Feature
Embryo
Fetus
Blastogenesis
Organogenesis
Week
1
2
3
4
5
6
7
8
9
10
Bilaminar disc. Endoderm
X
Yolk sac. Connecting stalk
X
Trilaminar disc
X
Early: midline developmental field. Induction of gastrointestinal patterning
X
Late: primitive foregut, midgut, hindgut; respiratory and hepatic primordia
X
Cylindrical embryo. Definitive tubular gastrointestinal tract. Umbilical cord
X
Beginning intestinal rotation
X
Remodeling, growth, histogenesis. Anus completed
X
X
X
X
Return of bowel to the abdomen. Final rotation and fixation
X
Sexual differentiation of the perineum
X
Table 1.2
Patterns of congenital anomalies arising during various developmental periods
Developmental period | Age (weeks) | Developmental events | Congenital Anomalies |
---|---|---|---|
Embryo Early blastogenesis | 1–2 | Basic patterning of body: dorsoventrality, rostral caudal axis, laterality | Lethal to embryo: empty chorionic sac and global embryonic growth disorganization |
Late blastogenesis | 3–4 | Midline developmental field Right–left sidedness (visceral situs) Induction of endodermal primordia Basic patterning of the gastrointestinal tract | Lethal to embryo: empty chorionic sac and global embryonic growth disorganization |
Severe gastrointestinal anomalies as part of extensive, sometimes monstrous maldevelopment of embryo, not necessarily lethal (see Table 1.3) | |||
Organogenesis | 5–8 | Differentiation of endodermal primordia into specific segments of the gastrointestinal tract and accessory digestive organ | Isolated anomalies of the gastrointestinal tract Disruptions and deformations |
Histogenesis | |||
Fetus | 9–10 | Return and final rotation and fixation of the intestinal loop | Isolated abnormalities of rotation and fixation Disruptions and deformations |
Sexual differentiation of the perineum | |||
11–34 | Histogenesis, remodeling, growth |
1.1.2 Embryology
1.1.2.1 General Description
Table 1.1 presents the sequence of the major developmental events during the first 10 weeks of intrauterine life. These events are depicted in Figs. 1.1, 1.2, 1.3, 1.4, 1.5, 1.6, 1.7, 1.8, 1.9, 1.10, and 1.11. Each figure is a view of the left side of the embryo. The progression of events can best be appreciated by comparing each figure with the preceding and following figures. Each is labeled with the postconceptual age in days and the greatest length in millimeters, and some have measurement bars which are in millimeters. The postconceptual age and size of embryos are from the revisions by O’Rahilly and Muller in their Tables A-1 and A-2, pp. 490 and 491 (O’Rahilly and Muller 2001). Additional references on human embryology used regarding the figures and their descriptions include Lewis (1912c) and publications by O’Rahilly and Muller (Lewis 1912c; Muller and O’Rahilly 1983, 1985, 1986; O’Rahilly 1970, 1971; O’Rahilly and Muecke 1972; O’Rahilly and Boyden 1973; O’Rahilly and Tucker 1973; O’Rahilly 1978, 1983; O’Rahilly and Muller 1981, 1989).
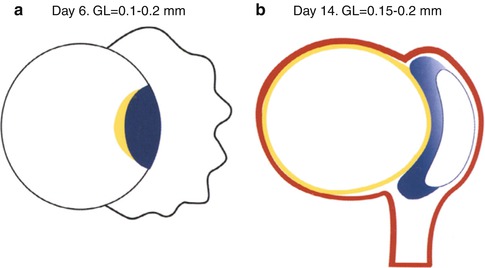
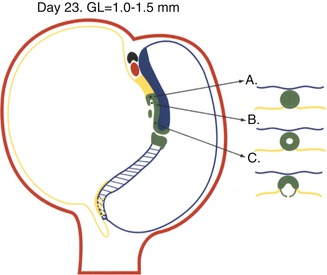
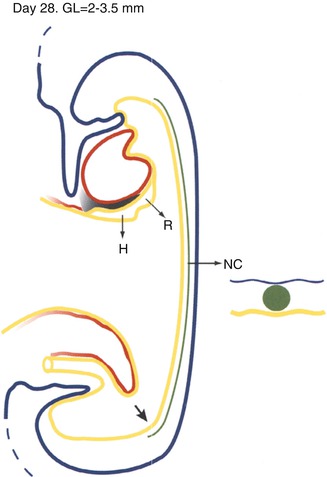
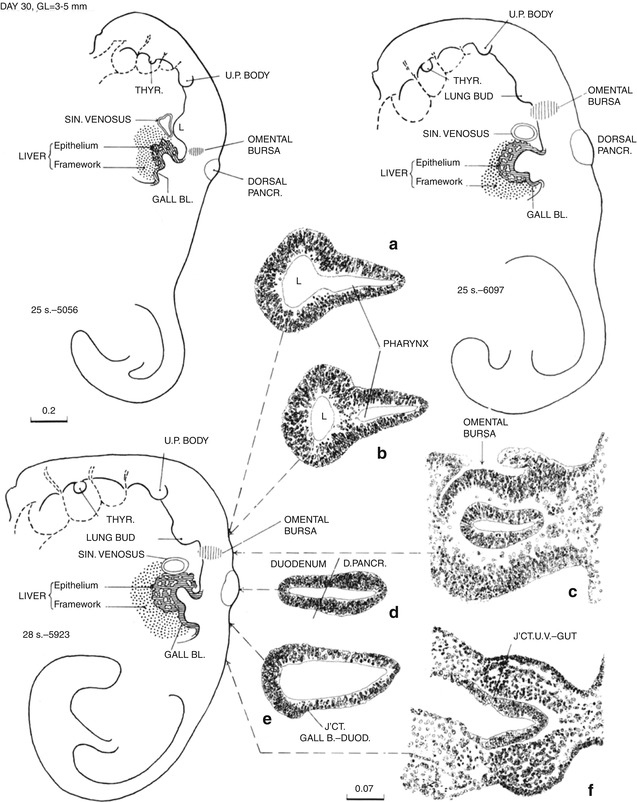
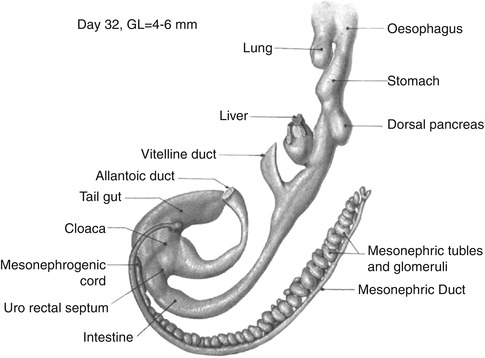
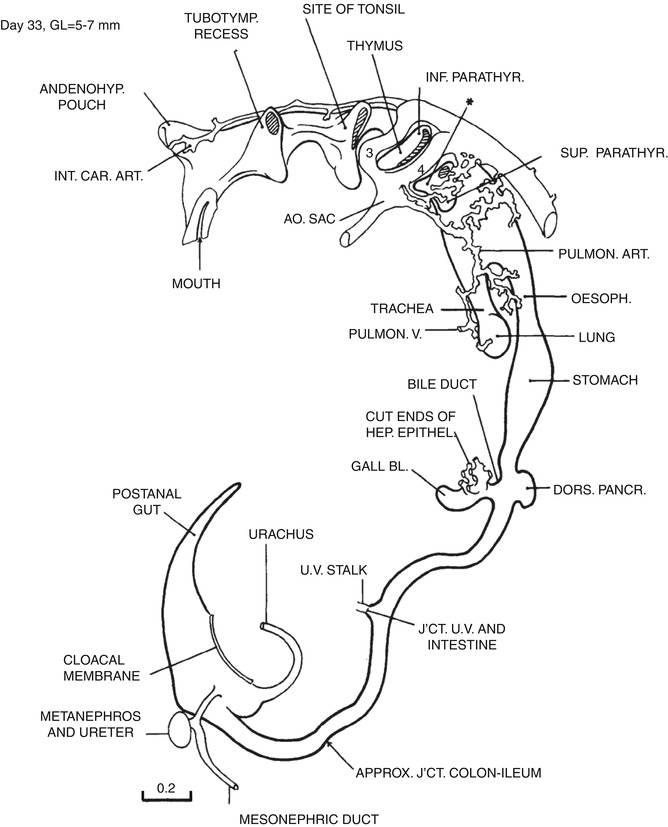
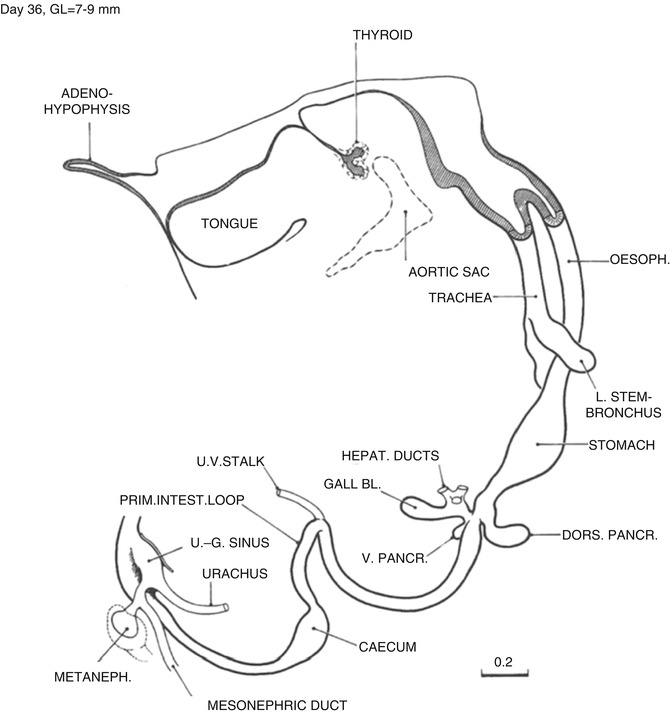
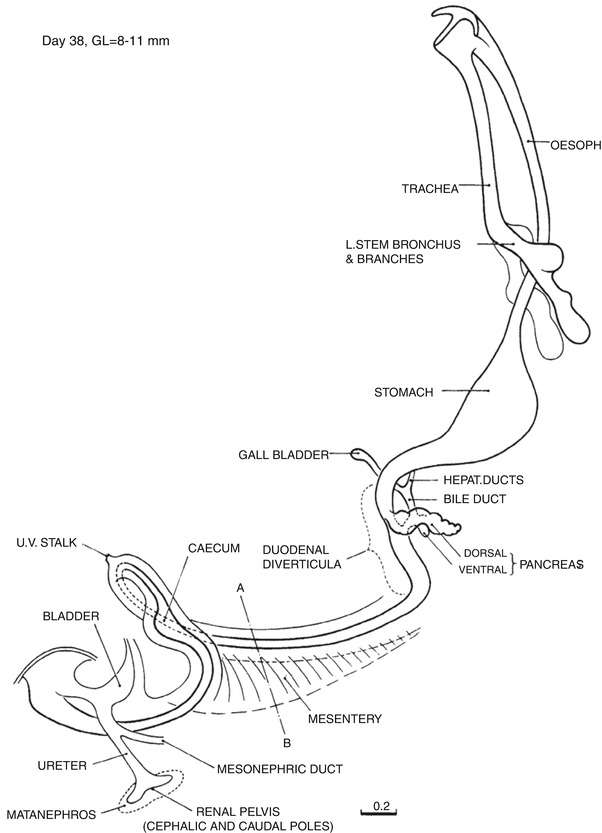
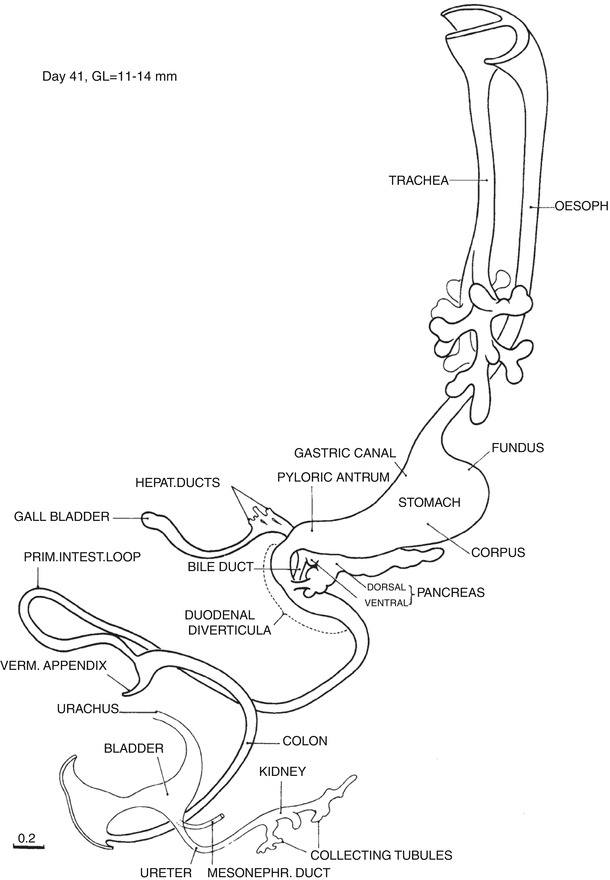
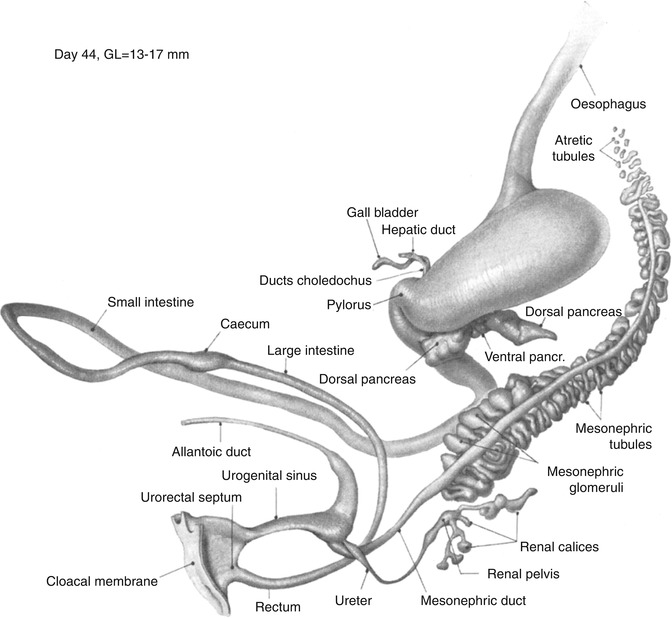
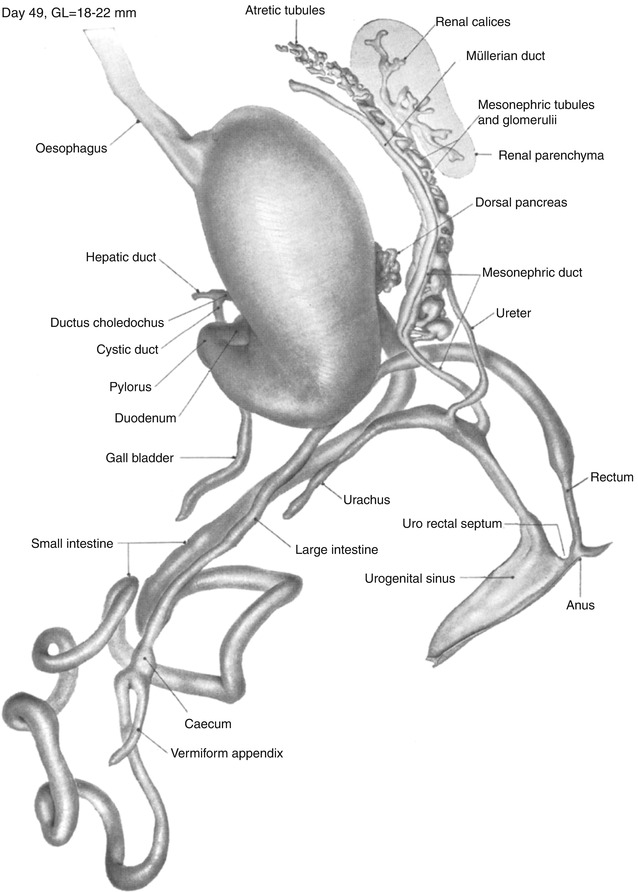
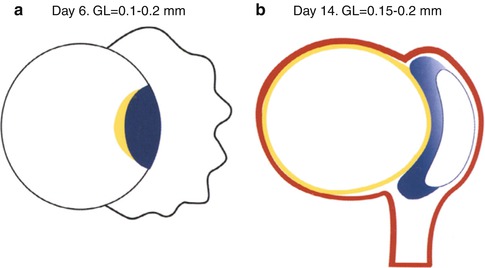
Fig. 1.1
Gastrointestinal development in the first 2 weeks. (a) Day 6. Schematic median section of the implanting blastocyst with bilaminar disc. The endoderm (yellow) is the anlage of the gastrointestinal tract. The endoderm and ectoderm (blue) form the bilaminar embryonic disc. The dorsal–ventral axis is established. The trophoblast (black) surrounds the blastocystic cavity to the left of the embryonic disc and invades the decidua (not shown) to the right of the disc. (b) Day 14. Schematic median section. The endoderm lines the secondary yolk sac. It is the midline endoderm of the roof of the yolk sac that will form the gastrointestinal tract. The ectoderm has formed the amnion which surrounds the amniotic cavity on the dorsal surface of the disc. The embryo is surrounded by the extraembryonic mesoderm (red) which condenses to form the connecting stalk at the caudal end of the embryo. This establishes the cranial–caudal axis of the embryo. The connecting stalk will later form a major part of the umbilical cord
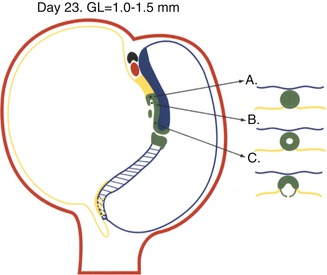
Fig. 1.2
Day 23. Schematic median section of the trilaminar disc showing the structures of the midline developmental field. The endoderm in the midline of the roof of the yolk sac has been replaced by the notochordal apparatus (green) and the primitive streak (blue crosshatch). The notochordal process (A) has been mostly hollowed out by the notochordal canal (B), and much of the floor of the canal has disintegrated. The neurenteric canal forms a direct communication between the amniotic cavity and yolk sac through the primitive pit. The notochordal plate (C) and the primitive streak form the roof of the yolk sac. The prechordal plate, the thick yellow endodermal plate rostral to the green notochordal process, and the allantoic diverticulum which extends into the connecting stalk are the first evidences of endodermal differentiation. The cloacal membrane (blue and yellow crosshatch caudal to the primitive streak) is located on the dorsal surface of the embryonic disc. The cardiogenic primordium (red) and septum transversum (black) have appeared rostral to the prechordal plate. Extraembryonic mesoderm (red shading) surrounds the embryo and forms the connecting stalk. It is during this time that the destiny of specific areas of primitive endoderm to become specific segments of the gastrointestinal tract or its accessory digestive organs is induced by the notochordal apparatus, primitive streak, mesoderm, and cardiogenic mesenchyme. Right–left laterality including visceral situs is determined
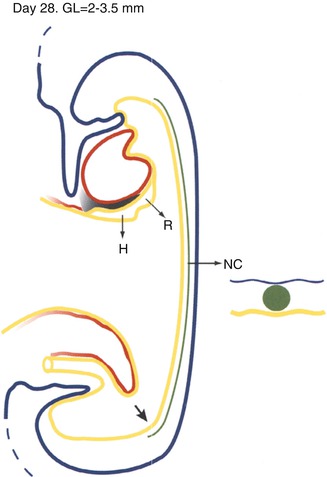
Fig. 1.3
Day 28. Schematic median section of early cylindrical embryo. The head fold has resulted in the formation of the foregut and brought the heart (red) and septum transversum (black shading) into a ventral position abutting the yolk sac. The respiratory (R) and hepatic (H) primordia are endodermal thickenings in the ventral midline at the junction of the foregut and yolk sac. These are the first specific areas of endoderm to differentiate into their predetermined organs. The notochordal plate has separated from the endoderm and thereby has been transformed into the notochord (N). The endoderm has been reconstituted in the roof of the yolk sac. The tail fold has resulted in the formation of the hindgut and cloaca and brought the cloacal membrane, connecting stalk, and allantoic diverticulum from a dorsal to a ventral position. The midgut is still essentially the roof of the yolk sac. The approximate site of the closed neurenteric canal is still visible near the caudal end of the roof of the gut
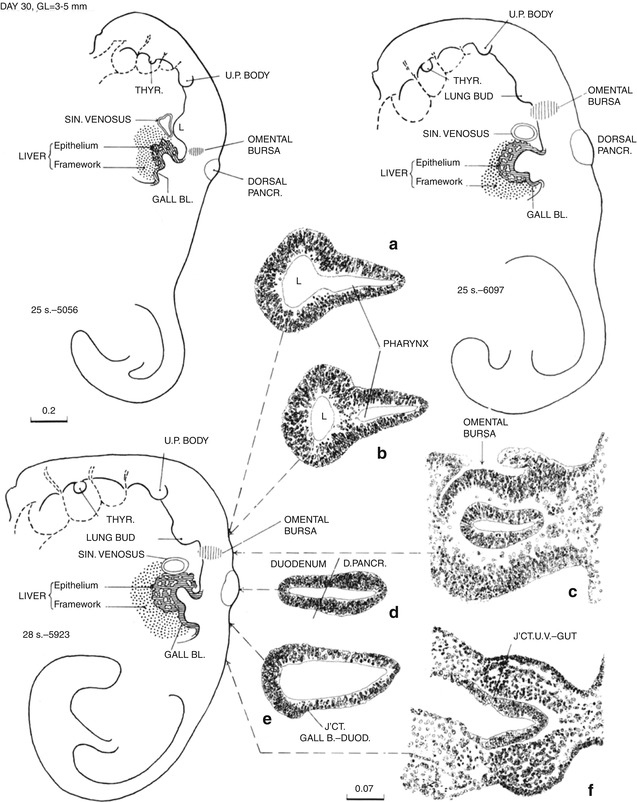
Fig. 1.4
Day 30, three embryos numbers 5056, 6097, and 5923 with slightly increasing degrees of maturation. The elongating gastrointestinal tract including the midgut is now tubular. The lung bud (L in 5056) has arisen from the pulmonary primordium. The hepatic diverticulum has arisen from the hepatic primordium, and the diverticulum of the gallbladder has arisen from its primordium on the caudal wall of the hepatic diverticulum. The dorsal pancreatic diverticulum is now present. Each of these diverticula is lined by thick proliferating epithelium (insets A, B, D, and E). The waist-like constriction of the foregut at lower border of the lung bud is the gastropharyngeal isthmus which will elongate to become the esophagus. The segment between there and the dorsal pancreas is the stomach. The label “GALL BL.” is in the junction of the stalk of the yolk sac with the midgut. The stalk is becoming relatively more narrow (inset F: UV umbilical vesicle or yolk sac). The hindgut and allantoic diverticulum join to form the cloaca, and the tailgut (postanal gut) extends caudally from the cloaca (all unlabeled). The cloacal membrane faces dorsally and lies between the base of the allantoic diverticulum and the origin of the tailgut (unlabeled) (From Streeter (1942) by permission of the Carnegie Institution of Washington)
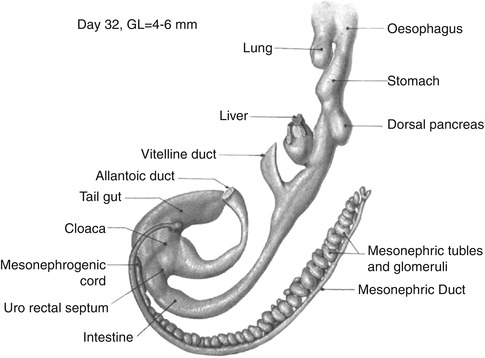
Fig. 1.5
Day 32. All segments are elongating and becoming more well defined. The stomach is fusiform. The liver has been removed from the hepatic ducts. The diverticulum of the gallbladder is the unlabeled bulge on the caudal surface of the hepatic diverticulum. The stalk of the yolk sac is now a narrow duct, the vitelline or omphalomesenteric duct. The connecting stalk containing the allantoic duct and the omphalomesenteric duct have been ensheathed by amnion to form the umbilical cord (not shown). Urorectal separation is beginning. The cloacal membrane lies between the base of the allantoic duct and the origin of the tailgut (unlabeled) (From Shikinami (1926) by permission of the Carnegie Institution of Washington. Illustration by J.F. Didusch from modified Born reconstruction and model by Shikinami)
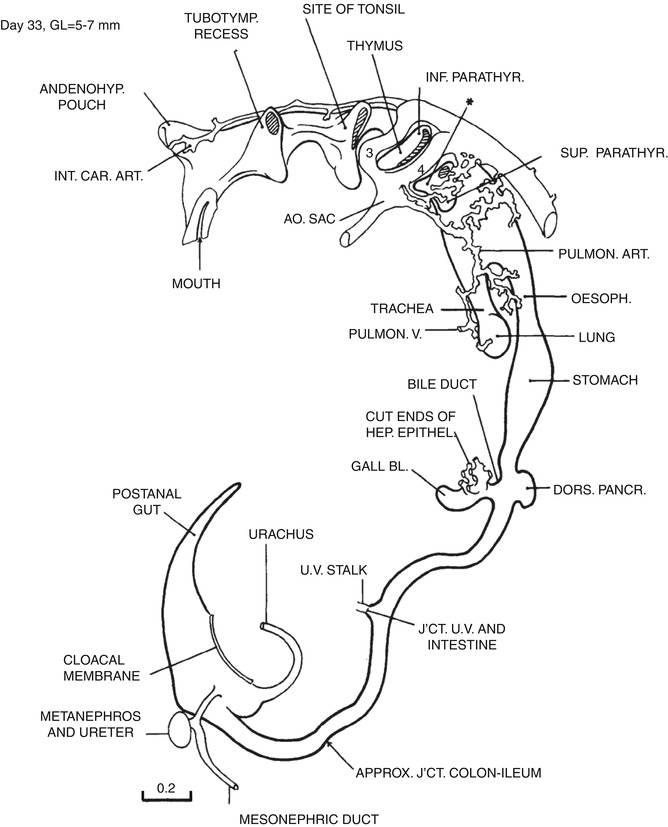
Fig. 1.6
Day 33. The esophagus is completely separated from the trachea. The greater curvature is becoming defined. The primary intestinal loop has formed, and the proximal limb from the duodenal–midgut junction to the omphalomesenteric duct (UV stalk) has already rotated slightly to the right. The distal loop from the omphalomesenteric duct to the mid-hindgut remains stationary. The cloacal membrane still faces dorsally (From Streeter (1945) by permission of the Carnegie Institution of Washington. Drawing based on a three-dimensional model by O.O. Heard)
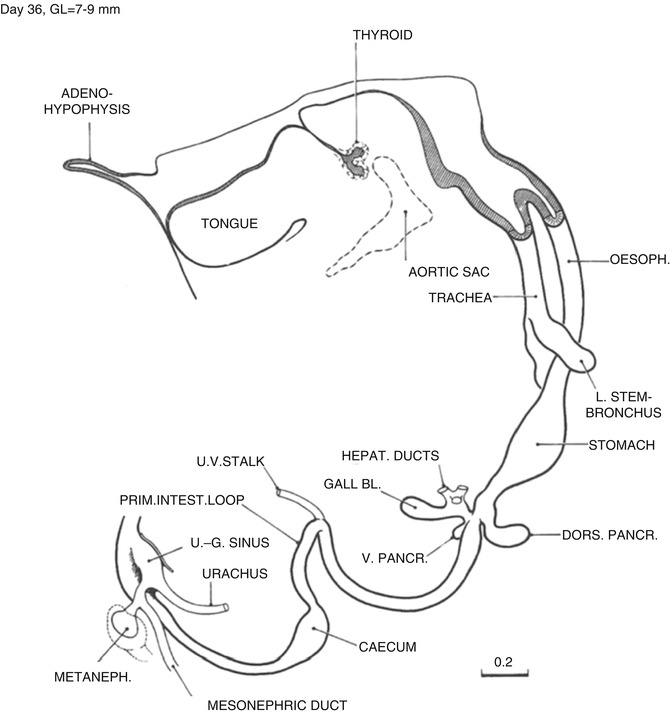
Fig. 1.7
Day 36. The esophagus is beginning to elongate rapidly. The greater curvature begins to face toward the left, and the beginning of the duodenal loop is discernable. The diverticulum of the ventral pancreas has arisen from its primordium on the ventral wall of the hepatic diverticulum which now enters the right side of the duodenum. A waist-like narrowing of the duodenum appears at the level of the entrance of the ducts of the gallbladder and pancreas giving the duodenum an hourglass shape. The anchoring points of the proximal and distal limbs of the intestinal loop are identifiable in the midline at the duodenal–jejunal junction and just distal to the cecum, respectively. The superior mesenteric artery exits from the aorta in the midline just caudal to the anchoring point of the proximal limb (not shown). It extends to the apex of the loop at the site of the omphalomesenteric duct. The origin of the superior mesenteric artery is a fixed point around which the intestinal loop rotates counterclockwise. Continued separation of the rectum from the cloaca has resulted in the formation of the urogenital sinus. The caudal curl is beginning to unfold so that the cloacal membrane now faces rostrally rather than dorsally (From Streeter (1948) by permission of the Carnegie Institute of Washington. Illustration by J.F. Didusch from a reconstruction by O.O. Heard)
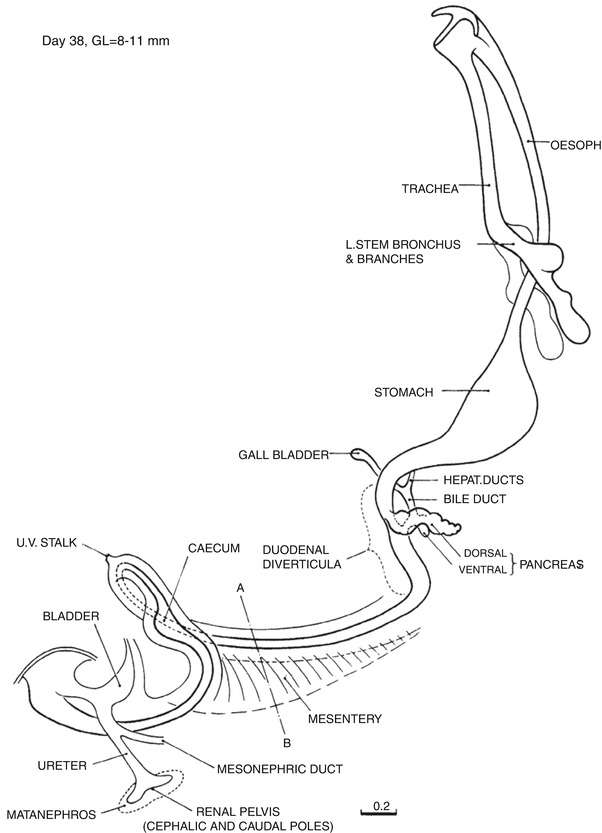
Fig. 1.8
Day 38. The esophagus is elongating rapidly. The duodenal loop is distinct. The cystic and common hepatic ducts are well defined and join to form the common bile duct which enters the duodenum posteriorly. The ventral and dorsal portions of the pancreas are contiguous but unfused. This is the period of rapid proliferation of esophageal and duodenal epithelium and formation of duodenal diverticula. The dorsal mesentery is present and defines the position of the future ligament of Treitz and the caudal anchoring point of the intestinal loop just distal to the cecum. The intestinal loop has herniated into the umbilical cord, and the proximal limb has now completed 90° of counterclockwise rotation to lie to the right of the stationary distal limb. The tailgut has disappeared. The caudal curl continues to uncurl, and the cloacal membrane faces ventrally (From Streeter (1948) by permission of the Carnegie Institution of Washington. Illustration by J.F. Didusch from a reconstruction by O.O. Heard)
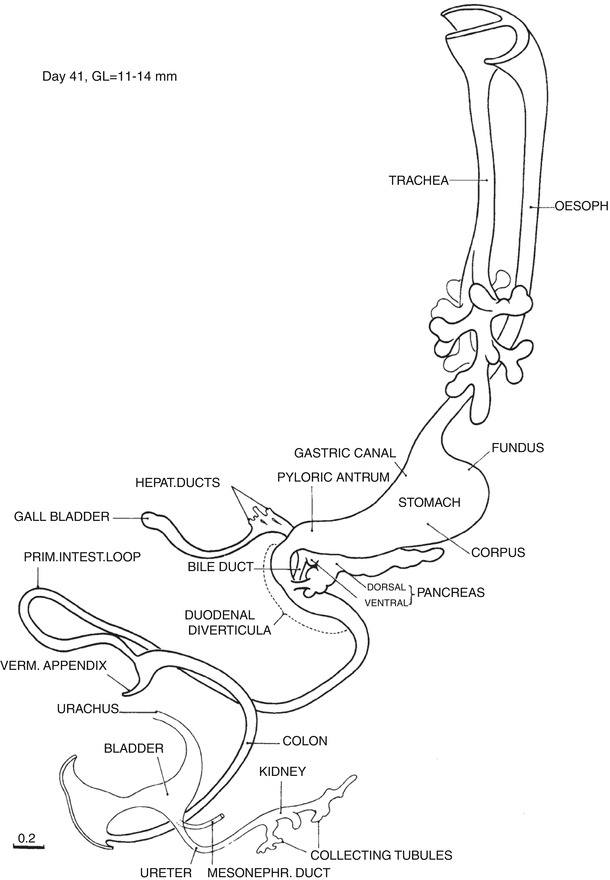
Fig. 1.9
Day 41. The esophagus is long. The anatomy of the stomach is defined, and the greater curvature faces increasingly to the left. The two pancreases are fused, but their ducts are not. The duodenal lumen is occluded, and the hourglass shape of the duodenum is accentuated. The omphalomesenteric duct has disappeared. The appendix is present. The distal limb of the intestinal loop has begun to rotate counterclockwise to the left of the midline. The urorectal separation is complete in some. The continued straightening of the caudal curl results in the cloacal membrane facing ventro-caudally (From Streeter (1948) by permission of the Carnegie Institution of Washington. Illustration by J.F. Didusch from a reconstruction by O.O. Heard)
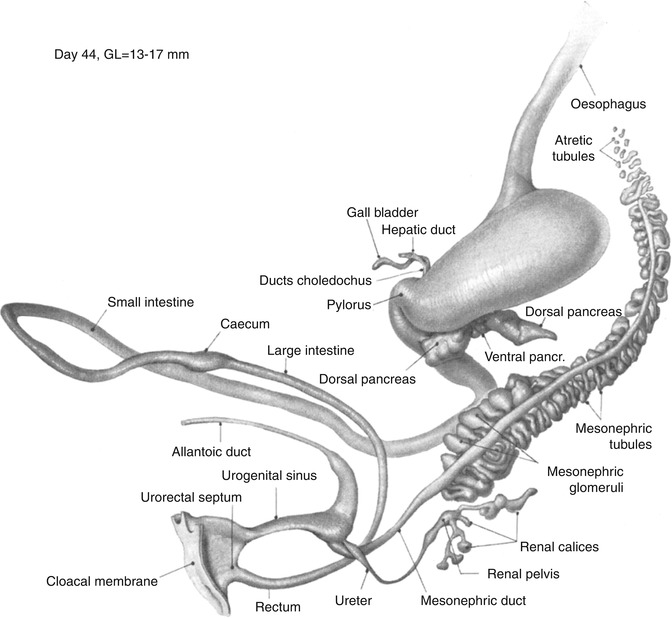
Fig. 1.10
Day 44. The greater curvature faces directly to the left. The third part of the duodenum has grown from right to left along the lower border of the head of the pancreas under the superior mesenteric artery and lies to the left of the midline. The distal loop continues to rotate counterclockwise so that the cecum lies rostral and anterior to the proximal loop. The cloacal membrane faces caudally (From Shikinami (1926) by permission of the Carnegie Institution of Washington. Illustration by J.F. Didusch from a reconstruction by J. Shikinami)
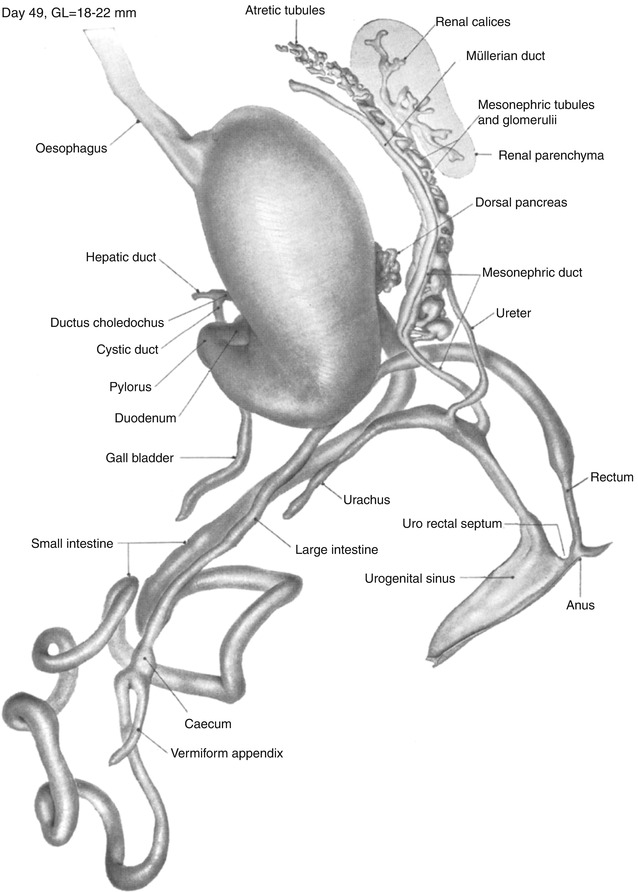
Fig. 1.11
Day 49. The intestinal loop remains in the umbilical cord. The fourth part of the duodenum has turned vertically along the left border of the head of the pancreas to the left of the superior mesenteric artery and then ventrally out from under the peritoneum to form the duodenal–jejunal flexure. The duodenal loop is now complete, and the ligament of Treitz has formed to the left of the flexure. The proximal limb with six primary coils continues to rotate counterclockwise and now hangs caudal and dorsal to the distal loop. Rotation of the midgut loop concludes with the return of the loop into the abdomen. The rectum is completely separate from the urinary tract, and the rectum and anus are beginning to be delineated (From Shikinami (1926) by permission of the Carnegie Institution of Washington. Illustration by J.F. Didusch from a reconstruction by J. Shikinami)
1.1.2.2 Blastogenesis
During the first 2 weeks when the embryo is a bilaminar disc, endodermal development is slow. By the end of the second week, the endoderm forms the secondary yolk sac. The endoderm of the median plane of the roof of the yolk sac will become the gastrointestinal tract (Fig. 1.1a, b). Developmental abnormalities during the first 2 weeks are lethal. During the third and fourth weeks, the structures of the midline developmental field appear. The primitive streak and notochordal apparatus replace the endoderm in the median plane of the roof of the yolk sac, and with the appearance of the mesoderm, the trilaminar disc is formed (Fig. 1.2). During this crucial period, the plan for the future form of the gastrointestinal tract is imprinted on the endoderm by the inductive influences of the notochord, primitive streak, mesoderm, and cardiogenic primordium (see Sect. 1.1.5 below for details). By the end of the fourth week, the lateral folds transform the embryonic disc into a cylinder; the head and tail folds form; the primitive foregut, midgut, and hindgut can be seen; and the respiratory and hepatic primordia appear (Fig. 1.3). Developmental abnormalities that arise during the third and fourth weeks of blastogenesis are often severe, widespread, and sometimes monstrous malformations of the embryo which often involve the gastrointestinal tract (Opitz 1993) (Table 1.3).
Table 1.3
Errors of blastogenesis involving the gastrointestinal tract
Microgastria–limb reduction anomaly |
VACTERL association |
VACTERL-H autosomal recessive syndrome |
Sirenomelia |
Caudal regression |
Anal atresia |
Esophageal atresia and tracheoesophageal fistula |
Pentalogy of Cantrell (ectopia cordis with sternal, pericardial, diaphragmatic, and upper anterior abdominal wall defects) |
Disorganization anomaly |
Early amniotic rupture sequence |
Limb–body wall complex |
OEIS complex (omphalocele, exstrophy, imperforate anus, spinal defects) |
Anomalies of the neurenteric canal |
Cloacal exstrophy |
Currarino triad (imperforate anus, sacral defects, presacral teratoma) |
Urorectal septum sequence |
Anomalies of body stalk, body wall, and umbilical formation |
Heterotaxy (polysplenia/asplenia) anomalies |
Monozygotic twinning including conjoined and acardiac twins |
1.1.2.3 Organogenesis
Organogenesis begins with the fifth week when suddenly the entire tubular gastrointestinal tract, its major divisions, and its accessory organs of digestion emerge from the imprinted primordium of the primitive endoderm (Figs. 1.4, 1.5, and 1.6). During the sixth week, organogenesis continues, rotation and histogenesis begin, and the digestive tract attains much of its final form (Figs. 1.7, 1.8, and 1.9). During the seventh and eighth weeks, rotation and histogenesis continue, and remodeling of the anal canal and anus is completed (Figs. 1.10 and 1.11). During the ninth and tenth weeks, the intestine returns to the abdomen; rotation and fixation are completed, and sexual differentiation of the perineum takes place. During the remaining 30 weeks of intrauterine life, development consists of continued histogenesis, remodeling, growth, and maturation. Malformations arising during late organogenesis and fetal life are less extensive, more often single, and more likely due to disruptions than those arising during the third and fourth weeks.
1.1.3 Rotation and Fixation
Rotation begins with the formation of the primary intestinal loop on day 33, gradually continues through the eighth week, and is quickly completed when the intestine returns to the abdomen between 9 and 10 weeks. The portrayal of intestinal rotation in many current texts as counterclockwise rotation of the proximal and distal anchoring points of the midgut loop around the superior mesenteric artery is probably not completely accurate. Neither the anchoring point nor the cecum rotates around the superior mesenteric artery. Both anchoring points and the cecum remain stationary. Rotation is confined to the midgut loop (Frazer and Robbins 1915; Frazer 1940; Hansen 1973; Kluth et al. 1995, 2003; Metzger et al. 2011a, b). The growth of the duodenal loop around the expanding head of the pancreas brings the third part of the duodenum under the superior mesenteric artery before the midgut loop returns to the abdomen. As a result the superior mesenteric artery hangs over the ventral wall of the third portion of the duodenum. Follow the early stages of rotation in Figs. 1.6 (day 33), 1.7, 1.8, 1.9, 1.10, and 1.11 (day 49). The position seen in Fig. 1.11 is similar to that prior to the sudden return of the intestine into the abdomen at 63 days (Mall 1898, 1899). On day 63 the proximal limb returns first. As it returns, it swings under the distal limb into the left side of the abdomen. As the distal limb then rapidly returns, the midgut–hindgut junction lies in its definitive position to the left of the midline in the epigastrium ventral to the duodenal–jejunal flexure and ligament of Treitz, the distal loop is draped from that point diagonally in front of the small intestinal loops to the right lower quadrant, and the cecum lies in the right lower quadrant at the level of the iliac crest and the lower pole of the right kidney. The lower border of the right lobe of the liver extends to the iliac crest and covers the cecum. Because of the rapid increase in the length of the lumbar spine, the position of the liver relative to the cecum subsequently moves rostrally uncovering the cecum. This has been misinterpreted as “cecal descent.” The cecum does not descend, the liver ascends. Rotation is completed by the tenth week, and fixation continues throughout fetal life. See Estrada (1958) for an extensive review and Kim et al. (2003) for a recent review of the timetable of rotation in staged human embryos.
1.1.4 Histogenesis
1.1.4.1 Introduction
This description is compiled from Lewis (1912b), Salenius (1952), Grand et al. (1976), Valdes-Dapena (1979), Semba et al. (1983), O’Rahilly and Muller (1987), and Skandalakis et al. (1994; Skanalakis et al. 1994a, b, c). For uniformity the timing of events is given in gestational ages. When primary sources used greatest lengths, these have been converted to postconceptual ages prior to 20 weeks’ gestation using data from O’Rahilly and Muller (2001) and Kalousek, Fitch, and Paradice (1990) or menstrual ages after 20 weeks using data from Singer, Sung, and Wigglesworth (1991). Mucosal histogenesis begins with a transient phase of epithelial proliferation. The proliferating epithelium completely occludes the lumen of the duodenum, significantly narrows the lumen of the esophagus, and may mildly narrow the lumens of the cardia, pylorus, upper jejunum, and distal ileum. These proliferations may be accompanied by the transient formation of multiple antimesenteric diverticula in the duodenum, upper jejunum, and distal ileum. Some instances of congenital atresia, stenosis, or diverticula may be the result of abnormalities in the formation or resolution of the proliferative phase.
1.1.4.2 Esophageal Mucosa
The proliferative phase begins in the 6th and ends in the nineth week. The epithelial membrane becomes stratified. Strands of rapidly proliferating epithelium traverse the lumen without completely occluding it. Ciliated columnar epithelium covers the epithelial surface of the mid-esophagus at 10 weeks and spreads to both ends by the 11th week. Stratified squamous epithelium begins to replace the ciliated columnar epithelium in the mid-esophagus at 16 weeks and spreads proximally and distally to cover the entire esophagus by birth except for the proximal esophagus where islands of ciliated columnar epithelium may persist. These disappear shortly after birth. The superficial cardiac glands of the lamina propria appear in the 13th week. The submucosal mucous glands appear in the 27th week. A recent ultrastructural study suggests slightly different timing and details of esophageal histogenesis (Menard 1995).
1.1.4.3 Gastric Mucosa
The mild proliferative phase is most pronounced in the cardia and pylorus where minor compromise of the lumen is reported by some authors. Gastric mucosa with foveolar epithelium and gastric pits appears at 7–8 weeks. Gastric glands with mucous neck cells, parietal cells, chief cells, and endocrine cells appear at 9–10 weeks. Intestinal villi appear in the cardia and pylorus where they are normally abundant by 30 weeks. They disappear by birth. Intestinal metaplasia of cardiac or pyloric epithelium may be a dedifferentiation to the normal fetal condition. The cardiac mucosa is thought to arise from undifferentiated gastric mucosa and not from esophageal metaplasia (Zhou et al. 2001). The cardia may be extremely short, and parietal cells may be seen within 1 or 2 mm of the esophageal mucosa. The ultrastructure of gastric mucosa in staged human embryos has been described (Otani et al. 1993). See Table 1.4.
Table 1.4
Histogenesis of gastric mucosa
Feature | Week |
---|---|
Proliferation (mild) | 7 |
Gastric pits | |
Lesser curvature | 7 |
Body and fundus | 8 |
Cardia and pylorus | 10 |
Fundal glands | 9 |
Parietal cells | 9 |
Enterochromaffin cells | 9 |
Goblet cells | 10 |
Chief cells | 10 |
Surface epithelium differentiates | |
Cardia and pylorus | 11 |
Lesser curvature | 12 |
Fundus | 13 |
Cardiac and pyloric glands | 13 |
Esophagogastric junction | |
Intestinal villi (goblet cells, enterocytes, and brush border) in the cardia and pylorus | 30a |
1.1.4.4 Small Intestinal Mucosa
In the duodenum exuberant epithelial proliferation begins early in the sixth week and completely occludes the lumen by the end of the sixth week. A recent study suggests that the occlusion is not due to proliferation but to morphogenetic events involved in elongation of the duodenum (Matsumoto et al. 2002). The expanding mass of proliferating epithelium distends the duodenum proximal and distal to the hepatobiliary and pancreatic diverticula imparting an hourglass shape to the duodenum with the narrow waist at the level of the hepatobiliary and pancreatic ducts. Congenital duodenal atresia or stenosis often occurs at the site of this waist and may be associated with hepatobiliary and pancreatic duct abnormalities. Epithelial diverticula form along the antimesenteric border. By the eighth week the proliferation ends, the lumen is reestablished, and the diverticula disappear. Some authors (Matsumoto et al. 2002; Lewis 1912b; Lewis and Thyng 1908) report similar but less exuberant, nonocclusive proliferations accompanied by similar antimesenteric diverticula in the proximal jejunum and distal ileum. These begin in the seventh week and disappear by the 16th week. Villi appear first in the duodenum in the eighth week, and crypts of Lieberkühn appear in the nineth week. Villi are shortest in the proximal duodenum and become progressively longer distally. Brunner’s glands appear in the proximal duodenum by 12–14 weeks and spread caudally to the distal duodenum. They are largest and most numerous in the proximal duodenum and become progressively smaller and less numerous distally. They are sparse distal to the papilla of Vater. Villi and crypts spread from rostral to caudal. They arrive in the mid-small intestine by the nineth week and reach the distal ileum by the 12th week (Siedman and Walker 1987; Dimmick and Hardwick 1992). The stem cells are located in the bases of the crypts. They proliferate and differentiate into enterocytes, goblet cells, and enteroendocrine cells. Enterocytes and goblet cells migrate centripetally up the crypts to the tips of the villi where they may undergo apoptosis and slough into the lumen. Some enteroendocrine cells migrate up to the mid-region of the crypt, while others remain in the depths of the crypts. Paneth cells remain in the depths of the crypts. Cytologic differentiation of the crypts begins with the appearance of goblet cells in the eighth week followed by enterocytes with prominent brush borders, Paneth cells and enteroendocrine cells in the nineth week, and Segi’s cap (Kobayashi et al. 1980) in the 20th week. Paneth cells contain large numbers of coarse, brightly eosinophilic globules in the supranuclear region. Some enteroendocrine cells can be identified in hematoxylin- and eosin-stained sections by the presence of very fine eosinophilic granules in the subnuclear region; however, most enteroendocrine cells can be identified only by immunohistochemical stains which label nonspecific neurosecretory granules or specific neurosecretory products. The time of appearance and electron microscopic features of 13 enteroendocrine cells have been described in human embryos between 9 and 22 weeks’ gestation (Moxey and Trier 1977). The author has identified coarse, brightly eosinophilic, hyaline globules in the cytoplasm of enterocytes of fetuses between 16 and 20 weeks. See Huff in Ernst et al. (2011). They have been described but not illustrated by Semba and Tanaka (1983) and are similar to the thanatosomes described by Dikov et al. (2007). The histologic appearance of the small intestine resembles that of a newborn by 20 weeks (Lebenthal and Lebenthal 1999).
1.1.4.5 Colonic Mucosa
In the colon the proliferative phase is minor. At approximately the tenth week, villi cover the surface of the large intestine and persist until the 28th to 30th week. Intestinal villi are normally seen in the embryo not only in the small intestine but also in the cardia, pylorus, and colon. The stems cells are located in the base of the colonic crypts. By the 14th week, they proliferate and differentiate into colonocytes, goblet cells, Paneth cells, and enteroendocrine cells. The colonocytes and goblet cells migrate centripetally up the sides of the crypts and villi to the tips of the villi. The colonocytes are tall columnar cells with basal nuclei, mucin-filled cytoplasm, and faint brush borders. The enteroendocrine cells migrate up to the mid-region of the crypts. The Paneth cells which are rarely found in the colon and then only in the right colon remain in the depth of the crypts. The intervillous surface epithelium differentiates into a single layer containing goblet cells by the 13th to 16th week. Differentiating between microscopic sections of the colon and small intestine may be very difficult especially prior to 30 weeks’ gestation and in poorly preserved specimens. All of the cells covering the colonic villi are mucus containing, either colonocytes or goblet cells, while the cells covering small intestinal villi are predominately nonmucus-containing enterocytes with a bright brush border interspersed with only a few scattered goblet cells.
1.1.4.6 Appendiceal Mucosa
The histogenesis of the appendiceal mucosa is similar to that of the colon except between 20 and 25 weeks’ gestation when the lengths of the crypts become very irregular resulting in a very uneven undulating muscularis mucosae in contrast to the nearly perfect equality in the lengths of the test tube–like colonic crypts and the straightness of the colonic muscularis mucosae. Some of the appendiceal crypts push into the submucosa somewhat like diverticula, although they are surrounded by muscularis mucosae. Some become microcystically dilated and filled with apoptotic and inflammatory debris particularly intact and degranulated eosinophils. The dilated crypts are surrounded by cuffs of spindle shaped and polygonal mesenchymal cells and inflammatory cells especially eosinophils. At the same time, submucosal aggregated nodules of lymphoid tissue become prominent. No evidence of appendicitis, swallowed infected amniotic material, or other abnormalities accompanies these changes. Evidence of regression of the microcystic cryptitis includes whorled foci of fibrosis surrounding degenerated epithelial cells with or without calcification or multinucleated giant cells. See Huff in Ernst et al. (2011). Similar findings have rarely been reported previously (Lewis 1912a). Because these changes occur at the same time that aggregated lymphoid tissue develops in the mucosa-associated lymphoid tissue, spleen, and other lymphoid tissues, the possibility that they are somehow associated with the normal maturation of the lymphoid system is raised.
1.1.4.7 Anal Mucosa
The anal canal including the internal sphincter and anal transitional epithelium is well developed by the end of the eighth week. The anal transitional epithelium is composed of irregular stratified cuboidal and columnar epithelium. Peg cells and columnar ciliated cells are sparsely scattered along the surface. A few goblet cells are seen within the epithelium and on the surface reminiscent of conjunctival epithelium. The length of the hypoganglionic segment in fetuses is often 1 cm or less.
1.1.4.8 Mucosa-Associated Lymphoid Tissue
As early as the seventh week, scattered lymphoid cells can be found in the lamina propria. By the 11th week, clusters of precursor lymphoid cells can be identified immunohistochemically in sites where lymphoid follicles will develop in the future. Recognizable diffuse and aggregated lymphoid tissue in the lamina propria is very hard to find in routine sections at 14 weeks, only slightly more easily seen at 16 weeks, and occasionally found at 19 weeks. At 20 weeks lymphoid follicles become larger, more well organized, and more frequent and may extend into the submucosa especially in the terminal ileum, appendix, duodenum, and anorectum. By 24 weeks, Peyer’s patches, sheets of aggregated primary follicles in the lamina propria and submucosa, appear especially in the terminal ileum (Spencer et al. 1986). The development of diffuse and aggregated mucosa-associated lymphoid tissue, Peyer’s patches, and primary follicles is independent of stimulation by foreign antigens. Germinal centers and plasma cells develop in response to a foreign antigenic stimulus. The fetal gastrointestinal tract is normally not exposed to such a stimulus and therefore lacks germinal centers and mature plasma cells. The neonate responds to the antigenic stimulus of colonization at birth with the formation of germinal centers and plasma cells 2–4 weeks after birth. The fetal mucosal lymphoid system has the capacity to respond to an abnormal intrauterine antigenic stimulus with the formation of germinal centers and plasma cells prior to 20 weeks possibly as early as 13–14 . The presence of prominent aggregated mucosa-associated lymphoid tissue prior to 20 weeks or the presence of germinal centers or plasma cells at any time during fetal life indicates fetal infection or other abnormal antigenic stimulus. See Table 1.5.
Table 1.5
Histogenesis of mucosal lymphoid tissue
Week | Feature |
---|---|
Intrauterine | |
7 | Intraepithelial lymphocytes |
10 | T cells with surface recognition |
12 | PHA-responsive lymphocytes |
14 | Lymphocytes with PHA cytotoxicity and ability to mediate graft-versus-host response |
17–20 | Mast cells in the small intestine |
21–24 | Mast cells in the large intestine. Solitary lymphoid follicle in the distal ileum, appendix, and colon |
24 | Peyer’s patches in distal ileum |
40 | Solitary lymphoid follicles in the duodenum, rectum, and possibly stomach |
Postnatal | |
2–4 | Germinal centers and plasma cells |
1.1.4.9 Muscular Coats
The inner circular muscle appears first followed by the outer longitudinal layer and finally by the muscularis mucosae. The waves of differentiation begin in the esophagus and propagate caudally and then at the anorectal junction and propagate cranially. The two meet at the ileocecal junction. See Table 1.6.
Table 1.6
Histogenesis of the muscular coats and myenteric plexus
Layer | Esophagus | Stomach | Small bowel | Colon | |
---|---|---|---|---|---|
Distal | Proximal | Distal | |||
Circular | 6 | 7 | 7 | 9 | 8 |
Longitudinal | 8 | 11 | 10 | 26 | 11 |
Muscularis mucosae | 12 | 14 | 21 | 26 | 21 |
Myenteric plexus | 6 | 7 | 7 | 8 | 12 |
Bidirectional peristalsis | 12 | 12 | |||
Unidirectional peristalsis | 30 | 30 |
1.1.5 Developmental Control Mechanisms
The midline or median plane of the embryo during the third and fourth weeks is the midline developmental field discussed by Opitz and Gilbert (1982), O’Rahilly and Muller (1989), and Martinez-Frias et al. (1998). The prechordal plate, primitive node and streak, notochordal apparatus, mesoderm, septum transversum, and cardiogenic primordium are anatomic constituents of the midline developmental field (Table 1.7 and Figs. 1.2 and 1.3). The primitive streak extends caudally, and the notochordal process extends cranially from the primitive node in the midline of the roof of the yolk sac (Fig. 1.2 and insert a). The primitive streak and notochordal process become intercalated in and replace the endoderm in the midline of the roof of the yolk sac. The median plane of the roof of the yolk sac is no longer formed by endoderm. The primitive pit extends cranially into the notochordal process forming the notochordal canal (Fig. 1.2 and insert b). The floor of the canal rapidly disappears, first directly under the primitive pit forming the neurenteric canal and then cranially until the entire floor of the canal is gone and only its roof, the notochordal plate, remains (Fig. 1.2 and insert c). The notochordal plate, not the endoderm, forms the roof of the yolk sac in the median plane, and the neurenteric canal forms a direct communication between the amniotic and yolk sacs through the developing neural tube. The notochordal plate rolls longitudinally (Fig. 1.2c) into a rod to become the notochord (Fig. 1.3 and insert NC). The endoderm separates from and reforms under the notochord and once again forms the roof of the yolk sac (Fig. 1.3). The relative position of the neurenteric canal shifts caudally. On day 25 it is in the cranial area; on day 28, at the 10th somite (future 6th thoracic vertebra). On day 29 its site is still visible at the extreme caudal end of the embryo distal to the last (the 14th) somite (Muller and O’Rahilly 1985) in what is probably the future lumbar area (Fig. 1.3, arrow). During this period interchanges between the endoderm and mesoderm occur. The notochord contributes cells to the prechordal plate and to the endoderm destined to form the hepatic plate. The prechordal plate contributes cells to the cardiogenic primordium and cephalic mesenchyme. Cells are exchanged between the mesoderm and endoderm. The primitive streak contributes cells to the endoderm. The midline developmental field is involved in the axial and laterality patterning of the embryo including visceral situs. The molecular genetic mechanisms which control this patterning have recently been reviewed (Opitz et al. 2002; Gilbert-Barness et al. 2001; Bohring et al. 1999; Martinez-Frias et al. 1998).
Table 1.7
Development of the primitive streak, notochord, and neurenteric canal in the third and fourth weeks
Feature | Week | 3rd | 4th | ||||||||||||||||
---|---|---|---|---|---|---|---|---|---|---|---|---|---|---|---|---|---|---|---|
Day | 15 | 16 | 17 | 18 | 19 | 20 | 21 | 22 | 23 | 24 | 25 | 26 | 27 | 28 | |||||
Primitive streak | ![]() | ![]() | ![]() | ![]() | ![]() | ![]() | ![]() | ![]() | ![]() | ![]() | ![]() | ![]() | ![]() | ![]() | ![]() | ![]() | ![]() | ||
Endoderm disappearing, median plane | ![]() | ![]() | ![]() | ![]() | ![]() | ![]() | ![]() | ![]() | ![]() | ![]() | ![]() | ![]() | ![]() | ![]() | |||||
Notochordal process | ![]() | ![]() | ![]() | ![]() | ![]() | ||||||||||||||
Primitive node | ![]() | ![]() | ![]() | ![]() | ![]() | ![]() | ![]() | ![]() | ![]() | ![]() | ![]() | ![]() | ![]() | ||||||
Primitive pit | ![]() | ![]() | ![]() | ![]() | ![]() | ![]() | ![]() | ![]() | |||||||||||
Notochordal canal | ![]() | ![]() | ![]() | ||||||||||||||||
Neurenteric canal | ![]() | ![]() | ![]() | ![]() | ![]() | ![]() | ![]() | ![]() | |||||||||||
Notochordal plate | ![]() | ![]() | ![]() | ![]() | ![]() | ||||||||||||||
Notochordal plate, beginning to roll | ![]() | ![]() | |||||||||||||||||
Notochord | ![]() | ![]() | ![]() | ||||||||||||||||
Endoderm restored, median plane | ![]() | ![]() | ![]() |
The structures of the midline developmental field also control the induction of the pattern of the gastrointestinal tract. Differentiation of the endodermal tube precedes that of its investing mesodermal layers. The endodermal tube establishes the gross form of the embryonic gastrointestinal tract. Localized proliferations of endodermal cells result in focal plates or placodes of thickened endoderm at specific sites along the endodermal tube. These thickened endodermal plates are the primordia from which the specific segments of the tract and its endodermal derivatives including the lung, liver, gallbladder, and pancreas will subsequently differentiate (Figs. 1.3, 1.4, 1.5, 1.6, and 1.7 and Table 1.8). It is thought that the primitive node and streak, notochordal apparatus, cardiogenic primordium, septum transversum, and mesoderm induce the formation and determine the destiny of these endodermal primordia to form specific segments and organs of the gastrointestinal tract (O’Rahilly and Muller 1987). The endodermal–mesodermal cellular interchanges described above may be the histologic basis for the molecular endodermal–mesodermal signaling interactions responsible for the initial patterning of the gastrointestinal tract. Similar endodermal–mesodermal interactions within the wall of the digestive tract induce the continued histogenesis of the epithelial, muscular, and stomal components described below. The molecular mechanisms governing these developmental events are emerging primarily from experimental studies (Montgomery et al. 1999; Lebenthal and Lebenthal 1999; Kapur 2002; Beck 2002). The developmental biology of the Adriamycin rat model of the VACTERL association (Diez-Pardo et al. 1996) supports the concepts discussed above and may advance our understanding of the pathogenesis in humans of tracheoesophageal malformations (Kluth and Fiegel 2003), bronchopulmonary foregut malformations (Qi and Beasley 1999), gastrointestinal atresia/stenosis (Gillick et al. 2002) from the esophagus to anus, duplications of the alimentary tract (Qi et al. 2001), malformations of the neurenteric canal, cloacal abnormalities, and anorectal anomalies (Liu and Hutson 2000).
1.2 Congenital Anomalies of the Gastrointestinal Tract
1.2.1 General Considerations
Congenital anomalies of the gastrointestinal tract arise during all developmental periods (Table 1.2). The earlier in embryogenesis a developmental error occurs, the more extensive and severe the resulting anomalies are. Some of the errors of blastogenesis that include anomalies of the gastrointestinal tract are listed in Table 1.3. The causes of anomalies of the gastrointestinal tract include chromosomal abnormalities (numerical and structural), single gene defects, maternal diseases especially diabetes, and maternal exposure to drugs especially hydantoin (pyloric stenosis, duodenal and anal atresia). Causes of disruptions include inherited (Johnson and Meyers 2001) and noninherited maternal and fetal thrombophilic diseases, intrauterine hypoxic/ischemic events, intrauterine infection including varicella (Bruder et al. 2000), iatrogenic vascular disruptions (Stoler et al. 1999), and maternal exposure to vasoactive drugs (Werler et al. 2002). Other diseases of the embryo such as cystic fibrosis and epidermolysis bullosa underlie some gastrointestinal anomalies. Deformations are limited to abnormal shapes of the liver and abnormal rotation and fixation associated with defects of the diaphragm, body wall, and umbilicus. The cause of most anomalies is unknown. Anomalies that arise after completion of organogenesis are often disruptions or deformations; otherwise the causes are not specific to any developmental period.
1.2.2 Atresia and Stenosis
1.2.2.1 General Considerations
The types of atresia/stenosis are schematically depicted in Fig. 1.12. They may occur at any site along the tract, but some sites are more commonly involved than others (Table 1.9). Several disorders associated with multiple atresias/stenoses of the gastrointestinal tract are listed in Table 1.10.
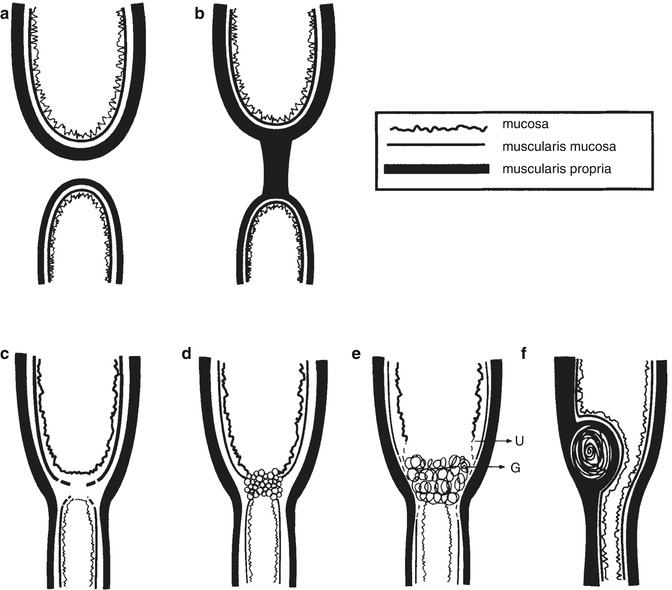
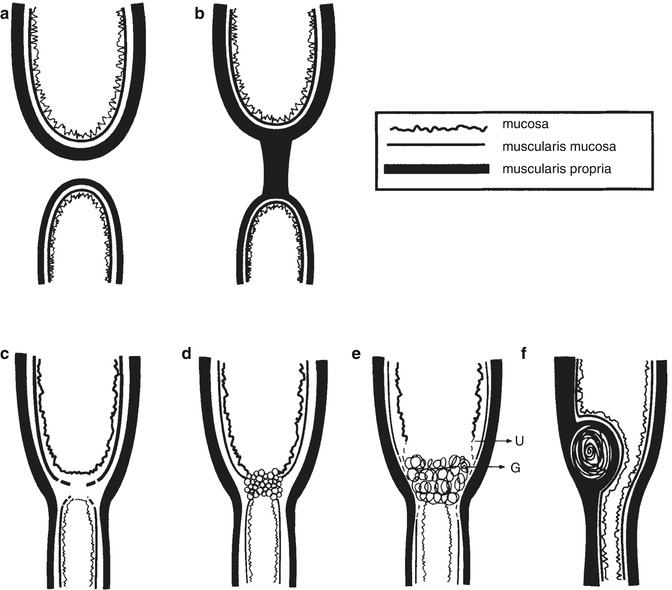
Fig. 1.12
Types of gastrointestinal atresia and stenosis. (a) The blind ends are not connected. This type is associated with absence of the mesentery when it involves the small intestine. (b) The blind ends are connected by a solid cord which may be fibrous, fused muscularis propria, or a tube of muscularis propria with a central core of inflamed granulation tissue containing hemosiderin and calcification indicative of a vascular or other disruption. (c) Membranous atresia, also called a diaphragm or a web. It is composed of two fused layers of submucosa covered on each side by mucosa and remnants of muscularis mucosa. (d) A variant of membranous atresia. The membrane is composed of epithelium which forms multiple glandular or alveolar spaces reminiscent of the proliferative phase of endodermal development. This picture may be seen in familial intestinal atresia and suggests that the embryogenesis involves an abnormality of proliferation or recanalization (Kao et al. 1983). (e) Ulceration (U) is healed by granulation tissue (G) which leads to adhesions between the two apposing mucosal surfaces. This type may be seen in epidermolysis bullosa (Chang et al. 1983). (f) Congenital tumors such as large heterotopias, duplications, or leiomyomas can cause stenosis or atresia
Table 1.9
Occurrence of atresia and stenosis
Number per live births | % of all intestinal atresia | |
---|---|---|
Esophagus | 1:1,500 | |
Stomach | Rare | |
Duodenum | 1:1,500 | 50 % |
Jejunum | 1:2,000 | 20 % |
Ileum | 1:2,000 | 25 % |
Colon | Rare | 5 % |
Rectum and anus | 1:5,000 | |
Multiple | 15 % |
Table 1.10
Disorders with multiple gastrointestinal atresias/stenoses
Familial intestinal polyatresia syndrome | AR (Lambrecht and Kluth 1998) |
Multiple gastrointestinal abnormalities | AR (Gentile and Fiorente 1999) |
Epidermolysis bullosa, lethal and nonlethal | AR (Cetinkursun et al. 1995) |
Carmi syndrome (aplasia cutis) | AR (Carmi et al. 1982) |
Congenital immunodeficiency syndrome | |
Severe combined immunodeficiency syndrome | AR, X (Moreno et al. 1990) |
Other types not specified | |
Cystic fibrosis | |
Gastroschisis | Maternal vasoactive drug use (Werler et al. 2002) |
1.2.2.2 Esophageal Atresia and Tracheoesophageal Fistula
Pathology. Gross (1953) and Swenson et al. (1962) proposed the classical classifications of esophageal atresia and tracheoesophageal fistula. Kluth (1976) presented a comprehensive scheme of nearly 100 major types and subtypes. A subsequent editorial plea to use descriptions instead of complex classifications appeared (Anonymous 1978). Figure 1.13 schematically depicts five common variations found in many current publications. Figures 1.14 and 1.15 represent the two most common types.
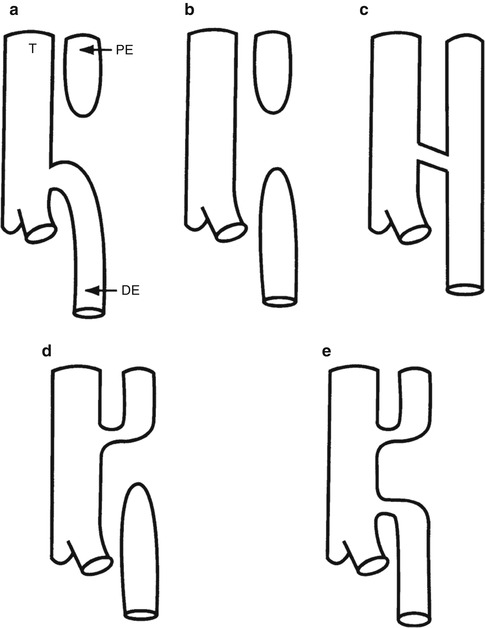
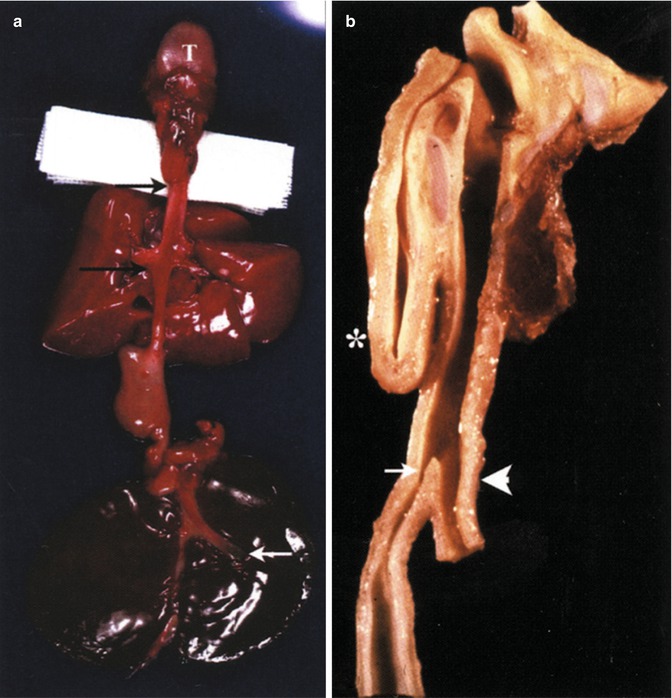
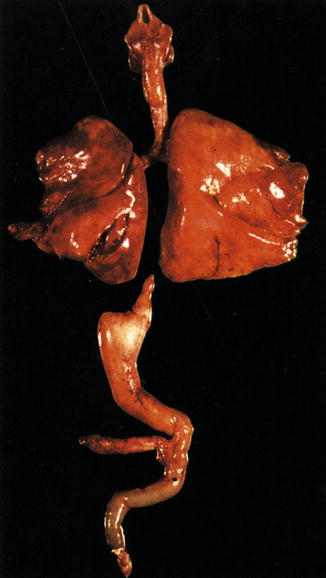
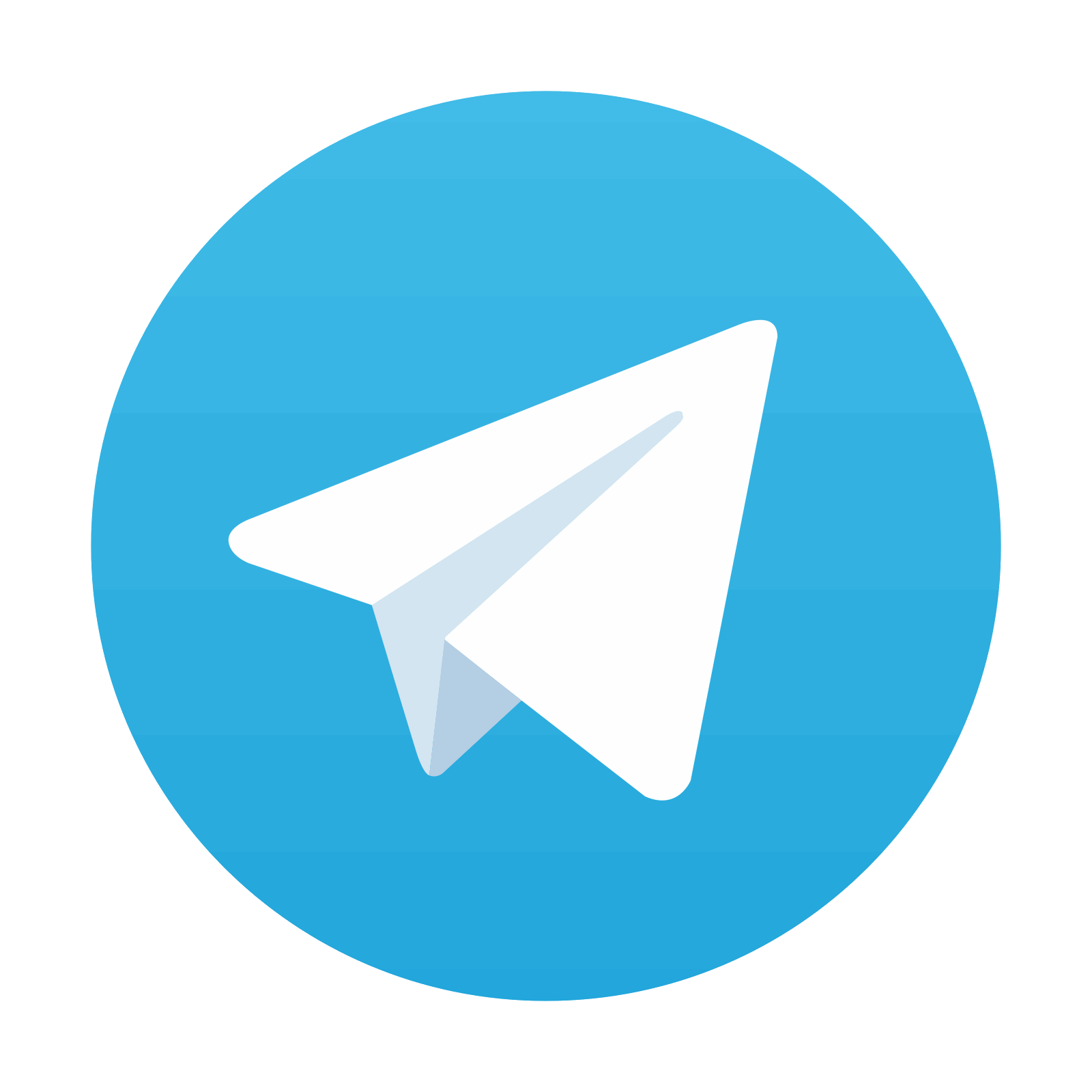
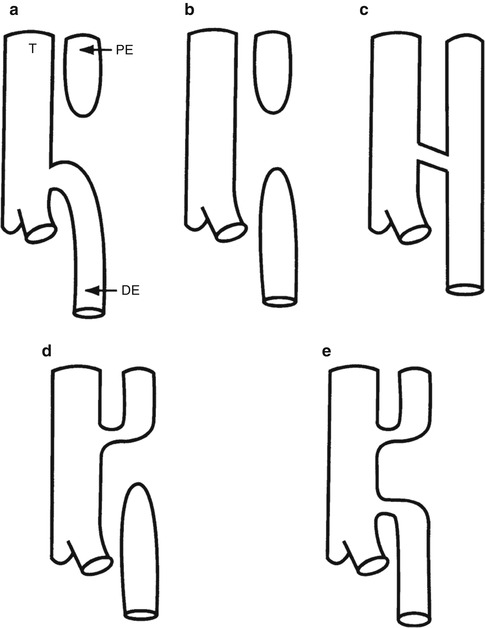
Fig. 1.13
Types of esophageal atresia and tracheoesophageal fistula. T trachea, PE proximal esophageal pouch, DE distal esophageal segment. (a) 85 % of cases. Blind proximal esophageal pouch, fistula from distal trachea to distal esophageal segment. (b) 8 % of cases. Esophageal atresia without a fistula, “pure esophageal atresia.” (c) 4 % of cases. Tracheoesophageal fistula without esophageal atresia, “H type” or “N type” (Skandalakis et al. 1994) fistula. (d) 1 % of cases. Fistula from upper trachea to proximal esophageal pouch, blind distal esophageal segment. (e) 1 % of cases. Fistula from upper trachea to proximal esophageal pouch and fistula from distal trachea to distal esophageal segment (Data from Stocker (2001))
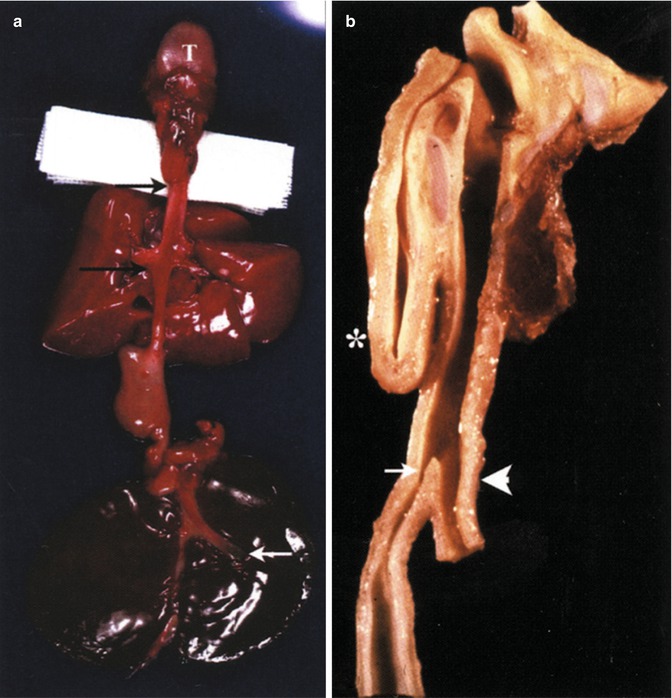
Fig. 1.14
The most common type of esophageal atresia and tracheoesophageal fistula. (See (a) in Fig. 1.13 above.) (a) This is a variation with a blind proximal esophageal pouch (upper black arrow) and a wide mouthed fistula from the carina to the distal esophageal segment (lower black arrow). T tongue. The white arrow points to the hypoplastic gallbladder. The proximal part of the distal esophageal segment demonstrates agenesis of the muscularis propria. Tracheomalacia is present. Same patient as Figs. 1.16, 1.17, and 1.18. (b) Median section of another example. The blind proximal pouch is marked with an asterisk. The brown structure on the right opposite the proximal pouch is the thyroid. The fistula from the distal trachea has a narrow mouth (white arrow) and a normal muscularis propria. This is a short gap lesion. The white arrowhead points to the distal trachea
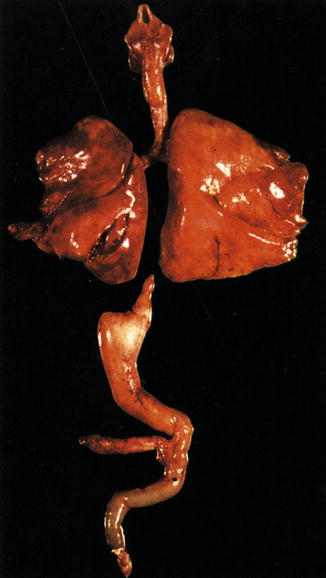
Fig. 1.15
Esophageal atresia without a fistula. The distal esophageal segment is extremely short. This is a long gap lesion. The stomach is small
< div class='tao-gold-member'>
Only gold members can continue reading. Log In or Register a > to continue
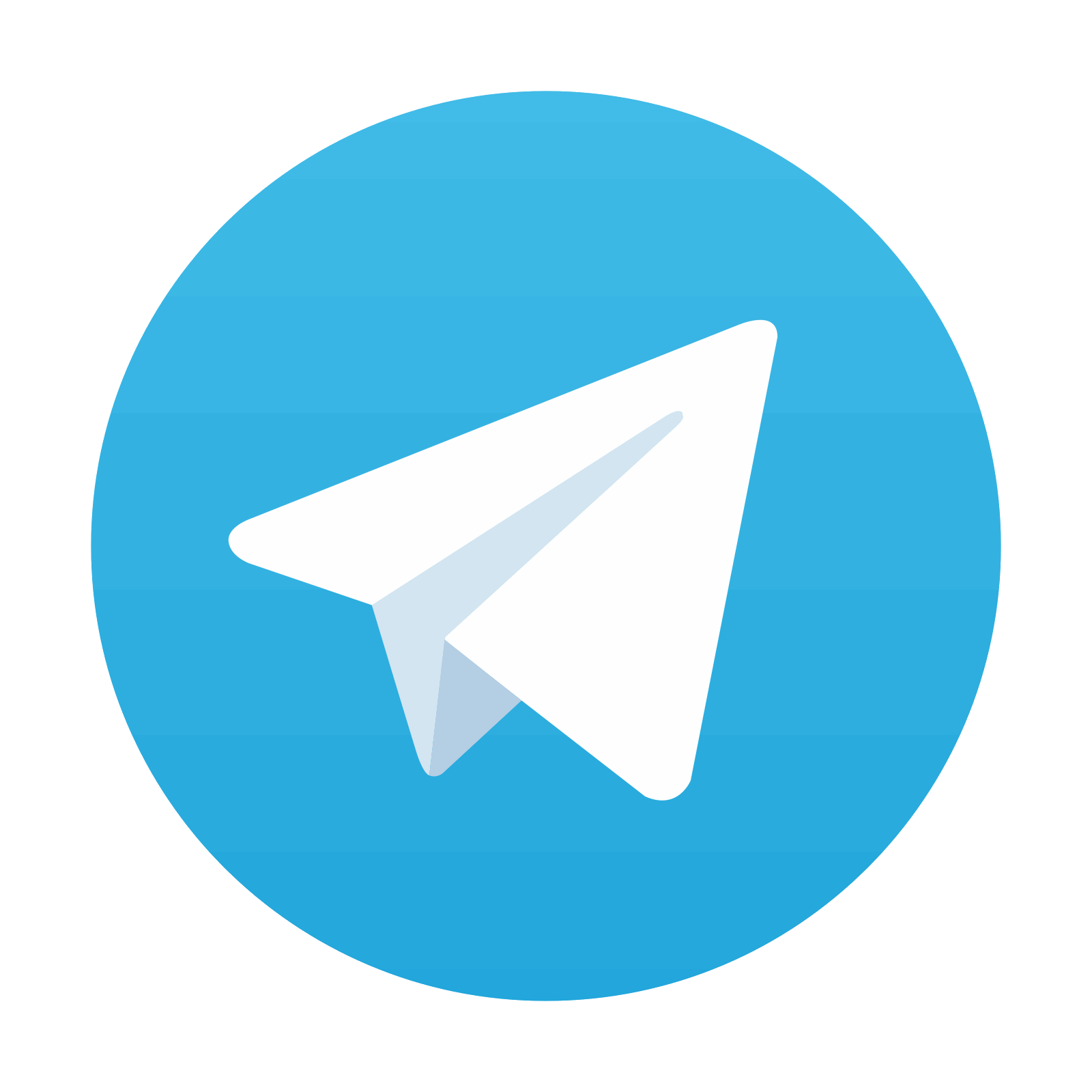
Stay updated, free articles. Join our Telegram channel

Full access? Get Clinical Tree
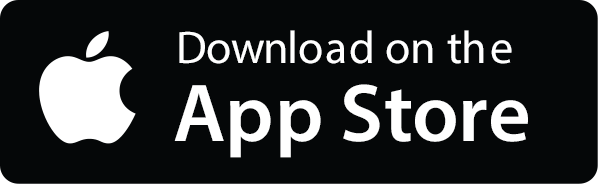
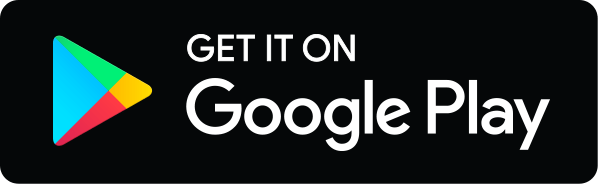
