content tends to be reduced in these patients. Hypercalciuria may precede hypercalcemia and may be an early indicator of this complication.
Standard treatment consists of administration of glucocorticoids, which decreases the abnormal 1,25(OH) 2 D production. Chloroquine and ketoconazole, which also decrease 1,25(OH) 2 D production by competitive inhibition of CYP450-dependent 1α-hydroxylase, have also been shown to be efficacious.
Liver Disease
Hypercalcemia has been reported in patients with end-stage liver disease with hyperbilirubinemia awaiting liver transplantation in the absence of HPT or hypervitaminosis D.
Acute and Chronic Kidney Disease
Hypercalcemia may be observed in patients with certain forms of acute and chronic kidney disease and in kidney transplant recipients. These conditions are discussed in detail in Chapter 55 .
Management of Hypercalcemia
The optimal therapy for hypercalcemia must be tailored to the degree of hypercalcemia, clinical condition, and underlying cause ( Table 19.3 ). Theoretically, a decrease in serum calcium levels can be achieved by enhancing its urinary excretion, augmenting net movement of calcium into bone, inhibiting bone resorption, reducing intestinal absorption of calcium, and/or removing calcium from the ECF by other means. Patients with mild hypercalcemia (<12 mg/dL) do not require immediate treatment. They should discontinue any medications implicated in causing hypercalcemia, avoid volume depletion and physical inactivity, and maintain adequate hydration. Moderate hypercalcemia (12 to 14 mg/dL), especially if acute and symptomatic, requires more aggressive therapy. Patients with severe hypercalcemia (>14 mg/dL), even without symptoms, should be treated intensively.
Intervention | Dose | Adverse Effect |
---|---|---|
Hydration or Calciuresis | ||
Intravenous saline | 200-500 mL/hr, depending on patient’s cardiovascular and renal status | Congestive heart failure |
Furosemide | 20-40 mg IV (after rehydration has been achieved) | Dehydration, hypokalemia, hypomagnesemia |
First-Line Medications | ||
IV bisphosphonates † | ||
Pamidronate | 60-90 mg IV over 2 hr in 50-200 mL saline solution or 5% dextrose in water § | Renal failure, transient flulike syndrome with aches, chills, and fever |
Zoledronate | 4 mg IV over 15 min in 50 mL of saline solution or 5% dextrose in water | Renal failure, transient flulike syndrome with aches, chills, and fever |
Second-Line Medications | ||
Glucocorticoids ‡ | Example: prednisone, 60 mg orally daily, for 10 days | Potential interference with chemotherapy; hypokalemia, hyperglycemia, hypertension, Cushing’s syndrome, immunosuppression |
Mithramycin | Single dose of 25 µg/kg of body weight over 4-6 hr in saline | Thrombocytopenia, platelet aggregation defect, anemia, leukopenia, hepatitis, renal failure ∥ |
Calcitonin | 4-8 IU/kg subcutaneously or intramuscularly every 12 hr | Flushing, nausea, escape phenomenon |
Gallium nitrate | 100-200 mg/m 2 of body surface area IV given continuously over 24 hr for 5 days | Renal failure |
Denosumab ¶ | 120 mg on days 1, 8, 15, and 29 and every 4 wk | Hypocalcemia, hypophosphatemia, osteonecrosis of the jaw, atypical femoral fractures |
* Many recommendations in this table are based on historical precedent and common practice rather than on randomized clinical trials. There are data from randomized trials comparing bisphosphonates to the other agents listed and to one another.
† Pamidronate and zoledronate are approved by the FDA. Ibandronate and clodronate are available in continental Europe, United Kingdom, and elsewhere. Bisphosphonates should be used with caution, if at all, when the serum creatinine level exceeds 2.5 to 3.0 mg/dL (221.0 to 265.2 µmol/L).
‡ Pamidronate is generally used at a dose of 90 mg, but the 60-mg dose may be used to treat patients of small stature or those with renal impairment or mild hypercalcemia.
§ These drugs have a slow onset of action, as compared with bisphosphonates; approximately 4 to 10 days are required for a response.
∥ These effects have been reported in association with higher dose regimens used to treat testicular cancer (50 µg/kg body weight/day over a period of 5 days) and in patients receiving multiple doses of 25 µg/kg; they are not expected to occur with a single dose of 25 µg/kg unless preexisting liver, kidney, or hematologic disease is present.
¶ Approved by the FDA for the prevention of skeletal-related events in patients with bone metastasis from solid tumor; used in an open label fashion as a rescue therapy if bisphosphonates are not effective.
Volume Repletion and Loop Diuretics
Correction of the ECF volume is the first and most important step in the treatment of severe hypercalcemia from any causes. It can be achieved with a normal isotonic saline infusion at 200 to 500 mL/hour, adjusted to obtain a urine output of 150 to 200 ml/hour and with appropriate hemodynamic monitoring. Volume repletion can lower the calcium concentration by approximately 1 to 3 mg/dL by increasing GFR and decreasing sodium and calcium reabsorption in the proximal and distal tubules.
Once volume expansion is achieved, loop diuretics can be given concurrently with saline to increase the calciuresis by blocking the Na-K-2Cl cotransporter in the TAL. Usually, furosemide is given at a dose of 40 to 80 mg every 6 hours and this, together with saline therapy, may decrease the serum calcium concentration by 2 to 4 mg/dL. Urinary losses of fluid, potassium, and magnesium should be evaluated at intervals of 2 to 4 hours and quantitatively replaced to prevent dehydration, hypokalemia, and hypomagnesemia. Usually, 20 to 40 mEq of KCl and 15 to 30 mg of magnesium ion/L of saline infusate are adequate to replenish the urinary losses of these electrolytes. Care must be taken to monitor the patient’s volume status closely during the administration of large amounts of saline and diuretic, particularly in hospitalized patients with cardiac or pulmonary disease. It must be noted that the use of loop diuretics for hypercalcemia is not supported by any randomized controlled studies and has been criticized for this reason. However, in our opinion, loop diuretics still remain an important tool in the management of hypercalcemia, especially for patients at risk of volume overload.
Inhibition of Bone Resorption
The increase in bone resorption, as the most common pathology leading to hypercalcemia, must be addressed concurrently with volume expansion and hydration. Bisphosphonates are currently the agents of choice in the treatment of mild to severe hypercalcemia, especially that associated with cancer and vitamin D toxicity. They are pyrophosphate analogues with a high affinity for hydroxyapatite and inhibit osteoclast function in areas of high bone turnover. The U.S. Food and Drug Administration (FDA) has approved two bisphosphonates for the treatment of hypercalcemia, zoledronate (4 mg intravenous [IV] over 15 minutes or longer) and pamidronate (60 to 90 mg IV over 2 to 24 hours). The clinical response takes 48 to 96 hours and is sustained for up to 3 weeks. Doses can be repeated no sooner than every 7 days. Both agents are effective in lowering calcium levels. Zoledronate was slightly more efficacious than pamidronate in a randomized clinical trial. In Europe, other bisphosphonates, such as clodronate and ibandronate, have also been approved.
Fever is observed in about 20% of patients taking bisphosphonates; rare side effects include acute renal failure, collapsing glomerulopathy, and osteonecrosis of the jaw. Ibandronate seems to have minimal to no renal toxicity. The dose of bisphosphonates should be adjusted in patients with preexisting kidney disease. The renal component of hypercalcemia, which includes increased distal tubular calcium reabsorption driven by PTH-PTHrP, does not respond to bisphosphonates.
Calcitonin is also an effective inhibitor of osteoclast bone resorption. It has a rapid onset (within 12 hours), its effect is transient, and it has minimal toxicity. Calcitonin is usually given as 4 to 8 U/kg subcutaneously every 6 to 12 hours. Its role is mainly in the initial treatment of severe hypercalcemia while waiting for the more sustained effect of bisphosphonates.
Gallium nitrate inhibits bone resorption by increasing the solubility of hydroxyapatite crystals. The usual dose is 200 mg/m 2 IV over 24 hours, with adequate hydration for 5 consecutive days; the hypocalcemic effect is not generally observed until the end of this period. Gallium nitrate is effective, but can be nephrotoxic.
Denosumab, a fully humanized monoclonal antibody that binds to RANKL and inhibits osteoclasts. Denosumab inhibits the maturation of preosteoclasts to osteoclasts by binding to and inhibiting RANKL. In 2010, denosumab was approved by the FDA for use in postmenopausal women at risk for osteoporosis. It was also approved for the prevention of skeleton-related events in patients with bone metastasis from a solid tumor. It has also been used for the treatment of hypercalcemia of malignancy not corrected by bisphosphonates in an open label study.
Plicamycin (Mithramycin), 25 µg/kg IV, every 5 to 7 days, may be used in patients with severe renal failure. Other therapies for hypercalcemia, such as chelation with ethylenediaminetetraacetic acid (EDTA) and IV phosphate, have adverse side effect profiles and are no longer recommended.
Glucocorticoids are useful therapy for hypercalcemia in a specific subset of causes. They are most effective in hematologic malignancies (e.g., multiple myeloma, Hodgkin’s disease) and disorders of vitamin D metabolism (e.g., granulomatous disease, vitamin D toxicity).
In severely hypercalcemic patients who are comatose with changes in the ECG, in severe renal failure, or who cannot receive aggressive hydration, hemodialysis with a low- or no-calcium dialysate is an effective treatment. Continuous renal replacement therapy can also be used to treat severe hypercalcemia. The effect of dialysis is transitory and needs to be followed by other measures.
As discussed above, cinacalcet, an allosteric activator of CaSR, is approved for patients with inoperable parathyroid cancer to control hypercalcemia. The off-label use of cinacalcet has been reported in patients with PHPT who have mild disease, failed parathyroid surgery, or contraindications to surgery. Other hypercalcemic disorders, such as FHH and lithium-induced HPT, have also been treated with cinacalcet.
Hypocalcemia
Hypocalcemia is usually defined as a total serum calcium concentration, corrected for protein, of less than 8.4 mg/dL and/or an ionized calcium level less 1.16 mmol/L, although these values may vary slightly, depending on the laboratory. Estimation of the ionized calcium concentration based on the total serum calcium corrected for albumin is encumbered with errors, as discussed in the introduction. Thus, ionized calcium should be directly measured before a major workup for the causes of hypocalcemia is undertaken.
Hypocalcemia is highly prevalent in hospitalized patients (10% to 18%) and is particularly common in the intensive care unit (70% to 80%).
Signs and Symptoms
Acute hypocalcemia can result in severe clinical symptoms that need rapid correction, whereas chronic hypocalcemia may be an asymptomatic laboratory finding. The clinical features of hypocalcemia are summarized in Table 19.4 . Their presentation reflects the absolute calcium concentration and the rapidity of its fall. The threshold for overt symptoms depends also on serum pH and the severity of any concurrent hypomagnesemia, hyponatremia, or hypokalemia. The classic symptoms of hypocalcemia include neuromuscular excitability in the form of numbness, circumoral tingling, feeling of pins and needles in the feet and hands, muscle cramps, carpopedal spasms, laryngeal stridor, and frank tetany. Tapping over the facial nerve anterior to the ear can induce facial muscle spasm (Chvostek’s sign). However, Chvostek’s sign may occur in 10% of normal people, and it was negative in 29% of patients with mild hypocalcemia. Trousseau’s sign of main d’accoucheur , elicited by inflation of a sphygmomanometer cuff placed on the upper arm to 10 mm Hg above systolic blood pressure for 3 minutes, has greater than 90% sensitivity and specificity. Patients with hypocalcemia may experience emotional disturbances, irritability, impairment of memory, confusion, delusion, hallucination, paranoia, and depression. Epileptic seizures, often Jacksonian, may occur but are usually not associated with aura, loss of consciousness, and incontinence. Patients with chronic hypocalcemia, including those with idiopathic and postsurgical hypoparathyroidism and those with pseudohypoparathyroidism, may have papilledema, elevated cerebrospinal fluid pressure, and neurologic signs simulating those of a cerebral tumor.
Neuromuscular Irritability |
|
Altered Central Nervous System Function |
|
Cardiovascular |
|
Dermatologic and Ocular |
|
Bilateral cataracts affecting the anterior and posterior subcapsular areas of the cortical portions of the lens may develop after 1 year of hypocalcemia. The cataracts do not resolve after correction of the hypocalcemia. In patients with idiopathic hypoparathyroidism, the skin could be dry and scaly, eczema and psoriasis may worsen, and candidiasis can occur. The eyelashes and eyebrows may be scanty, and axillary and pubic hair may be absent. Because some forms of this disease have an autoimmune cause, manifestations of other autoimmune diseases, such as adrenal, thyroid, and gonadal insufficiency, diabetes mellitus, pernicious anemia, vitiligo, and alopecia areata may be present and should be sought.
Long-lasting hypocalcemia in children and adults can cause congestive heart failure caused by cardiomyopathy, which is reversible with correction of the calcium. Prolongation of the QTc interval on the ECG is a well-known effect of hypocalcemia on heart conduction.
Hypoparathyroidism in children often causes teeth abnormalities, such as defective enamel and root formation, dental hypoplasia, or failure of adult teeth to erupt. Severe skeletal mineralization may occur in the fetus of untreated pregnant women with hypoparathyroidism and hypocalcemia.
Laboratory Findings
It is important to establish the diagnosis of hypocalcemia, based not only on the measurement of total calcium with proper adjustment to albumin and pH levels, but with evidence that ionized calcium is low. The alterations in serum PTH and serum and urinary electrolyte levels in various hypocalcemic states depend on the mechanisms responsible for the hypocalcemia (see Figure 19.2 ), and knowledge of these changes aids in the differential diagnosis of these disorders.
X-ray examination of the skull or computed axial tomography scanning of the brain may reveal intracranial calcifications, especially of the basal ganglia. These have been noted in up to 20% of hypocalcemic patients with idiopathic hypoparathyroidism but are less common in postsurgical hypoparathyroidism unless the disease is long-standing. Such calcifications are also encountered in patients with pseudohypoparathyroidism. Bone disease may be observed, but its findings differ in the various causes of hypocalcemia (see later).
Diagnosis
The most common causes of hypocalcemia in the nonacute setting are hypoparathyroidism, hypomagnesemia, renal failure, and vitamin D deficiencies ( Table 19.5 ). These entities should be considered early in the diagnosis of hypocalcemic individuals. It is conceptually and clinically useful to subclassify hypocalcemic individuals into those with elevated PTH levels and those with subnormal or inappropriately normal PTH concentrations, as in primary hypoparathyroidism ( Figure 19.3 ). A thorough medical history and physical examination are diagnostically important because hypocalcemia can be caused by postsurgical, pharmacologic, inherited, developmental, and nutritional problems, in addition to being part of complex syndromes.
|

Causes
The causes of hypocalcemia are summarized in Table 19.5 . They can be broadly classified into one of three categories—PTH-related (hypoparathyroidism and pseudohypoparathyroidism), vitamin D–related (low production, vitamin D resistance), and miscellaneous causes.
Parathyroid Hormone–Related Disorders: Hypoparathyroidism and Pseudohypoparathyroidism
This group of disorders presents with hypocalcemia and hyperphosphatemia caused by failure of the parathyroid gland to secrete adequate amounts of biologically active PTH or resistance to PTH action at the tissue level. Both can be inherited or acquired. The levels of PTH are low or absent in hypoparathyroidism (HP) due to lack of PTH production, but elevated in pseudohypoparathyroidism (PHP) due to a secondary or adaptive increase in PTH secretion. The fractional calcium excretion is elevated in HP and low in PHP. Insufficient 1,25(OH) 2 D is generated for efficient intestinal calcium absorption because of decreased activity of 1α-hydroxylase in the proximal tubules. Skeletal response in both categories is appropriate to the levels of circulating PTH, with low bone turnover in HP and excessive bone remodeling in PHP. Hypoparathyroidism is a rare disorder. One study from Japan found the prevalence to be 7.2/million people.
Genetic Causes of Hypoparathyroidism.
At least four different mutations affecting the PTH gene(s) involved in parathyroid development have been identified as a cause of familial isolated HP ( Table 19.6 ). All these conditions present during the neonatal period with severe hypocalcemia without any other organ involvement and respond well to therapy with vitamin D analogues.
Reference | Syndrome | Other Clinical Features | Process Affected | Inheritance | Gene Mutated | Syndrome OMIM No. |
---|---|---|---|---|---|---|
Ding et al | Familial isolated hypoparathyroidism | None | Parathyroid gland development | AR | GCM2 | 146200 |
Bowl et al | X-linked | SOX3? | 307700 | |||
Parkinson and Thakker | PTH gene mutation affecting its synthesis | AR | Prepro-PTH splice site | |||
Arnold et al | AD | Prepro-PTH signal peptide | ||||
Pollak et al | Autosomal dominant hypoparathyroidism | Hypocalcemia, hypomagnesemia, hypercalciuria | Calcium sensing | AD | CaSR | 146200 601298 |
Yagi et al | DiGeorge, CATCH22 | Cardiac anomalies, abnormal facies, thymic aplasia, cleft palate | Defective third and fourth branchial pouch development | Sporadic or AD | Chromosome 22q11 deletions (including TBX1) | 188400 |
Van Esch et al | HDR | Hypoparathyroidism, deafness, renal anomalies | Parathyroid development | AD | GATA3 transcription factor | 146255 |
Parvari et al | Kenny-Caffey, Sanjad-Sakati | Microcephaly, mental retardation, growth failure ± osteosclerosis | AR | TBCE (chaperone for tubulin folding) | 241410 244460 | |
Neufeld et al | Autoimmune polyendocrinopathy—candidiasis—ectodermal dystrophy (APECED) | Chronic mucocutaneous candidiasis, Addison’s disease | Immune tolerance | AR | AIRE (autoimmune transcriptional regulator) | 240300 |
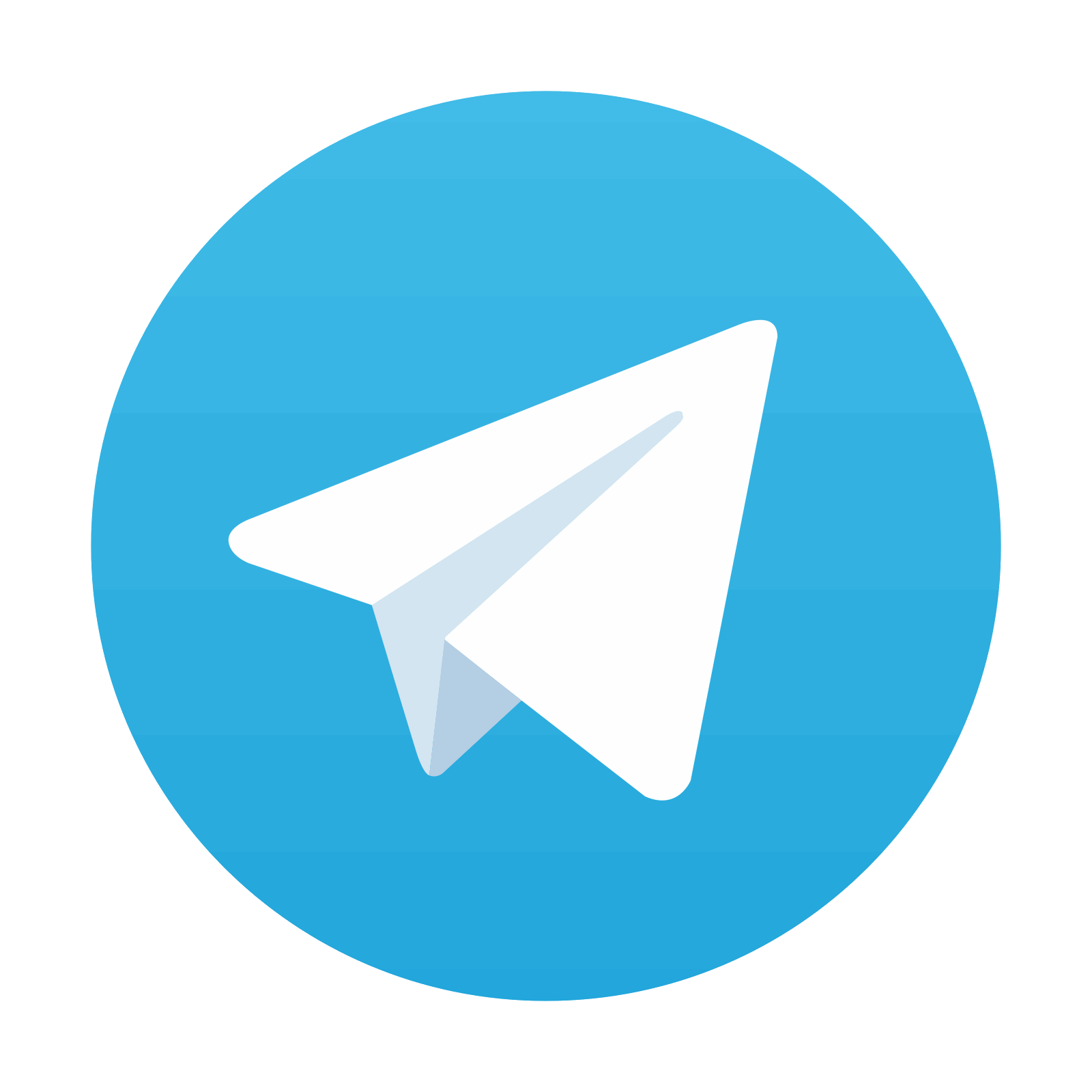
Stay updated, free articles. Join our Telegram channel

Full access? Get Clinical Tree
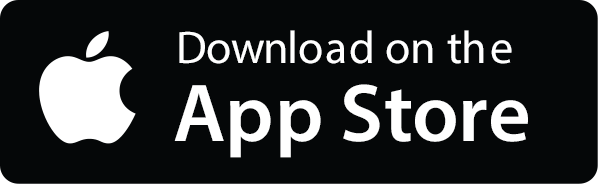
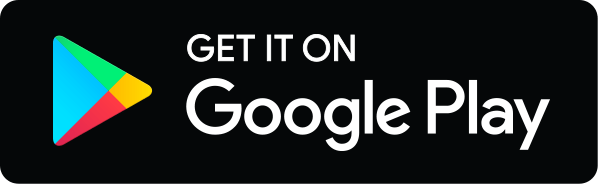
